Abstract
Rationale: Kidneys are dynamic organs and represent one of the major systems maintaining the body homeostasis; they are affected by many chemicals and drugs. Grape seed extract (GSE) has been targeted to prevent drug-induced renal toxicity. Objectives: This study investigates the possible renoprotective effect of GSE against oxidative stress, renal impairment, and hypercholesterolemia (HC) induced by gentamicin (GM) and cholesterol-enriched diet. Seventy adult male Wistar rats (160 ± 10 g) were divided into seven groups: (1) served as control, (2) GSE, (3) GM, (4) GSE + GM, (5) hypercholesterolemic (HC) group, (6) GM + HC group, and (7) GM + HC + GSE. Kidney functions, inflammatory mediators, cytokines, lipid profile, nitric oxide (NO), cyclic guanosine monophosphate (cGMP), and oxidative and antioxidative stress parameters were assessed in all groups. Main findings: GM induced renal dysfunction, which was exacerbated by the presence of HC as confirmed by laboratory determinations. Administration of GSE attenuated the renal toxicity evidenced in significant reduction in elevated kidney function, inflammatory cytokines as well as lipid profile, NO, cGMP, enzymatic, and nonenzymatic antioxidants. Conclusion: Administration of GSE simultaneously with GM attenuated oxidative stress, diminished renal toxicity, and improved lipid profile induced by GM and HC.
INTRODUCTION
A number of environmental contaminants, chemicals, and drugs including antibiotics and high cholesterol diet dramatically alter the structure and function of kidney tissues and produce multiple deleterious effects.Citation1,2
Aminoglycoside antibiotics, particularly gentamicin (GM), are frequently used in the treatment of severe gram-negative infection.Citation3 However, their clinical exploitation is limited due to the chance of nephrotoxicity.Citation4 GM nephrotoxicity has a complex phenomenon characterized by an increase in plasma creatinine and urea levels as well as severe proximal renal tubular necrosis, followed by deterioration and renal failure.Citation1 It is apparent that the toxicity of GM involves renal free radical generation, reduction in antioxidant defense mechanism, acute tubular necrosis and glomerular congestion,Citation3 renal inflammatory cascades, high renal oxidative stress, and associating pathological signaling mechanisms.Citation5
Hypercholesterolemia (HC) plays an important role in the development and progression of atherosclerotic process, accelerates the development of fibrosis in the stenotic kidney, and increases the propensity for end stage of renal disease.Citation6 Cholesterol-rich diet significantly elevated urinary excretion of urea, creatinine, lipid peroxidation (LPO) products in the kidney and erythrocytes, while significantly attenuated reduced glutathione (GSH) and antioxidative enzymes activities.Citation7 It exacerbated the development of nephropathy with elevations of renal hypertrophy and mesangial matrix,Citation8 affected the level of hemoglobin and its derivatives which cause anemia, and may produce reactive oxygen species (ROS) and other free radicals increasing thiobarbituric acid reactive substances (TBARS) and decreasing superoxide dismutase (SOD) and glutathione peroxidase (GSH-Px) enzyme activities.Citation2 HC aggravates contrast media-induced nephrotoxicity through the reduced production of nitric oxide (NO).Citation9 The concurrent feeding of cholesterol-enriched diet with the GM treatment resulted in significantly greater biochemical and morphological changes in renal tissue than those following GM alone.Citation10
Grape seed extract (GSE) contains important vitamins, minerals, and polyphenols including flavonoids and tannins.Citation11,12 Tannins are secondary plant metabolites subdivided into condensed and hydrolyzable compounds. Condensed tannins are known as proanthocyanidins, which are the most abundant flavonoids present in GSE.Citation13 GSE demonstrated the strongest antioxidant effects and free radical scavenging abilityCitation14 as well as being anti-inflammatory,Citation15 anticancer,Citation16 antibacterialCitation17; it has platelet aggregation inhibiting and metal chelating propertiesCitation18 and vasodilatory, immunostimulating, antiallergic, antiviral activatesCitation19; it reduced plasma cholesterol in animals fed a high cholesterol diet and that may reduce risk of atherosclerosis.Citation20 Recently, GSE has been marketed as dietary supplement and advocated for its multiple beneficial properties.Citation14
Based on the reported antioxidant and anti-inflammatory properties and vasodilatory action of polyphenolic compounds that exist in GSE, the hypothesis was made that GSE could attenuate oxidative stress, renal damage, and atherosclerosis induced by GM and HC. Therefore, the aim of this study was to investigate the renoprotective effect of GSE against oxidative stress, renal damage, and atherosclerosis induced by GM and HC in rats through the assessment of kidney biochemical parameters and lipid profile.
MATERIAL AND METHODS
Seventy adult male Wistar rats of similar age and weight (160 ± 10 g) were obtained from the Egyptian Organization of Biological Products and Vaccines (Helwan, Egypt). Animals were housed in stainless steel wire mesh suspended rodent cages under standard laboratory conditions of humidity 62%, temperature 25 ± 2°C, and 12 h light/dark cycle. Rodent chow diet and water were allowed ad libitum. All animals received human care in compliance with guidelines of the Ethical Committee of Medical Research of National Research Centre, Egypt.
