Abstract
Expression of epithelial-mesenchymal transition (EMT) markers has been detected clinically in benign prostatic hyperplasia (BPH) tissues. To understand the molecular basis, we investigated the role of stromal microenvironment in the progression of EMT in BPH cells. First, we used cell culture supernatant from normal prostate stromal WPMY-1 cells to provide supernatant-conditioned medium (WSCM) to culture the BPH-1 cell line. Then, the morphological changes and migratory capacity were detected in BPH-1 cells. The expression of EMT markers was examined in BPH-1 cells by Western blot and immunofluorescent analysis. Finally, to investigate the role of transforming growth factor beta 1 (TGF-β1) in this process, the WSCM-cultured cells were treated with monoclonal antibody against TGF-β1 to study its effect on EMT. We found that the morphology of BPH-1 cells changed to a spindle-like shape after cultured in WSCM, and the levels of E-cadherin and cytokeratin 5/8 (CK5/8) were significantly lower than the cells cultured in ordinary medium. These BPH-1 cells were also tested positive for mesenchymal markers vimentin and a-smooth muscle actin (SMA) as well as Snail. We also found WSCM can increase the migratory capacity of BPH-1 cells. In addition, when they were treated with anti-TGF-β1, upregulation of E-cadherin and CK5/8 levels was observed but no expression of vimentin, alpha-SMA or Snail was detected. Furthermore, phosphorylated-Smad3 expression in WSCM-cultured BPH-1 cells was also suppressed by anti-TGF-β1 treatment. Our results demonstrated that stromal cell supernatant was able to induce EMT in BPH-1 cells, possibly through secreting TGF-β1 to activate Smad signaling. Our results suggest novel molecular targets for clinical treatment of BPH by modification of stromal microenvironment through inhibiting TGF-β1/Smad expression.
Introduction
The pathogenesis of benign prostatic hyperplasia (BPH) is still largely unknown. For decades, BPH has been suggested as a proliferative stromal disease (Rohr & Bartsch, 1980). Strong experimental evidence suggests that prostatic stroma plays a crucial role in the development of BPH, which forms the epithelial-mesenchymal interaction theory in BPH pathogenesis (Cunha, Citation1994). Recently, Alonso-Magdalena et al. (Citation2009) found that regions of ductal epithelium where epithelial cells did not express E-cadherin or cytokeratin 8 (CK8) but vimentin had lost polarization and became spindle shaped, suggesting that EMT occurred in BPH. EMT is normally observed in tissue morphogenesis during embryonic development and is involved in the progression of carcinoma and some fibrotic disorders (Guarino, Citation1995). It is possible that BPH might involve in an accumulation of mesenchymal-like cells derived from the prostatic epithelium.
EMT occurs in certain physiological and pathological cases (van der Pluijm, Citation2011). During EMT, cells lose baso-apical polarization and acquire front-rear polarization, then the cytoskeleton undergoes remodeling (Voulgari & Pintzas, Citation2009). The most prominent manifestation is loss of epithelial markers such as E-cadherin and CK8 and expression of mesenchymal markers such as vimentin and a-smooth muscle actin (a-SMA) (Kalluri & Weinberg, Citation2009). Although evidence of EMT is suggested in BPH, the regulatory mechanism of EMT in the prostate remains to be elucidated. Transforming growth factor beta 1(TGF-β1) is also known as a inducer of EMT (Zavadil & Bottinger, Citation2005) and large amount of TGF-β1 has been shown to be released from stromal cells at a high concentration in the stromal microenvironment which affects the function of epithelial cells (Huang & Lee, Citation2003).
We hypothesized that the stromal microenvironment in BPH may play an important role in inducing EMT in prostate epithelial cells. To confirm this hypothesis, we used the prostate stromal cell line WPMY-1 to provide a stromal microenvironment to study its effect on BPH-1 cells in vitro.
