Abstract
It is not known whether, in obesity, the capillary density or the number of circulating endothelial progenitor cells (EPCs) are reduced, or whether fibrosis of small vessels is also present. In addition, possible effects of weight reduction on these parameters have never been evaluated. Therefore, we investigated EPCs and capillary density in 25 patients with severe obesity, all submitted to bariatric surgery, and in 18 normotensive lean subjects and 12 hypertensive lean patients as controls. All patients underwent a biopsy of subcutaneous fat during bariatric surgery. In five patients, a second biopsy was obtained after consistent weight loss, about 1 year later, during a surgical intervention for abdominoplasty. EPCs and capillary density were reduced in obesity, and EPCs were significantly increased after weight reduction. Vascular collagen content was clearly increased in obese patients. No significant difference in vascular collagen was observed between normotensive obese patients and hypertensive obese patients. After pronounced weight reduction, collagen content was nearly normalized. No difference in stress–strain relation was observed among groups or before and after weight loss. In conclusion, our data suggest that microvascular rarefaction occurs in obesity. EPCs were significantly reduced in obese patients. Pronounced weight loss induced by bariatric surgery seems to induce a significant improvement of EPC number, but not of capillary rarefaction. A pronounced fibrosis of subcutaneous small resistance arteries is present in obese patients, regardless of the presence of increased blood pressure values. Consistent weight loss induced by bariatric surgery may induce an almost complete regression of microvascular fibrosis.
Introduction
Changes in the microcirculation, which include small resistance arteries, arterioles and capillaries, were well demonstrated in essential and secondary hypertension (Citation1,Citation2) as well as in type 2 diabetes mellitus (Citation3,Citation4) and in obesity (Citation5,Citation6). These changes include mainly arterial wall thickening and reduction of the internal diameter of small resistance arteries (internal lumen 100–300 μm) with a consequent increase in media to lumen ratio, that may be ascribed to eutrophic remodeling in essential hypertension (Citation1,Citation2,Citation7), and to hypertrophic remodeling in secondary hypertension (Citation7,Citation8), diabetes mellitus (Citation3) and obesity (Citation5,Citation6). Alterations in the capillary structure and distribution (microvascular rarefaction) may also be observed (Citation9).
An increased collagen deposition is a common finding in small resistance arteries of hypertensive patients (Citation10). It may be a direct consequence of the increased hemodynamic load, or of the effect of growth-promoting factors such as endothelin-1, angiotensin II and catecholamines (Citation10), and may be accounted for changes in compliance and stiffness.
Both in essential and secondary hypertension (Citation8) and in obesity (Citation5,Citation6), a pronounced endothelial dysfunction may be observed in subcutaneous small arteries, when evaluated as acetylcholine-induced vasodilation. Essential hypertension has been extensively reported to cause endothelial dysfunction, characterized by unbalanced vasodilation and vasoconstriction, increased oxidative stress, vascular inflammation, alteration of prothrombotic and fibrinolytic pathways, abnormal smooth muscle cell proliferation, and impaired repair mechanisms (Citation11,Citation12). All these factors contribute to vascular damage, to the development and progression of atherosclerosis and to the onset of cardiovascular events (Citation13–15). Therefore, integrity and functional activity of the endothelial monolayer play a crucial role in the prevention of hypertension and atherosclerosis. Recently, it has been demonstrated that the number of circulating endothelial progenitor cells (EPCs) reflects the endogenous ability to repair endothelial and vascular damages. The EPC pool declines in the presence of cardiovascular risk factors (Citation16,Citation17). EPCs are recruited from the bone marrow to areas of endothelial injury, where they differentiate and promote revascularization, restoring the integrity of the monolayer of the endothelium (Citation17). Numerical and functional impairment of EPCs are inversely related to cardiovascular risk factors and increased atherosclerotic disease risk (Citation18), and they have been linked to endothelial dysfunction (Citation19,Citation20), and to increased cardiovascular morbidity and mortality (Citation21,Citation22).
However, no data are presently available about changes in collagen content, microvascular density or number of circulating EPCs in small resistance arteries of obese patients, before or after consistent weight loss.
Patients and methods
We have investigated EPCs and capillary density in 25 patients with abdominal severe obesity, according to current definitions (Citation23), admitted to the Surgical Department of our hospital having indication to bariatric surgery (jejuno-ileal bypass or biliopancreatic derivation). Indication to bariatric surgery was made according to current clinical practice and ethics guidelines.
