Abstract
Platelet activation is traditionally quantified using turbidimetric aggregometry, which reflects integrin αIIbβ3 activity, an important determinant of platelet function during pathophysiological thrombus formation. However, aggregometry does not recreate the shear conditions prevailing during thrombosis in vivo. Here we describe novel whole-frame analysis of real-time video microscopy to quantify platelet adhesion receptor activity under shear in parallel-plate flow chambers. We demonstrate that the rate of change of surface coverage (designated Platelet Population Mobility, PM) quantifies platelet mobility. On collagen, PM falls exponentially to a low level, corresponding to firm platelet adhesion, while on other substrates, PM remains high. Different receptor-specific thrombogenic surfaces reveal that the PM time constant reflects real-time changes in integrins αIIbβ3 and α2β1 activity. This ensemble kinetic analysis has the potential to provide valuable diagnostic information about platelet thrombus formation with both academic and clinical applications.
Introduction
Since its development 50 years ago, turbidimetric (light transmission) platelet aggregometry has been the principal technique used to assess platelet activity in research and clinical settings [Citation1, Citation2]. More recently, flow cytometry [Citation3] has provided a measure of platelet activation by quantifying antibody binding to activated membrane receptors, while the PFA-100 [Citation4] offers a shear-dependent assessment of platelet function. However, neither aggregometry nor flow cytometry recreates the physiological conditions experienced by circulating platelets in vivo, and although the PFA-100 operates at shear, it is unable to characterize receptor-specific platelet function. None of these techniques reports the full repertoire of hemostatic responses to the multiple activatory and adhesive receptors that regulate platelet behavior.
Imaging of thrombus deposition in perfusion chambers is increasingly employed to investigate platelet pathophysiology under hemodynamic blood flow [Citation5]. Surface coverage (SC) is the parameter most frequently used to report platelet interaction with a thrombogenic surface. However, SC reveals little about platelet physiology as it takes no account of activation-dependent platelet-platelet interactions that are fundamental to thrombus formation. Where cooperativity exists between receptors, long recognized for the platelet collagen receptors [Citation6], their specific roles cannot be resolved using SC. A similar argument can be made for measurement of thrombus volume. Low volume could report an unactivated platelet monolayer or an activated thrombus with low adhesive potential. Improved methods of analysis are required to achieve a clear understanding.
Here, we introduce a novel image analysis technique that yields the parameter platelet population mobility (PM), which allows us to quantify platelet recruitment to a thrombogenic surface under flow.
DIOC6-stained human blood was perfused over thrombogenic surfaces (collagen fibers or synthetic collagen peptides) in a parallel plate flow chamber mounted on a laser scanning confocal microscope. Blood was perfused at a shear rate of 1000 s−1 for 5 minutes with images being acquired at the plane of the thrombogenic surface at 0.2 Hz.
Image series were thresholded empirically, and SC was calculated for each binary image. A duplicate image series was created, off-set by a single frame, from which the original series was subtracted on a frame-by-frame basis. The resultant SC for each frame was designated the change in surface distribution (dSD/dT, %). Expressing dSD/dT relative to SC (of the corresponding, unprocessed frame) produces dSD/dT/SC, an ensemble measurement of the rate of change of platelet capture. We designated this parameter PM (%). Data were fitted to an exponential decay model by non-linear regression. This simple mathematical procedure was performed rapidly using ImageJ (NIH) and Excel (Microsoft) (see Supplementary material for details).
Platelet population mobility was plotted against time (). Using both peptide VWF-III [Citation6] and collagen fiber substrates, PM achieved a steady state after an initial period of high platelet mobility. On collagen, PM fell rapidly from its initial value of 58.7 ± 3.6%, reaching a plateau of 9.1 ± 0.2% (), progressing from its initial, transient interaction to stable platelet adhesion. On VWF-III, PM was initially higher (102.4 ± 4.1%) and fell to a much higher steady state than on collagen (Plateau: 73.3 ± 1.0%), indicating that platelets interacted only transiently with this surface and did not achieve stable adhesion. This behavior indicates platelet rolling [Citation7].
Figure 1. PM quantifies platelet adhesion under flow conditions. (A) Whole blood was perfused at 1000 s−1 over type I collagen fibers (○) or VWF-III (•) and images were acquired at 0.2 Hz for 5 min. Image processing yielded PM measurements throughout the 5-min perfusion, which are plotted vs. time. PM curves were modeled as exponential decays, yielding the parameter of Plateau (B) (see Supplementary material for details). End-point image analysis yielded ZV50 (C).