Materials and methods
Cell culture
We obtained the human BPH-1 cell line from the George Whipple Lab (University of Rochester, Rochester, NY). Cells were maintained in RPMI-1640 medium (Hyclone) supplemented with 10% fetal bovine serum (Gibco, Washington, DC). WPMY-1 cells, a human prostatic stromal myofibroblast cell line, were obtained from American Type Culture Collection (Manassas, VA) and cultured in RPMI-1640 medium with 5% fetal bovine serum (Gibco). The two cell lines were maintained at 37 °C in a humidified atmosphere of 5% CO2/95% air.
Reagents and WPMY-1 supernatant-conditioned medium (WSCM)
WSCM was derived from WPMY-1 cell culture supernatant. WPMY-1 cells at ∼80% confluence were changed to fresh complete medium for 48 h. Supernatant was filtered through a 0.22-μm filter and spun in centrifuge tubes (5 ml per tube) for concentration to a crystalline state by a freeze-drying machine, then excess salt was removed by dialysis. The same amount of fresh BPH-1 complete medium was added to each tube for storage at −80 °C.
Monoclonal anti-TGF-β1 antibody and mouse IgG isotype control were purchased from R&D systems (Minneapolis, MN), and 500 μg/ml stock was reconstituted in phosphate buffered saline (PBS). For anti-TGF-β1 treatment, anti-TGF-β1 solution was added to the above WSCM and adjusted to a final concentration of 1 μg/ml.
Cell grouping and treatment
5 × 104 cells were seeded per well in six-well plates and cultured for 24 h, then the plates were divided into seven groups for treatment: BPH-1 cells cultured in BPH-1 complete medium; WSCM-cultured BPH-1 cells; WSCM-cultured BPH-1 cells with anti-TGF-β1 treatment; WSCM-cultured BPH-1 cells with mouse IgG; BPH-1 cells treated with anti-TGF-β1 antibody only; BPH-1 cells treated with mouse IgG only; WPMY-1 cells cultured in WPMY-1 complete medium.
Immunofluorescent staining
Cells were cultured on glass coverslips in six-well culture plates for seven days. Cells were triple-washed with PBS, fixed with 4% paraformaldehyde at room temperature for 30 min, then permeabilized in 0.5% Triton X-100/PBS for 5–10 min at room temperature and blocked in PBS containing 2% bovine serum albumin for 20 min at room temperature. After three washings with PBS for 5 min, cells were incubated with the monoclonal antibodies rabbit anti-E-cadherin and anti-vimentin (both 1:100; all Bioworld Technology, Atlanta, GA); mouse anti-CK5/8 (1:100; Santa Cruz Biotechnology, Santa Cruz, CA); and mouse anti-a-SMA and anti-Snail (both 1:100; both Abcam, Hong Kong) at 4 °C overnight. Cells were incubated with FITC-labeled secondary antibody (1:1000; Santa Cruz Biotechnology, Santa Cruz, CA) for 1 h at room temperature. Nuclear counterstaining involved use of DAPI (Molecular Probes, OR). Stained cells were viewed by confocal laser microscopy (PerkinElmer Life Sciences).
Western blot analysis
After each treatment, cells were washed twice in PBS and lysed with radioimmunoprecipitation assay buffer containing 1% protease inhibitors (Amresco, Cochran, GA). Protein concentration was determined by BCA protein assay (Amresco, Cochran, GA). The cell lystate was mixed with 5 × SDS-PAGE loading buffer (Amresco, Cochran, GA). Equivalent protein quantities were heated at 95 °C for 10 min before separation on precasted 7–15% SDS-polyacrylamide gels (Bio-Rad). Proteins were electotransferred to nitrocellulose membranes (Millipore, Atlanta, GA) that were blocked in Tris-buffered saline plus 0.05% Tween-20 (TBS-T) containing 5% non-fat dried milk for 1 h. The membranes were washed in TBS-T and incubated with primary monoclonal antibodies overnight at 4 °C in TBS-T containing 1% non-fat milk. The following primary antibodies were used: rabbit anti-E-cadherin and anti-vimentin (all 1:500; all Bioworld Technology, Atlanta, GA); mouse anti-CK5/8 (1:200; Santa Cruz Biotechnology, Santa Cruz, CA); mouse anti-alpha-SMA (1:200) and anti-Snail (1:1000; both Abcam, Hong Kong); rabbit anti-phosphorylated Smad3 (1:1000; Cell Signalling Technology, Boston, MA) and rabbit anti-GAPDH (1:1000; GE Healthcare, USA). After a washing in TBS-T buffer, membranes were incubated with goat anti-horseradish peroxidase-conjugated secondary antibody (1:1000; GE Healthcare, USA) for 1 h at room temperature in 1% non-fat milk dissolved in TBS-T. Membranes were then washed with TBS-T buffer, and signals were visualized by use of an enhanced chemiluminescence system (Amersham, GE Healthcare, USA). Bands were quantified by use of Quantity-one software (Bio-Rad). Protein level in each sample was relative to that of GAPDH.