Ten of them were normotensive and 15 hypertensive, according to the European Society of Hypertension and of the European Society of Cardiology guidelines 2007 (Citation24). As control groups, we investigated also 18 lean normotensive subjects and 12 lean patients with essential hypertension, undergoing standard elective surgery. No patient or subject was diabetic and duration of hypertension was similar in obese and lean patients. Hypertensive patients were treated with different classes of drug including beta-blockers (4% of patients), diuretics (17% of patients), calcium channel antagonists (9% of patients), angiotensin-converting enzyme (ACE) inhibitors (17% of patients), angiotensin II antagonists (17% of patients). All subjects and patients underwent a biopsy of subcutaneous fat during surgery. In five obese patients, a second biopsy was obtained after consistent weight loss (body mass index, BMI, from 43.6 ± 5.15 to 27.7 ± 5.12, p < 0.01), about 1 year later, during a surgical intervention for abdominoplasty. Subcutaneous small resistance arteries were dissected and mounted on a pressure myograph, and mechanical properties were assessed; in particular, stress–strain relation was calculated. Populations investigated in the present study were subgroups of those enrolled in a previous study (Citation6). In a subgroup of 10 obese patients (five normotensive and five hypertensive), we investigated collagen content within the tunica media (confocal microscopy) and mechanical properties of subcutaneous small arteries. Data obtained for collagen content were compared with those observed in five lean normotensive controls.
Capillaroscopy
Skin capillary density was assessed by capillaroscopy before and after venous congestion, as described elsewhere (Citation25,Citation26). Briefly, after a period of rest in a sitting position in a quiet and temperature controlled room (21–22°C), capillaries from nailfold and the dorsum of the fourth finger of the non-dominant hand were visualized by using an epi-illuminated microscope containing a 100-W mercury vapor lamp light source, and pictures (final magnification of 200×) were obtained by video-microscopy (Videocap 3.0 D1 200, DS Medica, Milano, Italy) at baseline conditions (baseline capillary density, BCD) and after venous congestion (total capillary density, TCD), in order to visualize of functionally excluded capillaries (functional capillary density, FCD). Venous congestion was induced by inflating a miniature blood pressure cuff at 60 mmHg for 2 min, applied to the base of the fourth finger of the non-dominant hand (Citation27). Images were also obtained before and after venous congestion at the distal third forearm on the sagittal line by using a traditional pressure cuff. Capillary density was defined as the number of capillaries per square millimeter of the microscopic field and was counted by hand. Capillary density was determined by two independent operators and findings were averaged.
EPCs isolation and count
The number of EPCs was assessed using an in vitro assay as previously described (Citation28,Citation29). Mononuclear cells were isolated from peripheral venous blood by using density gradient centrifugation with Ficoll (Amersham) and seeded 107 cells on six-well plates coated with human fibronectin (Sigma) in endothelial basal medium (EBM-2; Clonetics) supplemented with growth factors (VEGF, FGF-2, EGF, IGF-1), ascorbic acid, hydrocortisone, gentamicin and 10% fetal bovine serum. After 7 days in culture, we assessed fluorescent chemical detection of the attached cells using 1,1′-dioctadecyl-3,3,3′,3′-tetramethylindocarbocyanine-labeled acetylated low-density lipoprotein (acLDL- DiI, 2.4 mg/ml; Molecular Probes) and fluorescein isothiocyanate-labeled Ulex europaeus agglutinin-1 (10 mg/ml UEA-1; Sigma) for cell staining. Samples were counted with an inverted fluorescent microscope (cell number/107 plated cells per 1.17 mm2 of area). Positive double staining for both UEA-1 and acLDL-DiI were identified as EPCs. DAPI were used to stain the nuclei.
Vascular mechanics
Small resistance arteries were dissected from surgical biopsies and mounted on a pressure myograph and perfused with Ca2+-free PSS containing 10 mmol/l EGTA for 30 min to eliminate myogenic tone. In order to evaluate vascular mechanics, intraluminal pressure was increased stepwise 10 to 140 mmHg. Internal diameter and media thickness were measured at each step (Citation30,Citation31).