Chemicals
GSE is a dried powder in capsules (150 mg) commercially known as Gervital® and was obtained from Mepco Pharma (Cardiff, UK) produced by Arab Company for Pharmaceuticals and Medicinal Plants, Cairo, Egypt. GM was purchased from Sigma Chemicals (St. Louis, MO, USA). All other chemicals and reagents used in the experiment were of analytical grade.
Experimental Design
Two weeks after acclimatization, rats were divided into 7 groups of 10 animals each. The first group was kept on saline and served as control. The second group was orally (16 G ball-tipped feeding needle with syringe) administered 150 mg/kg/day GSE for 30 daysCitation21 and served as GSE group. The third group was injected subcutaneously with 50 mg/kg GM twice daily for 15 daysCitation1,22 and served as GM group. The fourth group was injected subcutaneously with 50 mg/kg GM twice daily for 15 days and orally administered GSE (150 mg/kg day) concomitantly with GM and 15 days after and served as GSE + GM group. The fifth group was kept on cholesterol-rich diet (4% cholesterol, 1% cholic acid, and 0.5% thiouracil) first for 6 weeks and continued throughout the drugs administration for another 30 daysCitation23 and served as hypercholesterolemic (HC) group. The sixth group was kept on cholesterol-rich diet and injected subcutaneously with 50 mg/kg GM twice daily for 15 days and served as HC + GM group. The seventh group was kept on cholesterol-rich diet and injected with 50 mg/kg GM twice daily for 15 days and was orally administered 150 mg/kg/day GSE concomitantly with GM and 15 days after and served as HC + GSE + GM group.
Blood Sampling
At the end of treatment period (30 days), animals were fasted overnight and anesthetized using diethyl ether. Blood samples were collected from retro-orbital venous plexus using a glass capillary collector. One portion of each blood sampling was collected in dry centrifuge tubes for serum separation, centrifuged at 3000 × g for 15 min (4°C), then stored at −20°C for further determination of creatinine, urea, uric acid, N-acetyl-β-glucosaminidase (NAG), interleukin 1β (IL-1β), tumor necrosis factor α (TNF-α), monocyte chemoattractant protein 1 (MCP-1), total cholesterol (TC), triglyceride (TG), high-density lipoprotein (HDL), and low-density lipoprotein (LDL). The other portion of each blood sample was collected in heparinized tubes for plasma separation, centrifuged at 3000 × g for 20 min (4°C) and stored at −20°C for subsequent determinations of NO and cyclic guanosine monophosphate (cGMP).
Tissue Sampling
After blood collection, all animals were rapidly killed and the right kidneys were removed and washed with 0.9% NaCl and quickly frozen in liquid nitrogen (–170°C ), then stored at −20°C for further determinations of LPO, SOD, GSH, GSH-Px, and catalase (CAT).
Biochemical Analysis
Serum creatinine concentration was measured kinetically as described by HenryCitation24 using Diamond Diagnostic Kits (Cairo, Egypt) following the manufacturer and expressed as mg/dL. Serum urea was quantitatively determined using the QCA kit (urea Quimica Clinica Aplicada, S.A. Tarragona, Spain) with the modified Searcy methodCitation25 and expressed as mg/dL. Uric acid concentration was determined colorimetrically as described by Haisman and Muller,Citation26 using Stanbio uric acid Liquicolor kit (Boerne, TX, USA) and expressed as mg/dL. Serum NAG was determined colorimetrically as described by Luqmani et al.,Citation27 using Diagnostic FAR kit (Verona, Italy) and expressed as microunit/mL. Serum cytokines IL-1β and TNF-α as well as MCP-1 were determined according to the methods of solid phase enzyme-linked immunosorbent assay using rat kits (Biosource International, Tucson, AZ, USA) and microtiter plate reader, Fisher Biotech (Ebersberg, Germany) and expressed as pg/mL. Serum TC, TG, and HDL were determined colorimetrically according to Allian et al.,Citation28 Wahlefeld,Citation29 and Lopes-Virella et al.,Citation30 respectively. LDL was calculated mathematically according to Friedwald et al.,Citation31 using the following equation:
Plasma NO measured as nitrite was determined using Griess reagent according to the method of Moshage et al.Citation32 and expressed as μmol/L. The quantitative determination of cGMP in plasma was carried out according to Kojda et al.,Citation33 using cGMP radioimmunoassay kit (IPL Com., Radolfzell, Germany) and expressed as pmol/mL.
The stored kidneys were homogenized in Teflon-glass homogenizer with buffer containing 1.5% potassium chloride to obtain 1:10 (w/v) of whole homogenate to determine LPO and antioxidant enzyme activates in renal tissue. The homogenate was prepared for analysis by centrifugation at 18,000 × g (4°C) for 30 min.