Migration assay
After 5 days’ WSCM with/without anti-TGF-β1 treatment, the BPH-1 cells (5 × 104) were seeded in the top chamber of the 24-well transwells (Corning; 8 µm pore-size) and cultured with FBS-free media at 37 °C with 5% CO2 for 24 h. The insert membrane was then fixed with 75% ethanol at room temperature for 30 min, followed by washing with PBS. The membrane was stained with crystal violet for 30 min and washed with distilled water. Cells that did not crawl over were removed with cotton swabs. The chamber was cut and placed on a glass slide by a clean scalpel, and mineral oil was added to the slide. Photos were taken under 100× microscope and 5 fields were photographed from each membrane randomly. The number of cells in each field was counted by IPP software.
MTT assay
Five thousand BPH-1 cells were plated in each well of 24-well plates. The wells were divided into two groups: BPH-1 cultured with ordinary media; BPH-1 cultured with WSCM. The cells were collected on 0, 1, 3 and 5 days and MTT assay was performed as follows: 100 μl of 5 mg/ml MTT solution was added to each well including one set of wells with MTT solution but no cells (control). The cells were then incubated for 3 h at 37 °C incubator. The media was removed and 150 μl DMSO was added. The plates were covered with tinfoil and agitated on an orbital shaker for 15 min. The readings was recorded at absorbance at 570 nm.
Quantitative PCR
Total RNA was extracted from each cell-lines using Trizol (Invitrogen, Grand Island, NY). Reverse transcription was performed using the iScript reverse transcription kit (Bio-Rad). Quantitative real-time PCR (qRT-PCR) was conducted using a Bio-Rad CFX96 system with SYBR green to determine the level of mRNA expression of a gene of interest. Expression levels were normalized to the expression of GAPDH RNA. Primer sequence: Smad3 5′-GAGTAGAGACGCCAGTTCTACC-3′ and 5′-GGTTTGGAGAACCTGCGTCCAT-3′; GAPDH 5′-ATCCCATCACCATCTTCC- AGGAG-3′ and 5′-CCTGCTTCACCACCTTCTTGATG-3′.
Statistical analysis
Data are expressed as mean ± SEM from at least three independent experiments. Statistical analyses involved one-way ANOVA with SPSS 17.0 (SPSS Inc., Chicago, IL); p < 0.05 was considered statistically significant.
Results
WSCM induces EMT in BPH-1 cells
The prostate normal stromal cell line WPMY-1 appeared a spindle-shaped myofibroblast morphology which has been used for similar studies previously (Webber et al., Citation1999). The WPMY-1 cell culture supernatant was collected to provide the stromal microenvironment for BPH-1 cell culture in vitro. As shown in , BPH-1 cells cultured in ordinary medium formed cobblestone cell islands (left panel), a typical characteristic of epithelial cells, while WPMY-1 cells cultured alone showed spindle-shaped morphology (right panel). After 5 days cultured in WSCM, the morphology of BPH-1 cells appeared more like WPMY-1 cells with spindle shaped appearance (middle panel).