Mechanical parameters were calculated as previously reported (Citation32,Citation33). Media cross-sectional area was calculated as (π/4)×(De2 − Di2), where De and Di are external and internal diameters, respectively. Circumferential strain (ϵ) is obtained as (D−Do)/Do, where D is the internal diameter for a given intraluminal pressure, and Do is the original diameter at 3 mmHg (baseline diameter). Circumferential stress (σ) is (PD)/(2M), where P is the intraluminal pressure (dyne/cm2), and D and M are lumen diameter and media thickness, respectively. Elastic modulus was assessed by fitting stress–strain data to σ = σoeβϵ, where σo was stress at Do and β is a constant related to the rate of increase of the stress–strain curve.
Collagen evaluation by confocal microscopy
Subcutaneous small resistance arteries were fixed for 30 min at 60 mmHg of intraluminal pressure with a solution containing 3.5% formaldehyde and 0.75% glutaraldehyde in 50 mmol/l phosphate-buffered saline (PBS), pH 7.4 (Citation31,Citation34). Vessels were then incubated with 0.5% BSA/PBS (5 min at 42°C), treated with hyaluronidase (1 mg/ml), and incubated with antibody to collagen type I/III (1:20, overnight at 4°C) (Calbiochem). A secondary antibody (Alexa Fluor 647 anti-rabbit IgG, Molecular Probes) was applied for 30 min at 37°C. For the final 30 min of incubation, rhodamine/phalloidin (Sigma, 10 μmol/l, Molecular Probes) was added to stain α-actin. Arteries were mounted in 1:1 glycerol/PBS (pH 7.4) on glass coverslips and studied by confocal immunofluorescence microscopy with a Zeiss LSM 510 system, and a stack of sliced images was obtained. The amount of collagen I/III present in the vessels were quantified by imaging (Zeiss LSM Examiner Software), and expressed as a percentage of collagen/total surface area (% pixel/surface area).
Statistical analysis
All data are expressed as mean± SD unless otherwise stated. One-way and two-way analysis of variance (ANOVA) as well as and χ-square test when appropriate were used to evaluate differences among groups or between groups. Where a one-way ANOVA was performed, a Tukey and a Dunnet's post hoc test were performed. In case of a two-way ANOVA, significance within steps or between groups and interactions between steps and groups (within and between factor) were calculated. Conventional statistical significance was set at 0.05. All variables were normally distributed. All analyses were carried out with the BMDP statistical package (BMDP software programs 7D, 8D, 1V, BMDP Statistical Software Inc., Los Angeles, CA, USA).
Results
Demographic data are summarized in . Differences in blood pressure and BMI were those expected on the basis of the definitions of groups. There were no significant differences in gender distribution among the groups. Additional information about demographic characteristics of control subjects and obese patients, before or after weight loss, were reported previously (Citation6).
Table I. Demographic characteristics, circulating endothelial progenitor cells (EPCs) and capillary density in the different groups.
Capillary density
Both BCD and TCD in the dorsum of the fourth finger were significantly reduced in normotensive and hypertensive obese patients considered together (71.85 ± 17.83 and 82.00 ± 18.49, respectively), compared with all lean patients (98.78 ± 19.4 and 108.7 ± 22.3, respectively) (p < 0.001 for both BCD and TCD). Similar data were obtained in the forearm (57.6 ± 9.69 and 66.5 ± 7.23 vs 84.3 ± 17.6 and 99.7 ± 21.3, respectively, p < 0.001 in both cases).
Data obtained in the different groups were reported in . BCD and TCD were reduced in both groups of obese patients compared with the remaining groups. No difference in BCD and TCD was found between obese hypertensive and obese normotensive patients in the dorsum. Similar results were obtained for BCD and TCD in the forearm between obese hypertensive group and obese normotensives. FCD did not differ between obese normotensive and hypertensive patients. We did not find any significant differences among lean hypertensive and lean normotensive groups ().
Representative images of BCD and TCD in normotensive and hypertensive lean subject, as well as in normotensive and hypertensive obese patients before and after weight reduction, are reported in .
Figure 1. Representative images of baseline (Citation1) and total (after venous congestion) (Citation2) capillary density (BCD and TCD, respectively) in the dorsum of the fourth finger of the non-dominant hand in normotensive lean subject (panel A), in hypertensive lean patient (panel B), and normotensive and hypertensive obese patients before weight reduction (panel C and D, respectively).