The amount of produced malondialdehyde (MDA) was used as an index of LPO. MDA concentration was assayed according to a modified method of Ohkawa et al.,Citation34 based on reaction with thiobarbituric acid and expressed as nmol MDA/g tissue. Tissue superoxide anion was determined according to the modified method of Hassoun and Stohs,Citation35 following the principle of Babior et al.,Citation36 on the basis of cytochrome c reduction and was expressed as nmol cytochrome c reduced/min/g tissue. Measurement of tissue SOD activity was performed according to the method described by Sun et al.,Citation37 which consists of the inhibition of nitroblue tetrazolium reduction with xanthine–xanthine oxidase used as superoxide generator and expressed as U/mg protein. Tissue GSH concentration was measured by kinetic assay using a dithionitrobenzoic acid recycling methodCitation38 and expressed as μmol/mg tissue. The GSH-Px activity in renal tissue was determined according to Beutler,Citation39 based on oxidation of reduced GSH coupled to the appearance of NADPH by glutathione reductase measured at 37°C and 340 nm and expressed as U/g protein. CAT activity was determined by measuring the decomposition of hydrogen peroxide (H2O2) at 240 nm according to the method of AebiCitation40 and was expressed as U/g. Protein concentration was measured according to the method described by Lowry et al.Citation41
Statistical Analysis
The obtained data were statistically analyzed. The differences between groups were assessed by one-way analysis of variance (ANOVA), using the SPSS software (IBM, New York, USA). The values are expressed as mean ± SD. Intergroup comparisons were performed by Duncan’s multiple rank test using MSTAT-C computer program. Mean values followed by the same alphabetical letter are not significantly different. Differences were considered to be significant at p < 0.05.
Table 1. Effect of GSE on blood fractions of hypercholesterolemic rats treated with gentamicin.
RESULTS
Feeding healthy rats with 150 mg/kg/day GSE for 30 consecutive days had insignificant effects on blood fractions level as compared to the control group. However, injecting rats with 50 mg/kg GM twice daily for 15 consecutive days resulted in significant increases in serum levels of creatinine (288%), urea (110%), uric acid (141%), NAG (165%), IL-1β (67%), TNF-α (159%), and MCP-1 (115%) (). Also, significant increases were detected in serum levels of TC (13%), TG (64%), and LDL (84%) (); while significant attenuation was recorded in serum levels of HDL (25%) () and plasma NO (40%) as well as cGMP (50%) () as compared with the control group.
Feeding the GM group concomitantly with GSE and 15 days after produced significant protection against renal toxicity. The amelioration of renal toxicity was evidenced in significant reductions in the elevated serum levels of creatinine (61%), urea (35%), uric acid (40%), and NAG (51%) () in comparison with the GM group. In addition, GSE supplementation significantly reduced the elevated serum levels of IL-1β (28%), TNF-α (54%), MCP-1 (45%) () as well as serum TG (28%) and LDL (21%) () while significantly increased the attenuated serum level of HDL (24%) () and plasma NO (49%) as well as cGMP (71%) () as compared with the GM group.
The current results demonstrated that keeping rats on cholesterol-rich diet significantly elevated the serum levels of urea (25%), NAG (14%), IL-1β (23%), TNF-α (29%), MCP-1 (29%), TC (21%), TG (116%), and LDL (135%), while significantly attenuated serum HDL (26%) and plasma cGMP (21%) as compared with the control group. The fluctuations of serum creatinine, uric acid, and plasma NO in HC rats did not reach the level of significance when compared with the normal group.
Injecting HC rats with 50 mg/kg GM twice daily for 15 days caused highly significant elevations in the serum levels of creatinine (350%), urea (125%), uric acid (190%), NAG (187%), IL-1β (83%), TNF-α (171%), MCP-1 (132%) (), TC (28%), TG (143%), LDL (173%) (), while significantly attenuated serum HDL (33%) () and the plasma levels of NO (49%) and cGMP (56%) () as compared with the normal diet control group. On the other hand, feeding HC GM-treated rats with GSE produced significant amelioration against renal toxicity, which was manifested in significant reductions in serum creatinine (56%), urea (32%), uric acid (36%), and NAG (44%) as compared with the HC GM group. Moreover, GSE supplementation significantly attenuated the elevated serum levels of IL-1β (31%), TNF-α (51%), MCP-1 (47%), TC (10%), TG (29%), and LDL (31%), while significantly elevated the attenuated serum level of HDL (29%) and plasma NO (58%) as well as plasma cGMP (86%) as compared with the HC rats injected with GM.
Healthy rats that received GSE for 30 days had insignificant effects on both oxidative and antioxidative stress parameters of renal tissue as compared with the normal control. However, injecting rats with GM twice daily for 15 days caused marked increases in LPO (90%) and superoxide anion (198%), while significantly reduced the levels of SOD (55%), GSH (53%), GSH-Px (54%), and CAT (56%) () in renal tissue of rat compared with the normal control. On the other hand, supplementing GM group with 150 mg/kg/day GSE concomitantly with GM administration and 15 days after significantly attenuated the elevated levels of LPO (38%) and superoxide anion (54%) while significantly elevated the attenuated activity of antioxidant stress parameters, that is, SOD (71%), GSH (83%), GSH-Px (98%), and CAT (209%) in renal tissue of rats as compared with GM-treated rats.