Figure 1. Evidence if WSCM-induced EMT in BPH-1 cells. Cells cultured in ordinary RPMI 1640 medium (BPH-1) and WPMY-1 supernatant (WSCM-BPH-1). WPMY-1 cells were photographed as a control. (A) Cells were cultured in different media for 5 days and photos were taken using a phase contrast microscope. Scale bar: 20 μm. (B) Western blot analysis of EMT-related proteins in BPH cells after 7 days’ culture period. Western blotting was performed on cell lysates using antibodies against EMT markers under different culture conditions. (C) Relative protein levels. Films were scanned and relative density of each band was calculated as ratio to GAPDH. Data are mean ± SD from 3 separate experiments. *p < 0.05. (D) Immunofluorescent staining of EMT marker proteins after 7 days’ cell culture. Immunofluorescent staining was performed as described in Materials and methods. Specific proteins were FITC labeled (green) and cell nuclei were stained with DAPI (blue, with exception of Snail). Photos were taken using a fluorescent microscope. (E) Migration assay. Crystal violet staining results showed WSCM can promote BPH-1 cell migration, Scale bar: 20 μm. *p < 0.05. (F) MTT assay. WSCM treatment did not alter the growth of BPH-1 cells after 1, 3 and 5 days of culture.
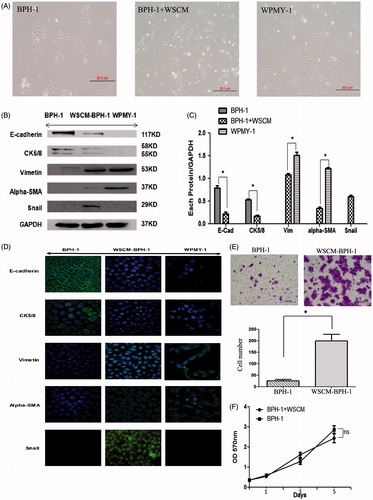
Western blotting analysis showed () that the expression of epithelial markers E-cadherin and CK5/8 was significantly downregulated in WSCM-cultured BPH-1 cells than in the cells cultured in ordinary medium (p < 0.05). In addition, positive expression of the mesenchymal markers vimentin and a-SMA and the EMT marker Snail was detected in the WSCM-cultured BPH-1 cells but not in the cells cultured in ordinary medium. Immunofluorescent staining results also agreed with the Western blotting that the WSCM-cultured BPH-1 cells lost E-cadherin and CK5/8 staining and acquired Vimentin, a-SMA and Snail expression (). These results suggest that culture medium from stromal cells is able to promote EMT transition in BPH-1 cells.
In addition, migration assay results showed that migratory capacity of BPH-1 cells was also increased after WSCM treatment (, right panel).
To investigate the effect of WSCM on cell proliferation, we performed MTT assay. As shown in , the growth rates of the BPH-1 cells were similar when cultured in ordinary medium and WSCM medium, respectively.
The WSCM-induced EMT is inhibited by anti-TGF-β1 treatment
Early studies suggested that the cytokine TGF-β1 is a potent inducer of EMT in various cancer cell types (Zavadil & Bottinger, Citation2005). To evaluate whether TGF-β1 played a role in the phenotypic and EMT marker changes observed in BPH-1 cells, we added anti-TGF-β1 antibody (1 μg/ml) in the WSCM medium and studied its effect on the WSCM-induced EMT. After 5 days’ cell culture, while the WSCM-cultured BPH-1 cells showed a spindle shape as observed previously (Representative images shown in , panel 2nd from left), adding anti-TGF-β1 to the culture medium reversed them to their original morphology (panel 2nd vs. 3rd from left).