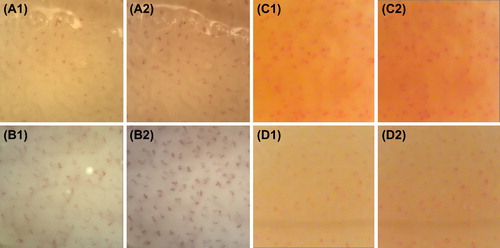
After weight loss, BCD and TCD in the dorsum (69.67 ± 17.16 and 80.00 ± 9.17, respectively) and in the forearm (73.50 ± 4.95 and 79.00 ± 5.66, respectively) were not significant different from BCD and TCD at baseline in the dorsum (72.00 ± 23.30 and 82.67 ± 12.66, respectively) and in the forearm (66.00 ± 4.88 and 79.00 ± 5.12, respectively). No significant differences were observed in the nailfold among the groups (data not shown).
EPC count
EPCs were significantly reduced in normotensive and hypertensive obese patients considered together (29.8 ± 46.7), compared with all lean patients (63.9 ± 45.7, p < 0.01).
Results for EPCs count in the different groups are reported in . At baseline, EPC count was significantly reduced in normotensive and hypertensive obese patients compared with normotensive and hypertensive lean patients. Hypertensive obese patients showed an even more evident impairment in EPC number compared with normotensive obese patients as well as compared with hypertensive lean patients.
A significantly increase in EPC number was observed after weight reduction (from 18.7 ± 14.3 to 116 ± 84.4, p < 0.05).
Vascular mechanics
Increasing intraluminal pressure to 140 mmHg decreased the media/lumen ratio of relaxed subcutaneous small resistance arteries from vessels from obese patients before and after weight loss to similar degrees without altering media cross-sectional area. Vessels from obese patients showed a modest, not significant, reduction in passive distensibility before and after weight loss, as expressed by strain curve (data not shown). In addition, in obese patients, media stress and increasing intraluminal pressure was slightly greater after weight reduction compared with baseline, although the difference was of borderline statistical significance (). The stress–strain curve was shifted rightward in small resistance arteries from obese patients after weight loss compared with baseline (), albeit also this difference did not reach the statistical significance. Indeed, when plotted against intraluminal pressure or stress, no differences were observed in incremental elastic modulus in obese patients before and after weight loss (data not shown).
Figure 2. Mechanic properties of subcutaneous small resistance arteries. Media stress (A) at different intraluminal pressures in relaxed subcutaneous small resistance arteries from normotensive and hypertensive obese patients (considered together) before and after weight loss. (B) Media stress–strain relationship in relaxed subcutaneous small resistance arteries from normotensive and hypertensive obese patients (considered together) before and after weight loss. Results are expressed as mean ± SEM. Full circle: obese patients at baseline; empty circle: obese patients after weight reduction.
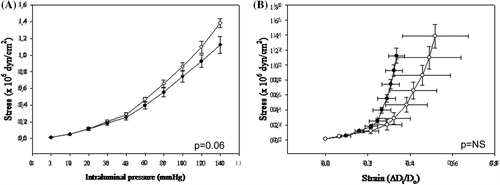
Collagen content in subcutaneous small resistance arteries
Vascular collagen content, expressed as a percent of total surface area, was significantly increased in obese patients, compared with normal controls (, ). No significant difference in vascular collagen content was observed between normotensive obese patients and hypertensive obese patients (). After pronounced weight reduction (BMI from 38.1 ± 1.88 to 25.7 ± 3.72, p < 0.05), collagen content was nearly normalized (from 17.6 ± 10.1 to 3.44 ± 3.84 arbitrary units, p < 0.05).
Table II. Demographic characteristics and vascular collagen content in the subgroup of obese patients an normotensive lean controls.
Figure 3. Representative Laser confocal microscopic images for collagen type I/III deposition in subcutaneous small resistance arteries from normotensive lean subject (A), normotensive and hypertensive obese patients (B and C, respectively), and normotensive and hypertensive obese patient after weight reduction (D and E, respectively). Vessels were labeled with rhodamin–phalloidin for smooth-muscle actin (red fluorescence) and with anticollagen type I/III (green fluorescence) and visualized by confocal microscopy.