The results illustrated in revealed that HC significantly raised oxidative stress levels, that is, LPO (12%) and superoxide anion (61%), while significantly attenuated antioxidative stress activities, that is, SOD (17%), GSH (30%), and CAT (8%) in the renal tissue of rats as compared with the normal diet control rats. Injecting HC rats with GM significantly elevated the levels of oxidative stress and markedly reduced the activities of antioxidant parameters in renal tissue of rats as compared with the normal control and other studied groups. Otherwise, feeding HC-injected GM rats 150 mg/kg/day GSE concomitantly with GM administration and 15 days after produced significant protection against renal toxicity which was evidenced by significant reductions in the elevated levels of oxidative stress parameters LPO (37%) and superoxide anion (49%), while significantly increased the attenuated antioxidant parameters levels, that is, SOD (74%), GSH (108%) GSH-Px (97%), and CAT (101%) in renal tissue of rats in comparison with the HC rats injected with GM group.
Figure 1. Effect of GSE on lipid profile of hypercholesterolemic rats treated with gentamicin. Columns followed by the same alphabetical letter for each parameter are not significantly different at p < 0.05. GSE, grape seed extract; GM, gentamicin group; HC, hypercholesterolemic group; TC, total cholesterol; TG, triglyceride; HDL, high-density lipoprotein; LDL, low-density lipoprotein.
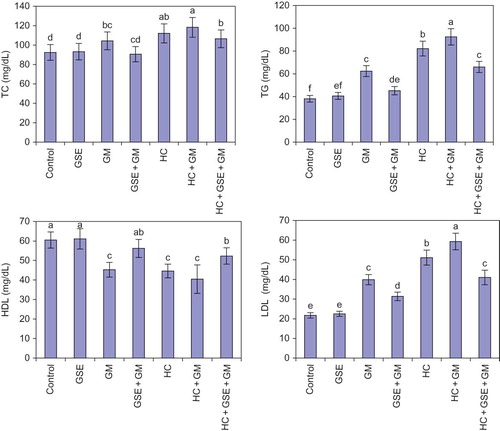
Figure 2. Effect of GSE on renal tissue fractions of hypercholesterolemic rats treated with gentamicin. Columns followed by the same alphabetical letter for each parameter are not significantly different at p < 0.05. GSE, grape seed extract; GM, gentamicin group; HC, hypercholesterolemic group; LPO, lipid peroxidation; SOD, superoxide dismutase; GSH, reduced glutathione; GSH-Px, glutathione peroxidase; CAT, catalase.
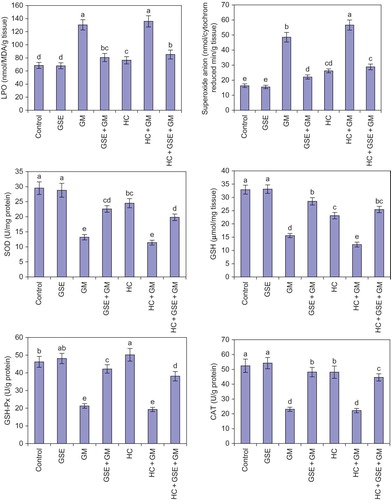
DISCUSSION
Recent interest in GSE has greatly increased due to its antioxidant capacity and free radical scavenging abilityCitation14 as well as being anti-inflammatory,Citation15 having platelet aggregation inhibiting and metal chelating properties,Citation18 and having possible beneficial implications in human health. This study investigates the possible renoprotective effects of GSE against oxidative stress, renal damage, and atherosclerosis induced by GM and HC in rats through the assessment of kidney biochemical parameters and lipid profile.
In this study, in the normal control and GSE groups, rats demonstrated normal renal functions (normal serum creatinine, urea, uric acid, and NAG). However, the toxic renal effects of GM were manifested in the GM group in significant increases in serum levels of creatinine, urea, uric acid, and NAG. Similar degrees of GM toxicity have been reported by different investigators.Citation1,42 The increases in urea and creatinine levels may indicate a reduction in the glomerular filtration rate (GFR) as a result of GM intoxication, since the serum concentration of these two parameters depends largely on the glomerular filtration.Citation43 NAG is a lysosomal enzyme widely distributed in body tissue; it is released into serum from cells by exocytosis or from the breakdown of cells.Citation44 The elevated serum NAG activity may be related to atherosclerosis, elevated serum cholesterol, increased uric acid,Citation45 and increased oxygen free radicals, which lead to destruction of lysosomal membrane with subsequent release of NAG enzyme.Citation46 Serum NAG activity has gained increased importance as an aid in the diagnosis of atherosclerosis.Citation45 The toxic renal effects of GM may be due to the interaction between molecular and subcellular components (lysosomes and mitochondria), where GM binds to the anionic phospholipids of cell membranes and gains access to the cell interior before binding to the subcellular organelles or being taken up by lysosomes. Accumulation of GM within the renal cortex is intimately related to the pathogenesis of renal failure.Citation47
This study demonstrated that GM exposure significantly elevated serum levels of IL-1β, TNF-α, and MCP-1. The inflammatory cytokine TNF-α itself causes injury to renal epithelial cells because it stimulates the production of ROS and produces oxidative stress by sensitizing infiltrating leukocytes.Citation48 TNF-α and IL-1β are proinflammatory cytokines and often elevated in parallel. They stimulate the production of one another and act synergistically to stimulate the production of other cytokines and chemokines.Citation49 A major chemotactic factor of monocytes is also induced by TNF-α and IL-1β in response to vascular injury and it is critical for the progression of atherosclerosis.Citation50 TNF-α appears to be involved in many types of inflammatory processes and has been negatively correlated with SOD, CAT, and renal tissue GSH.Citation51The obtained results showed that the administration of GM significantly elevated serum TC, TG, and LDL, while markedly decreased HDL as compared with the control group. Confirming these results, Rashid et al.Citation52 found that serum cholesterol was increased significantly in rats following GM exposure and also caused prominent damage in the kidney tissue. High serum TG levels have been reported to be an important risk factor as it influences lipid deposition and clotting mechanism.Citation53 The increase in serum LDL has also been pointed out as one of the risk factors for the development of atherosclerosis.Citation54 Elevation of serum cholesterol concentration played a major role in the progress of the GM-induced nephrotoxicity.Citation10 Therefore, the high lipid levels detected in GM-treated rats may be associated with the accumulation of filtered LDL fractions in the glomeruli, leading to a reduction in the glomerular filtration surface area.Citation55