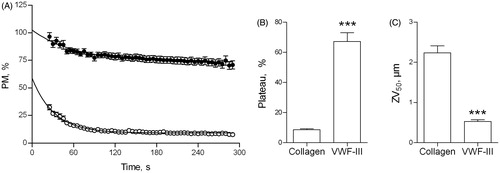
We recently described an end-point parameter of thrombus formation, ZV50, (a volume measurement) which reports platelet–platelet interactions and thus directly quantifies αIIbβ3 activity [Citation6]. An inverse relationship is apparent between ZV50 and PM, the latter being low on surfaces where ZV50 is high. However, while ZV50 provides a post hoc estimate, PM reports platelet integrin functionality dynamically, in real-time. On type I collagen, ZV50 (2.2 ± 0.2 µm) indicated that platelet activation and subsequent αIIbβ3-dependent platelet-platelet interactions had occurred by the end of the perfusion (). The corresponding low PM values are consistent with platelet activation. In contrast, ZV50 was lower on VWF-III (0.5 ± 0.04 µm), reflecting the presence of a monolayer of platelets, where αIIbβ3 is not activated and thrombus formation is absent [Citation6]. These data demonstrate that PM describes and quantifies the transition from non-activated, rolling platelets to activated, stationary platelets.
Within this overall framework, PM profiles can be used to resolve the role of the collagen-binding integrin α2β1 in thrombus deposition. Experiments were conducted using surfaces coated with a series of GxOGER-containing peptides with differing affinity for α2β1, in combination with VWF-III and the GpVI-specific collagen-related peptide (CRP) [Citation6]. The different PM profiles reflected the known affinities of the peptides for α2β1 (; Supplementary Figure S1). Perfusion of whole blood over surfaces including GPP10, the inert control peptide, or GAOGER, the low-affinity integrin ligand, resulted in gradual reductions of PM. PM fell more rapidly using surfaces including the higher affinity peptides, GFOGER and GLOGER, indicating stable adhesion, while the mid-affinity GMOGER yielded an intermediate PM profile. PM curves from the different surfaces converged, showing Plateau (an end-point parameter) to be unsuitable to resolve the role of α2β1 (). The differences in PM are reflected in significantly higher decay constants with GFOGER and GLOGER than on the lower affinity peptides, GMOGER, GAOGER or GPP10 ().
Figure 2. PM quantifies integrin activation under flow conditions. Whole blood was pre-treated with carrier (A), 300 µM DM-BAPTA-AM (B) or 5 µM GR144053 (C), before perfusion at 1000 s−1 over coverslips coated with combinations of CRP, VWF-III and one of a panel of integrin α2β1-adhesive peptides of varying affinities. PM was calculated as before. For clarity, only GFOGER, (○), GMOGER (•) and GPP10 (•) are shown. Other data sets (GLOGER and GAOGER) are shown in Supplementary Figure S2. End-point measurements were calculated as described in the text, Plateau (D), Decay constant (E) and ZV50 (F). Blood was pre-treated with: Carrier (□), 5 µM GR144053 (▪), 300 µM DM-BAPTA-AM (▪).
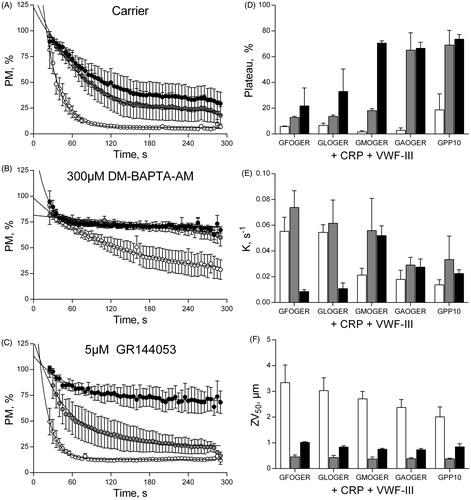
DM-BAPTA-AM treatment abolished stable adhesion on GMOGER, whereas adhesion was merely attenuated on GFOGER and GLOGER ( and S2). Therefore, an activation-dependent rise in intracellular calcium is an absolute requirement for platelet adhesion to the medium-affinity ligand, and mediates observable increases in integrin activity even to high-affinity peptides. PM thus reveals the rate at which α2β1 is activated in platelets adhering to a thrombogenic, activatory surface. This study provides quantitative evidence that the role of α2β1 under shear conditions is to accelerate recruitment of platelets to collagen surfaces.