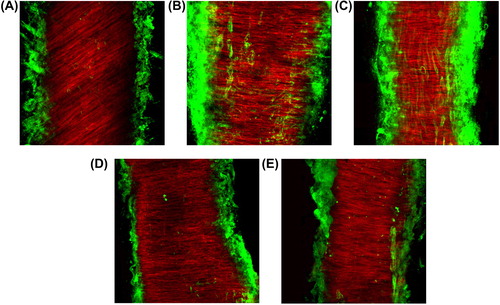
Discussion
In the present study, a significant increase in collagen deposition within the tunica media of subcutaneous small resistance arteries was observed. Vascular collagen deposition, which characterizes obesity, occurs independently of the presence of hypertension, and may be totally corrected by consistent weight loss obtained by bariatric surgery. However, mechanical properties of the vessels (stress–strain relation) were similar in obese patients and controls, and were almost unaffected by weight loss. Also Grassi and colleagues were unable to observe changes in the stiffness of subcutaneous small arteries of obese patients, compared with controls (Citation5,Citation35). An increased collagen and fibronectin deposition and collagen/elastin ratio were previously observed in the small arteries of non-diabetic and diabetic hypertensive individuals (Citation3,Citation36,Citation37), possibly triggered by elevated blood pressure values. However, an altered vascular structure and an increased collagen deposition do not seem to translate into a change of mechanical properties in essential or secondary hypertensive patients, since other factors (connections between vascular smooth muscle cells and the extracellular matrix proteins, etc.) may be involved (Citation38–40). In one case, an increased collagen content in small arteries was even associated with an increased elastic modulus with a paradoxical reduction in stiffness (Citation36) although this aspect, at least in humans, remains controversial (Citation41). As previously mentioned, in severe obesity wall stiffness in the microcirculation is not increased (Citation5), at variance from what has been reported in the large and medium-size arteries (Citation42–44). Similarly, in patients with metabolic syndrome the wall stiffness of the small resistance arteries is not increased, as demonstrated by Grassi et al. (Citation35) again, in contrast to what has been reported for large and medium-sized arteries (Citation42,Citation45–47). Therefore, it is not surprising that in our study no change in stress/strain relation after weight reduction in obese patients was observed, despite of an increase in vascular collagen content.
Structural changes in the microcirculation have been observed in hypertension at different vascular levels, such as both omental and subcutaneous tissues (Citation1–3,Citation5,Citation6). In our study, BCD and TCD resulted significantly reduced in normotensive and hypertensive obese patients compared with lean subjects. Decrease in BCD could be explained by an increased vascular tone in the obese population. However, it was previously demonstrated that microvascular rarefaction might be correlated with media thickness to lumen diameter ratio of small arteries (Citation48), therefore, the same mechanisms possible involved in the development of hypertrophic remodeling of small resistance artery in obesity (Citation5,Citation6,Citation35) could explain the capillary rarefaction observed in the present study.
In addition to structural changes, endothelial dysfunction is an important component of the metabolic syndrome (Citation35), and may be the consequence of an unbalance between vasoconstrictor and vasodilator factors, with consequent impairment of endothelium-dependent vasodilation, of an increased oxidative stress, and of an elevated expression of pro-inflammatory and prothrombotic factors. Endothelial dysfunction contributes to development of microvascular complications and it has been demonstrated to be present in essential hypertension (Citation8), type 2 diabetes (Citation3) and obesity (Citation5,Citation6,Citation35,Citation49).
Interestingly enough, increased levels of blood pressure per se, probably through an increased wall stress, may stimulate an inflammatory reaction and increase reactive oxygen species, which in turn may herald the changes in the vascular wall and functional characteristic of hypertension (Citation50). Similarly, visceral obesity may induce insulin resistance, reactive oxygen species production and low-grade chronic inflammation characterized by the production of abnormal adipokines and cytokines such as tumoral necrosis factor-alpha, interleukin 1 and 6, free fatty acids, leptin and resistin as well as an altered coagulation/fibrinolysis balance (Citation51–53).
In the present study, the reduction of TCD in obese patients was accompanied by a reduction of EPC count, thus suggesting a possible impairment of repair of vascular damage as well as neovascularization in obese patients. According with this, the presence of endothelial dysfunction in the microcirculation of obese patients, evaluated as vasodilation response to acetylcholine, has been already observed by our and other groups (Citation5,Citation6,Citation35). Inflammation and oxidative stress might be obvious candidates in explaining the observed reduction in EPCs (Citation6). An interesting finding is represented by the observation of an increase in circulating EPCs after consistent weight reduction. Also in this case, a reduction of oxidative stress and inflammation, observed in these patients after surgical correction of obesity, such us an improvement of both glycemic and lipid profile (Citation6), might contribute to explain the observed finding. Indeed, all these factors could result in a reduction of EPC apoptosis and senescence thus indicating an improvement in ability of vascular repair. Otherwise, the lack of change in capillary density after weight reduction could be ascribed to short observation time of our patient population since a longer period may be necessary to correct microvascular rarefaction. Accordingly, an improvement, but not a completely regression, of subcutaneous small artery vascular remodeling was observed in obese patients after weight loss (Citation6).