When compared with normal control rats, GM injection induced significant decrease in both plasma NO and cGMP. This result may be due to the inhibition of endothelium-dependent vasodilatation.Citation56 NO with the heme moiety of soluble guanylyl cyclase leads to the formation of cGMP from GTP.Citation57
The toxic renal effects of GM observed in this study are manifested in significant elevation in MDA and superoxide anion production and significant reduction in GSH level and SOD, GSH-Px, and CAT activities. It is believed that reduced activity of one or more antioxidant systems because of the direct toxic effect of GM causes an increase of LPO and oxidative stress and consequently renal toxicity.Citation3,5
Feeding cholesterol-rich diet to rats induced significant increases in serum levels of urea, NAG, IL-1β, TNF-α, MCP-1, TC, TG, and LDL as well as MDA and superoxide anion levels in renal tissue, while significantly reduced the serum level HDL and plasma cGMP as well as enzymatic and nonenzymatic antioxidants (SOD, GSH, GSH-Px, and CAT) activities in renal tissue. In this connection, feeding rats with cholesterol-rich diet resulted in increased LDL level and LPO, and reduced CAT and SOD activities.Citation58 In addition, HC elevated TC, TGs, LPO coupled with decreased enzymatic and nonenzymatic antioxidants in renal tissue, which indicate the onset of oxidative changes in the renal tissue.Citation59 Moreover, AbdelhalimCitation2 concluded that HC produces ROS and other free radicals increasing TBARS, serum level and decreasing plasma enzymes SOD and GSH-Px activity which confirmed the results of this study. However, there was no significant difference (p < 0.05) in the levels of serum creatinine, uric acid, NAG, and plasma NO of both animals fed on the control diet and those fed on the HC diet.
The concurrent feeding of the cholesterol-enriched diet with the GM treatment resulted in significantly greater biochemical changes and functional exacerbation of glomerular disorder than those following GM alone. These results were manifested in significant increases in the serum levels of creatinine (350%), urea (125%), uric acid (190%), NAG (176%), IL-1β (83%), TNF-α (171%), and MCP-1 (132%) as compared with the control group. This abnormal concentration of these catabolites in serum is a clear indication of renal function impairment.Citation60,61 There were also significant elevations in the serum levels of TC (28% and 13%), TG (143% and 48%), and LDL (173% and 48%) as compared with the normal control and GM groups, respectively. The elevation in serum cholesterol concentration probably played a major role in the progression of the GM-induced renotoxicity.Citation10 HC rats injected with GM showed significant reductions in the plasma levels of NO (48% and 20%) and cGMP (56% and 12%) as compared with the normal control and GM groups, respectively. This result is in the same line with Pear et al.,Citation62 who reported that the reduced production of NO may be implicated in the hemodynamic changes of HC animals. The accelerated generation of ROS induced by HC was accompanied by the depletion of their specific scavengers (enzymatic and nonenzymatic antioxidants),Citation59 which are in harmony with the results of this study.
The results of this study indicate the ability of GSE to ameliorate GM-induced renotoxicity in normal diet and HC rats, as serum levels of creatinine, urea, uric acid, and NAG were decreased in both GM and HC + GM groups to be approximately near their normal levels. Similarly, different studies that tested the protective effect of GSE against chemical assaults in various organs using animal models showed that pre-exposure GSE rescued cells from toxic assaults, significantly protected genomic integrity, and minimized apoptotic, necrotic, and apocrotic cell deaths.Citation63 The amelioration of serum creatinine and urea levels due to supplementation of GSE indicated the increase in GFRs. Moreover, the reduction of serum NAG and uric acid in GM and GM HC groups due to administration of GSE indicated the protective effect of GSE against the toxicity of GM and HC.
The hypothesis that GSE exhibits anti-inflammatory properties has been supported by our findings where the intake of GSE significantly reduced the serum level of TNF-α, IL-1β, and MCP-1 of the rats exposed to GM and HC. TNF-α and IL-1β as proinflammatory cytokines are often changed in parallel and act synergistically in production of other cytokines and chemokines.Citation49 Through this connection, Terra et al.Citation15 suggested that GSE procyanidin reduced the expression of proinflammatory cytokines TNF-α and IL-6 and enhanced production of the anti-inflammatory cytokines adiponectin.