Inhibition of αIIbβ3 with 5 µM GR144053 reduced ZV50 to ∼0.5 µm on all surfaces, consistent with the presence of a platelet monolayer (). On a surface containing CRP, VWF-III and GPP10, αIIbβ3 blockade caused the plateau of PM to increase from 21.5 ± 5.4% to 69.1 ± 11.4%, consistent with platelet rolling (). Plateau on surfaces containing the higher-affinity peptides, GFOGER and GLOGER, was insensitive to GR144053 treatment, while GMOGER supported intermediate behavior.
Hence, a high-affinity α2β1 ligand is necessary for stable platelet adhesion in the absence of αIIbβ3 activity, and as a corollary, αIIbβ3 contributes to stable adhesion to a surface that cannot bind α2β1. It follows that the PM profile on such a surface quantifies αIIbβ3 activity, further confirmed by the rise in plateau following DM-BAPTA-AM treatment (). Thus, on such surfaces, the PM profile is functionally similar to an aggregometry trace, in that it quantifies changes in the αIIbβ3 activation state in real time.
In conclusion, we demonstrate a novel imaging technique that offers parametric kinetic analysis of thrombus deposition. By manipulating platelet receptor function and the composition of the thrombogenic surface, we demonstrate that PM measurement can be used to report changes in the activity of integrins αIIbβ3 and α2β1. Although similar in some respects to aggregometry, PM profiling has the advantage of using whole blood under flow conditions that occur during pathogenic thrombus formation. Real-time, perfusion-based measurements are therefore less reductive and more direct. Additionally, the analysis is mathematically simple and quick and easy to perform, making our technique an alternative to aggregometry, but crucially under physiologically relevant flow conditions. PM profiling could be developed to analyze platelet function in a clinical setting using simpler, widefield fluorescence microscopy.
Contributions
N.P. and R.W.F. conceived this project. N.P. designed and conducted experiments, analyzed and interpreted data and wrote the manuscript. D.B. generated reagents. R.W.F. and D.J.P. analyzed and interpreted data and wrote the manuscript.
Supplementary material available online
Figure S1. Illustration of the subtraction of subsequent frames to yield a dynamic measure of platelet deposition on a thrombogenic surface.
Figure S2. PM quantifies the activation state of a2b1 and aIIbb3 under flow conditions.
Supplemental material can be viewed and downloaded at http://informahealthcare.com/plt
Acknowledgements
The authors thank Joanna-Marie Howes, Stephanie Jung, Ben Maddox, Masaaki Moroi and Andrew Thompson (University of Cambridge, UK) for useful feedback and discussions of this manuscript.
Declaration of interest
The authors declare no competing financial interests. This work was supported by the British Heart Foundation (RG/09/003/27122), the Medical Research Council (G0500707) and The Newton Trust.
References
- Born GVR, Cross MJ. The aggregation of blood platelets. J Physiol 1963;168:178–195
- Jarvis GE, Platelet aggregation: Turbidimetric measurement, in Gibbins JM, Mahaut-Smith MP (Eds). Platelets and megakaryocytes. Methods Mol Biol 2004;272:65–76
- Michelson A. Flow cytometry: A clinical test of platelet function. Blood 1996;87:4925–4936
- Harrison P. The role of PFA-100 testing in the investigation and management of haemostatic defects in children and adults. Br J Haematol 2005;130:3–10
- Van Kruchten R, Cosemans JMEM, Heemskerk JWM. Measurement of whole blood thrombus formation using parallel-plate flow chambers – a practical guide. Platelets 2012;23:229–242
- Pugh N, Simpson AMC, Smethurst PA, de Groot PG, Raynal N, Farndale R. Synergism between platelet collagen receptors defined using receptor-specific collagen-mimetic peptide substrata in flowing blood. Blood 2010;115:5069–5079
- Ruggeri ZM, Mendolicchio GL. Adhesion mechanisms in platelet function. Circ Res 2007;100:1673–1685