In conclusion, our data suggest that microvascular rarefaction occurs in obesity. EPCs were significantly reduced in obese patients, while collagen content in subcutaneous small arteries is increased, regardless of the presence of increased blood pressure values. Pronounced weight loss induced by bariatric surgery seems to induce a significant improvement of EPC number and collagen content, but not of capillary rarefaction or mechanical properties of small vessels.
Declaration of interest: The authors report no conflicts of interest. The authors alone are responsible for the content and writing of the paper.
References
- Mulvany MJ, Aalkjaer C. Structure and function of small arteries. Physiol Rev. 1990;70:921–971.
- Schiffrin EL. Remodeling of resistance arteries in essential hypertension and effects of antihypertensive treatment. Am J Hypertens. 2004;7:1192–1200.
- Rizzoni D, Porteri E, Guelfi D, Muiesan ML, Valentini U, Cimino A, et al. Structural alterations in subcutaneous small arteries of normotensive and hypertensive patients with non insulin dependent diabetes mellitus. Circulation. 2001;103: 1238–1244.
- Greenstein AS, Price A, Sonoyama K, Paisley A, Khavandi K, Withers S, et al. Eutrophic remodeling of small arteries in type 1 diabetes mellitus is enabled by metabolic control: A 10-year follow-up study. Hypertension. 2009;54:134–141.
- Grassi G, Seravalle G, Scopelliti F, Dell’Oro R, Fattori L, Quarti-Trevano F, et al. Structural and functional alterations of subcutaneous small resistance arteries in severe human obesity. Obesity (Silver Spring). 2010;18:92–98.
- De Ciuceis C, Porteri E, Rizzoni D, Corbellini C, La Boria E, Boari GEM, et al. Effects of weight loss on structural and functional alterations of subcutaneous small arteries in obese patients. Hypertension. 2011;58:29–36.
- Heagerty AM, Aalkjaaer C, Bund SJ, Korsgaard N, Mulvany MJ. Small artery structure in hypertension. Dual process of remodeling and growth. Hypertension. 1993; 21:391–397.
- Rizzoni D, Porteri E, Castellano M, Bettoni G, Muiesan ML, Muiesan P, et al. Vascular hypertrophy and remodeling in secondary hypertension. Hypertension. 1996;28:785–790.
- Levy BI, Schiffrin EL, Mourad JJ, Agostini D, Vicaut E, Safar ME, et al. Impaired tissue perfusion: A pathology common to hypertension, obesity, and diabetes mellitus. Circulation. 2008;118:968–976.
- Intengan HD, Schiffrin EL. Vascular remodeling in hypertension: Roles of apoptosis, inflammation, and fibrosis. Hypertension. 2001;38:581–587.
- Ross R. The pathogenesis of atherosclerosis – An update. N Engl J Med. 1986;314:488–500.
- Deanfield JE, Halcox JP, Rabelink TJ. Endothelial function and dysfunction: Testing and clinical relevance. Circulation. 2007;115:1285–1295.
- Lerman A, Zeiher AM. Endothelial function: Cardiac events. Circulation. 2005;1111:363–368.
- Drexler H. Endothelial dysfunction: Clinical implications. Prog Cardiovasc Dis. 1997;39:287–324.
- Widlansky MR, Gokce N, Keaney JF, Vita JA. The clinical implications of endothelial dysfunction. J Am Coll Cardiol. 2003;42:1149–1160.
- Asahara T, Murohara T, Sullivan A, Silver M, van der Zee R, Li T, et al. Isolation of putative progenitor endothelial cells for angiogenesis. Science. 1997;275:964 –967.
- Asahara T, Masuda H, Takahashi T, Kalka C, Pastore C, Silver M, et al. Bone marrow origin of endothelial progenitor cells responsible for postnatal vasculogenesis in physiological and pathological neovascularization. Circ Res. 1999;85: 221–228.
- Vasa M, Fichtlscherer S, Aicher A, Adler K, Urbich C, Martin H, et al. Number and migratory activity of circulating endothelial progenitor cells inversely correlate with risk factors for coronary artery disease. Circ Res. 2001;89:E1–E7.