Administration of GSE markedly suppressed the elevation of serum TC, TG, and LDL, while increased the attenuated serum level of HDL in GM and HC + GM groups. These results are in agreement with the findings of Adisakwattana et al.Citation64 HDL may play a protective role through reversing cholesterol transport, enhancing the oxidation of LDL, and neutralizing the atherogenesis effect of oxidized LDL.Citation2 The anti-hyperlipidemic activities of GSE may act through the inhibition of lipid digestion and absorption. Supplementation of GSE reduces plasma lipid profiles by improving adipokine secretion and oxidative stressCitation65 and inhibiting pancreatic lipase, cholesterol esterase, cholesterol micellization, and bile acid binding.Citation64
This study showed that supplementation of GSE to GM or GM HC rats significantly elevated the suppressed plasma levels of NO and cGMP. These results are confirmed by the findings of Fitzpatrick et al.Citation66 GSE reduced platelet aggregation, releasing NO from endothelial cells, activating the guanylate cyclase, increasing the cGMP production, and finally inducing vascular relaxation.Citation67
Providing both GM and GM HC rats with GSE significantly lowered the elevated superoxide anion and LPO in renal tissue, while it markedly raised the attenuated level of GSH and the activities of SOD, GSH-Px, and CAT. Elevating GSH in renal tissue is essential for the regulation of cell functions, maintaining cell integrity, cell protection against variety of toxins such as free radicals and their accompanied oxidative injury due to its reducing properties, and participation in the cell metabolism.Citation68 Many studies have reported that the monomers catechin, epicatechin (monomeric flavonols), and procyanidins are the major phenolic compounds in GSE that show antioxidant activity.Citation13,14
The renoprotective effects of GSE intake may be due to its multiple effects on LPO, inflammatory mediators such as MCP-1, and cytokines such as TNF-α and IL-1β, enhanced NO release and cGMP, and improved lipid profile. From this point of view, the intake of GSE may be a feasible therapeutic strategy for prevention and treatment of patients with GM and HC-induced renal impairment. Further studies in both animals and humans are necessary to demonstrate the antioxidant properties and other effects of GSE on renal diseases. This investigation is helpful for understanding the mechanism of action of GSE and its active ingredients and also reveals the potential of GSE for use as a natural oral agent with both renotoxicity and HC effects.
Declaration of interest: The authors report no conflicts of interest. The authors alone are responsible for the content and writing of the paper.
REFERENCES
- Salem EA, Salem Neveen A, Kamel M, . Amelioration of gentamicin nephrotoxicity by green tea extract in uninephrectomized rats as a model of progressive renal failure. Ren Fail. 2010;32(10):1210–1215.
- Abdelhalim MA. The potential influence of high cholesterol diet-induced oxidative stress on composition and properties of blood cells in rabbits. Afr J Microbiol Res. 2010;4(9):836–843.
- Martinez-Salgado C, Lopez-Hernandez FJ, Lopez Novoa JM. Glomerular nephrotoxicity of aminoglycosides. Toxicol Appl Pharmacol. 2007;223:86–98.
- Appel GB, Neu HC. The nephrotoxicity of antimicrobial agents. N Engl J Med. 1997;269:663–670.
- Balakumar P, Rohilla A, Thangathirupathi A. Gentamicin-induced nephrotoxicity: Do we have a promising therapeutic approach to blunt it? Pharmacol Res. 2010;62:179–186.
- Chade AR, Porecel MR, Grande JP, . Mechanisms of renal structural alterations in combined hypercholesterolemia and renal artery stenosis. Arterioscler Thromb Biol. 2003;23(7):1295–1301.
- Montilla P, Espejo I, Mufioz MC, Bujalance I, Mufioz- Castafieda JR, Tunez I. Protective effects of red wine on oxidative. Stress and antioxidant enzyme activities in the brain and kidney induced by feeding high cholesterol in rats. Clin Nutr. 2006;25(1):146–153.
- Taneja D, Thompson J, Wilson P, . Reversibility of renal injury with cholesterol lowering in hyperlipidemic diabetic mice. J Lipid Res. 2010;51:1464–1470.
- Yang DW, Jia RH, Yang DP, Ding GH, Huang CX. Dietary hypercholesterolemia aggravates contrast media-induced nephrotoxicity. Chin Med J (Engl). 2004;117(4):542–546.
- Abu-Spetan KA, Abdel-Gayom AA. Effect of high dietary cholesterol on gentamicin-induced nephrotoxicity in rabbits. Arch Toxicol. 2001;75:284–290.
- Yilmaz Y, Toledo RT. Health aspects of functional grape seed constituents. Trends Food Sci Technol. 2004;15:422–443.
- Weber HA, Hadges AE, Guthrie JR, . Comparison of proanthocyanidins in commercial antioxidants: Grape seed and pine bark extracts. J Agric Food Chem. 2007;55:148–156.
- Oszmianski J, Wojdylo A, Lamer-Zarawska E, Swiader K. Antioxidant tannin from Rosaceae plant roots. Food Chem. 2007;100:579–583.
- Singh RP, Agarwal R. Mechanisms of action of novel agents for prostate cancer chemoprevention. Endocr Relat Cancer. 2006;13:751–778.
- Terra X, Montagut G, Bustos M, . Grape seed procyanidins prevent low-grade inflammation by modulating cytokine expression in rats fed a high-fat diet. J Nutr Biochem. 2009;20:210–218.