Figure 2. Effect of TGF-β1 on WSCM-induced EMT in BPH-1 cells. BPH-1: BPH-1 cells cultured in ordinary culture condition; WSCM-BPH-1: BPH-1 cells cultured in WPMY-1 supernatant; BPH-1 + IgG: BPH-1 cells cultured in ordinary culture condition plus mouse IgG; BPH-1 + WSCM + IgG: BPH-1 cell cultured in WSCM medium plus mouse IgG. WSCM-anti-TGF-β1: BPH-1 cells cultured in WSCM medium plus anti-TGF-β1 antibody. WPMY-1: a prostate stromal ell line. (A) Morphological changes under different culture conditions. Scale bar: 20 μm. (B) Western blotting analysis of EMT marker protein expression. BPH-1 cells were lysed after cultured in different conditions. (C) Relative protein levels. Films were scanned and relative density of each band was calculated as ratio to GAPDH. Data are mean ± SD from 3 separate experiments. *p < 0.05. (D) Immunofluorescent staining of EMT marker proteins. Immunoflorescent staining was performed as described in Materials and methods. Specific proteins were FITC labeled (green) and cell nuclei were stained with DAPI (blue, with exception of Snail). Photos were taken using a fluorescent microscope. (E) Migration assay. Anti-TGF-β1 Ab was added into the culture system and detect the migration capability of BPH-1 cells induced by WSCM. Scale bar: 20 μm. *p < 0.05.
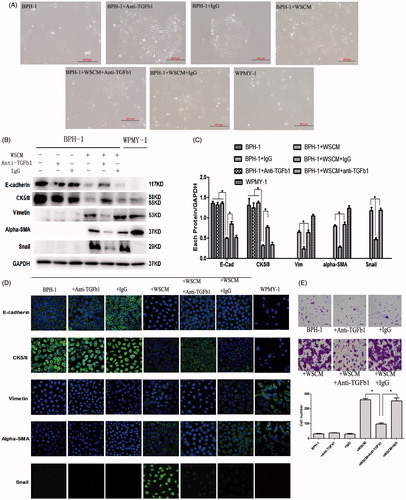
Western blotting analysis showed () that in WSCM-cultured BPH-1 cells, the expression of E-cadherin and CK5/8 was significantly upregulated with anti-TGF-β1 treatment (p < 0.05) but the expression of Vimentin, a-SMA or Snail was downregulated. In addition, immunofluorescence analysis also supports the Western blotting results that treatment with TGF-β1 led to suppression of vimentin, a-SMA and Snail and induction of E-cadherin and CK5/8 (). These lines of evidence indicate that TGF-β1 may play an important role in the WSCM-induced EMT in BPH-1 cells.
Migration assay was also consistent with the previous result, the inhibition of TGF-β1 can reverse the BPH-1 migratory potential induced by WSCM (, panel 5th vs. 4th & 6th from left).
Effect of TGF-β1 on its downstream mediator Smad3
To further investigate the role of TGF-β1 in the WSCM-induced EMT in BPH-1 cells, we next studied the involvement of Smad3, a TGF-β1 signalling-pathway receptor protein. Western blotting and Q-PCR were performed to detect Smad3 expression in BPH-1 cells (). After 1 day culture, phosphorylated-Smad3 was found increased in BPH-1 cells cultured in WSCM medium than in BPH-1 cells cultured in ordinary medium (). However, phosphorylated-Smad3 expression was significantly lower after treated with anti-TGF-β1 antibody in WSCM-cultured BPH-1 cells compared with the untreated cells (p < 0.05). But the total Smad3 showed no significantly change after 1 days’ WSCM treatment. After 7 days’ treatment, the mRNA level of total Smad3 were increased in BPH-1 cells cultured in WSCM medium, and this phenotype can be partly reversed by anti-TGF-β1 antibody (). To further check whether the neutralizing antibody used in the experiments was effective in blocking TGF-β1 activity, BPH cells were treated with higher concentrations (5 and 10 ng/ml) of TGF-β1 in the presence or absence of the neutralizing antibody and the expression of total and phosphorylated Smad3 proteins was examined by Western blotting (). These results further suggest that TGF-β1 may play an important role in WSCM-induced EMT which may be regulated by a Smad-dependent signalling pathway in BPH-1 cells.