- Hill JM, Zalos G, Halcox JP, Schenke WH, Waclawiw MA, Quyyumi AA, et al. Circulating endothelial progenitor cells, vascular function, and cardiovascular risk. N Engl J Med. 2003;348:593–600.
- Quyyumi AA, Hill JM. Circulating endothelial progenitor cells as novel biological determinants of vascular function and risk. Can J Cardiol. 2004;20 Suppl B:44B–48B.
- Schmidt-Lucke C, Rossig L, Fichtlscherer S, Vasa M, Britten M, Kamper U, et al. Reduced number of circulating endothelial progenitor cells predicts future cardiovascular events: Proof of concept for the clinical importance of endogenous vascular repair. Circulation. 2005;111:2981–2987.
- Werner N, Kosiol S, Schiegl T, Ahlers P, Walenta K, Link A, et al. Circulating endothelial progenitor cells and cardiovascular outcomes. N Engl J Med. 2005;353:999–1007.
- Crepaldi G, Belfiore F, Bosello O, Caviezel F, Contaldo F, Enzi G, et al. Italian Consensus Conference – Overweight, obesity and health. Int J Obes. 1991;15:781–790.
- 2007 Guidelines for the management of arterial hypertension: The Task Force for the Management of Arterial Hypertension of the European Society of Hypertension (ESH) and of the European Society of Cardiology (ESC). J Hypertens. 2007; 25:1105–1187.
- Ciuffetti G, Schillaci G, Innocente S, Lombardini R, Pasqualini L, Notaristefano S, et al. Capillary rarefaction and abnormal cardiovascular reactivity in hypertension. J Hypertens. 2003;21:2297–2303.
- Antonios TFT, Kaski JC, Hasan KM, Brown SJ, Singer DRJ. Rarefaction of skin capillaries in patients with anginal chest pain and normal coronary arteriograms. Eur Heart J. 2001; 22:1144–8114.
- Antonios TFT, Rattray FE, Singer DR, Markandu ND, Mortimer PS, MacGregor GA. Maximization of skin capillaries during intravital video-microscopy in essential hypertension: Comparison between venous congestion, reactive hyperaemia and core heat load tests. Clin Sci. 1999; 97:523–528.
- Dimmeler S, Aicher A, Vasa M, Mildner-Rihm C, Adler K, Tiemann M, et al. HMG-CoA reductase inhibitors (statins) increase endothelial progenitor cells via the PI 3-kinase/Akt pathway. J Clin Invest. 2001;108:391–397.
- De Ciuceis C, Pilu A, Cappelli C, Porteri E, Zani F, Santoro A, et al. Decreased number of circulating endothelial progenitor cells in patients with Graves’ hyperthyroidism. J Endocrinol Inves. 2011;34:335–339.
- Schiffrin EL, Deng LY. Structure and function of resistance arteries of hypertensive patients treated with a beta blocker or a calcium channel antagonist. J Hypertens. 1996;14: 1237–1244.
- Rizzoni D, De Ciuceis C, Porteri E, Paiardi S, Boari GE, Mortini P, et al. Altered structure of small cerebral arteries in patients with essential hypertension. J Hypertens. 2009; 27:838–845.
- Laurant P, Touyz RM, Schiffrin EL. Effect of pressurization on mechanical properties of mesenteric small resistance arteries from spontaneously hypertensive rats. J Vasc Res. 1997;34:117–125.
- Savoia C, Touyz RM, Endemann DH, Pu Q, Ko EA, De Ciuceis C, et al. Angiotensin receptor blocker added to previous antihypertensive agents on arteries of diabetic hypertensive patients. Hypertension. 2006;48:271–277.
- Savoia C, Touyz RM, Volpe M, Schiffrin EL. Angiotensin type 2 receptor in resistance arteries of type 2 diabetic hypertensive patients. Hypertension. 2007;49:341–6.
- Grassi G, Seravalle G, Brambilla G, Facchetti R, Bolla G, Mozzi E, et al. Impact of the metabolic syndrome on subcutaneous microcirculation in obese patients. J Hypertens. 2010;28:1708–1714.
- Intengan HD, Deng LY, Li JS, Schiffrin EL. Mechanics and composition of human subcutaneous resistance arteries in essential hypertension. Hypertension. 1999;33:569–574.