- Kaur M, Singh RP, Gu M, Agarwal R, Agarwal C. Grape seed extract inhibits in vitro and in vivo growth of human colorectal carcinoma cells. Clin Cancer Res. 2006;12:67–73.
- Mayer R, Stecher G, Waerzner R, . Proanthocyanidin target compounds as antibacterial agents. J Agr Food Chem. 2008;56:6955–6966.
- Bagchi D, Garg A, Krohn RL, Bahchi DJ, Balmoori J, Stohs SJ. Protective effects of grape seed proanthocyanidins dins and selected antioxidants against TPA-induced hepatic and brain lipid peroxidation and DNA fragmentation and peritoneal macrophage activation in mice. Gen Pharmacol. 1998;30:771–776.
- Fine AM. Oligomeric proanthocyanidin complexes, structure and phytopharmaceutical applications. Alter Med Rev. 2000;5:144–151.
- Yamakoshi J, Kataoka S, Koga T, Ariga T. Proanthocyanidin-rich extract from grape seeds attenuates the development of aortic atherosclerosis in cholesterol-fed rabbits. Atherosclerosis. 1999;142:139–149.
- Hemmati AA, Nazari Z, Samei M. A comparative study of grape seed extract and vitamin E effects on silica-induced pulmonary fibrosis in rats. Pulm Pharmacol Ther. 2008;21:668–674.
- Obatomi DK, Plummer DT. Renal damage caused by gentamicin a study of the effect in vitro using isolated rat proximal tubular fragments. Toxicol Lett. 1995;75:75–83.
- Zand T, Hoffman AH, Savilonis BJ, . Lipid deposition in rat aortas with intraluminal hemispherical plug stenosis: A morphological and biophysical study. Am J Pathol. 1999;155:85–92.
- Henry RJ. Clinical Chemistry, Principles and Technique. 2nd ed. New York: Harper and Row; 1974.
- Searcey RI, Reardon JI, Forenam JA. Enzymatic urea kits. Am J Med Technol. 1967;33:15–20.
- Haisman P, Muller BR. Glossary of Clinical Chemistry Terms. London: Butterworth; 1977:126.
- Luqmani Y, Temmim L, Memon A, . Measurement of serum N-acetyl β-glucosaminidase activity in patients with breast cancer. Acta Oncol. 2001;38(5):649–653.
- Allian C, Poon LS, Chan CS, Richmond W, Fu PC. Enzymatic determination of total serum cholesterol. Clin Chem. 1974;20:470–475.
- Wahlefeld AW. Methods of Enzymatic Analysis. London: Academic Press; 1974:1831–1835.
- Lopes-Virella MF, Stone P, Ellis S, Colell JA. Cholesterol determination in high-density lipoproteins separated by three different methods. Clin Chem. 1977;23(5):882–885.
- Friedwald WT, Levy RI, Friedrickson DS. Estimation of plasma and serum low density lipoprotein cholesterol concentration without use of preparaline ultracentrifuge. Clin Chem. 1972;18:499.
- Moshage H, Kok B, Huizenge JR, Jansen PLM. Nitrite and nitrate determination in plasma: A critical evaluation. Clin Chem. 1995;41(6):892–896.
- Kojda G, Kottenbery K, Nix P, Schluter KD, Piper HM, Noack E. Low increase in cGMP induced by organic nitrates and nitrovasodilators improves contractile response of rat ventricular myocytes. Circ Res. 1996;78:91–101.
- Ohkawa H, Ohishi N, Yahi K. Assay for lipid peroxides in animal tissues by thiobarbuturic acid reaction. Anal Biochem. 1979;95:351–358.
- Hassoun EA, Stohs SJ. Cadmium induced production of superoxide anion and nitric oxide, DNA single strand breaks and lactate dehydrogenase leakage in J 774 A-1 cultures. Toxicology. 1996;112(3):219–226.
- Babior BM, Kipnes RS, Curnutte ST. Biological defense mechanisms, the production by leukocytes of superoxide a potential bactericidal agent. J Clin Invest. 1973;52(3):741–744.
- Sun Y, Oberley LW, Li Y. A simple method for clinical assay of superoxide dismutase. Clin Chem. 1988;34(3):479–500.
- Ellman G. Tissue sulfhydryl groups. Arch Biochem Biophys. 1959;82:70–77.
- Beutler E. Red Cell Metabolism: A Manual of Biochemical Methods. New York: Grune & Stratton; 1975:67–69.
- Aebi H. Catalase. In: Bermeyer HU, ed. Methods in Enzymatic Analysis. New York: Academic press; 1983:276–286.
- Lowry OH, Rosebrough NI, Farr AL, Randall RJ. Protein measurement with folin phenol reagent. J Biol Chem. 1951;193:265–275.
- Khan SA, Priyamvada S, Farooq N, Khan MW, Yusufi AN. Protective effect of green tea extract on gentamicin induced nephrotoxicity and oxidative damage in rat kidney. Pharmacol Res. 2009;59(4):254–262.
- Gavin JB. Assessment of renal function. Md GR J. 1995; 23:102–105.