- Savoia C, Touyz RM, Amiri F, Schiffrin EL. Selective mineralocorticoid receptor blocker eplerenone reduces resistance artery stiffness in hypertensive patients. Hypertension. 2008; 51:432–439.
- Thybo NK, Mulvany MJ, Jastrup B, Nielsen H, Aalkjaer C. Some pharmacological and elastic characteristics of isolated subcutaneous small arteries from patients with essential hypertension. J Hypertens. 1996;14:993–998.
- Rizzoni D, Porteri E, Guefi D, Piccoli A, Castellano M, Pasini G, et al. Cellular hypertrophy in subcutaneous small arteries of patients with renovascular hypertension. Hypertension. 2000;35:931–935.
- Park JB, Intengan HD, Schiffrin EL. Reduction of resistance artery stiffness by treatment with the AT(1)-receptor antagonist losartan in essential hypertension. J Renin Angiotensin Aldosterone Syst. 2000;1:40–45.
- Mulvany MJ. A reduced elastic modulus of vascular wall components in hypertension?Hypertension. 1992;20:7–9.
- Ferreira I, Twisk JW, van Mechelen W, Kemper HC, Seidell JC, Stehouwer CD. Current and adolescent body fatness and fat distribution: Relationships with carotid intima-media thickness and large artery stiffness at the age of 36 years. J Hypertens. 2004;22:145–155.
- Ferreira I, Henry RM, Twisk JW van Mechelen W, Kemper HC, Stehouwer CD; Amsterdam Growth and Health Longitudinal Study. The metabolic syndrome, cardiopulmonary fitness, and subcutaneous trunk fat as independent determinants of arterial stiffness: The Amsterdam Growth and Health Longitudinal Study. Arch Intern Med. 2005; 165:875–882.
- Zebekakis PE, Nawrot T, Thijs L, Balkestein EJ, van der Heijden-Spek J, Van Bortel LM, et al. Obesity is associated with increased arterial stiffness from adolescence until old age. J Hypertens. 2005;23:1839–1846.
- Czernichow S, Bertrais S, Blacher J, Oppert JM, Galan P, Ducimetière P, et al. ; SU.VI.MAX. Vascular Study. Metabolic syndrome in relation to structure and function of large arteries: A predominant effect of blood pressure. A report from the SU.VI.MAX. Vascular Study. Am J Hypertens. 2005; 18:1154–1160.
- Toledo-Corral CM, Ventura EE, Hodis HN, Weigensberg MJ, Lane CJ, Li Y, et al. Persistence of the metabolic syndrome and its influence on carotid artery intima media thickness in overweight Latino children. Atherosclerosis. 2009;206: 594–598.
- Ghiadoni L, Penno G, Giannarelli C, Plantinga Y, Bernardini M, Pucci L, et al. Metabolic syndrome and vascular alterations in normotensive subjects at risk of diabetes mellitus. Hypertension. 2008;51:440–445.
- Paiardi S, Rodella LF, De Ciuceis C, Porteri E, Boari GEM, Rezzani R, et al. Immunohistochemical evaluation of microvascular rarefaction in hypertensive humans and in spontaneously hypertensive rats. Clin Hemorheol Microcirc. 2009;42: 259–268.
- Virdis A, Santini F, Colucci R, Duranti E, Salvetti G, Rugani I, et al. Vascular generation of tumor necrosis factor-α reduces nitric oxide availability in small arteries from visceral fat of obese patients. J Am Coll Cardiol. 2011;58:238–247.
- Touyz RM, Savoia C, He Y, Endemann D, Pu Q, Ko EA, et al. Increased inflammatory biomarkers in hypertensive type 2 diabetic patients: Improvement after angiotensin II type 1 receptor blockade. J Am Soc Hypertens. 2007;1: 189–199.
- Iyer A, Fairlie DP, Prins JB, Hammock BD, Brown L. Inflammatory lipid mediators in adipocyte function and obesity. Nat Rev Endocrinol. 2010;6:71–82.
- Karalis KP, Giannogonas P, Kodela E, Koutmani Y, Zoumakis M, Teli T. Mechanisms of obesity and related pathology: Linking immune responses to metabolic stress. FEBS J. 2009;276:5747–5754.
- Hopps E, Noto D, Caimi G, Averna MR. A novel component of the metabolic syndrome: The oxidative stress. Nutr Metab Cardiovasc Dis. 2010;20:72–77.