- Welman E, Selwyn AP, Peters TJ, Colbeek JF, Fox KM. Plasma lysosomal enzyme activity in acute myocardial infarction. Cardiovasc Res. 1978;12:99–109.
- Hashimoto R, Adachi H, Nishida H, Tsuruta M, Nomura G. Serum N-acetyl-β-D- glucosaminidase activity in predicting the development of hypertension. Hypertension. 1995;25:1311–1314.
- Li WG, Zhang XY, Wu YJ, Tian X. Anti-inflammatory effect and mechanism of proanthocyanidins from grape seeds. Acta Pharmacol Sin. 2001;22:1117–1120.
- Erdem A, Gundogan NU, Usubutun A. The protective effect of taurin against gentamicin-induced acute tubular necrosis in rats. Nephrol Dial Transplant. 2000;15:1175–1182.
- Ramesh G, Reaves WB. TNF-α mediates chemokine and cytokine expression and renal injury in cisplatin nephrotoxicity. J Clin Invest. 2002;11(6):835–842.
- Banas B, Luckow B, Moller M, . Chemokine and chemokine receptor expression in a novel human mesangial cell line. J Am Soc Nephrol. 1999;10(11):2314–2322.
- Funakoshi Y, Ichiki T, Shimokaw H. Rho-kinase mediates angiotensin II-induced monocyte chemo-attractant protein-1 expression in rat vascular smooth muscle cells. Hypertension. 2001;38(1):100–111.
- Akbulut G, Kilek ON, Kahraman A, Koken T, Serteser M. The correlation between tissue renal oxidative stress parameters and TNF-α levels in an experimental model of ischemia reperfusion injury in mice. Ulus Trauma Derg. 2005;11(1):11–16.
- Rashid F, Sheem MK, Bano B. Comparative effect of olive oil supplementation in combating gentamicin-induced nephrotoxicity in rats. Indian J Clin Biochem. 2005;20(1):109–114.
- Harnafi H, Aziz M, Amrani S. Sweet basil (Ocimum basilicum L.) improves lipid metabolism in hypercholesterolemic rats. Eur J Clin Nutr Metab. 2009;4(4):181–186.
- Getz GS, Reardon GA. Diet and murine atherosclerosis. Arterioscler Thromb Vasc Biol. 2006;26:242–249.
- Moawad Karima M. Possible prophylactic effect of vitamin E or lycopene treatment on renal toxicity induced by CCL, administration in albino rats. World J Zool. 2007;2(2):19–28.
- Buyukafsar K, Yazar A, Dusmez D, Ozturk H, Polat G, Levent A. Effect of trapidil: An antiplatelet and vasodilator agent on gentamicin-induced nephrotoxicity in rats. Pharmacol Res. 2001;44(4):321–328.
- Thomas G, Ramwell PW. Nitric oxide, donors and inhibitors. Basic Clin Pharmacol Chap. 2001;19:326–332.
- Visavodiya NP, Narasimhachary AVR. Hypercholesterolemic and antioxidant effects of Withania somnifera (Dunal) in hypercholesterolemic rats. Phytomedicine. 2007;14:136–142.
- Sudhahar V, Kumar SA, Varalkshmi P, Sujatha L. Protective effect of lupeol and lupeol linoleate in hypercholesterolemia associated renal damage. Mol Cell Biochem. 2008;317(1–2):11–20.
- Crook MA. The kidneys. In: Clinical Chemistry and Metabolic Medicine. 7th ed. London: Bookpower; 2007:36–56.
- Zanna H, Adeniji S, Shehu BB, Modu S, Ishaq GM. Effects of aqueous suspension of the root of Hyphened hepatica L. Mart on some indicators of liver and kidney function in rats. J Pharmacol Toxicol. 2008;3(4):330–334.
- Pear G, Blum M, Iaina A. Nitric oxide and acute renal failure. Nephron. 1996;73:375–381.
- El-Ashmawy IM, El- Nahas AF, Salama OM. Grape seed extract prevent gentamicin-induced nephrotoxicity and genotoxicity in bone marrow cells of cells. Basic Clin Pharmacol Toxicol. 2006;99:230–239.
- Adisakwattana S, Moonrat J, Srichairat S, . Lowering mechanisms of grape seed extract (Vitis vinifera L.) and its antihyperlidemic activity. J Med Plants Res. 2010;4(20):2113–2120.
- Deorde K, Teissedre PL, Sutra T, Ventura E, Cristol JP, Rouanet JM. Grape-seed procyanidins prevent low-grade inflammation by modulating cytokine expression in rats fed a high-fat diet. Mol Nutr Food Res. 2009;53:659–668.
- Fitzpatrick DF, Maggi DA, Fleming RC, O’Malley RM. Vasodilation procyanidins derived from grape seeds. Ann N Y Acad Sci. 2002;957:78–89.
- Naseri MKG, Hamidi MN, Heidari A. Vasorelaxatory effect of Vitis vinifera extract on rat aorta. Iranian J Pharm Res. 2005;2:93–99.
- Saad Abir A, Youssef MI, El-Shennawy Lamia K. Cisplatin-induced damage in kidney genomic DNA and nephrotoxicity in male rats: The protective effect of grape seed proanthocyanidin extract. Food Chem Toxicol. 2009;47:1449–1506.