Figure 3. Smad3 expression in BPH-1 cells. BPH-1: BPH-1 cells cultured in ordinary culture condition; BPH-1 + IgG: BPH-1 cells cultured in ordinary culture condition plus mouse IgG; BPH-1 + anti-TGF-β1: BPH-1 cells cultured in ordinary culture condition plus anti-TGF-β1 Ab; BPH-1 + WSCM: BPH-1 cells cultured in WPMY-1 supernatant; BPH-1 + WSCM + anti-TGF-β1: BPH-1 cells cultured in WSCM medium plus anti-TGF-β1 antibody; BPH-1 + WSCM + IgG: BPH-1 cell cultured in WSCM medium plus mouse IgG. (A) Western blot analysis of protein level of phosphorylated-Smad3 after 1 day treatment. (B) Q-PCR analysis of mRNA level of total Smad3 after 7 days’ treatment. (C) Smad3 expression in the cells treated with 5 ng/ml and 10 ng/ml TGF-β1.
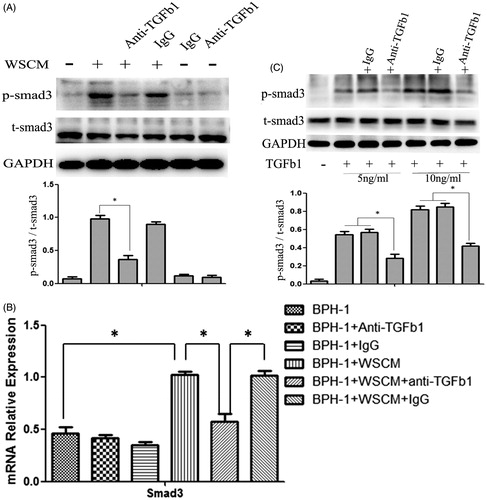
Discussion
With a recent theory of EMT proposed for BPH (Alonso-Magdalena et al., Citation2009), we aimed to explore the role of EMT regulatory mechanism in prostate epithelial cells. BPH is a classical age-related disease, and stromal cells play an important part in age-related tissue remodeling (Untergasser et al., Citation2005). Compared to young men, the components of stromal cells change in older men from mostly fibroblasts to a mixture of fibroblasts and myofibroblasts (Wong et al., Citation2011). Since myofibroblasts are more active than normal fibroblasts and can secrete aberrant factors that disrupt the architecture of the surrounding tissues and stimulate changes in neighboring epithelial cells, therefore, stromal cells derived from older men are more suitable for studying the mechanisms responsible for developing BPH than cells from young men (Gerdes et al., Citation2004; Tuxhorn et al., Citation2002). So in this study, we used a BPH cell line, BPH-1, which has been used in similar studies previously (Lu et al., Citation2012; Siejka et al., Citation2014), derived from an aged male to focus on the role of stromal microenvironment in BPH.
BPH-1 cells showed an elongated appearance after treatment with WSCM, and the levels of epithelial markers E-cadherin and CK5/8 were significantly decreased (). In addition, the WSCM-cultured BPH-1 cells expressed the mesenchymal markers Vimentin and a-SMA, as well as the EMT marker Snail (). Snail contains a zinc finger and is one of the best-characterized EMT inducers. It represses E-cadherin transcription by interacting with conserved E-boxes in the E-cadherin promoter (Comijn et al., 2001). Snail expression is high in cancer cells that lack E-cadherin and overexpression of Snail through transfection is able to induce EMT (Zeisberg & Neilson, Citation2009). In this study, we found that stromal environment was able to promote EMT in BPH-1 cells through induction of EMT factors which was accompanied by morphological changes. EMT can increase cell migration capability, so we further detected the function of BPH-1. By means of migration assay, we found that WSCM can promote the migratory potential of BPH-1 cells (). At the same time, we also detected the WSCM effect on the proliferation of BPH-1 cells. But the MTT result showed WSCM had no effect on the BPH-1 growth ().
When we added neutralizing anti-TGF-β1 antibody to WSCM culture medium to study the role of TGF-β1 in stromal microenvironment-induced EMT in BPH cells, we found () that as compared with the untreated control, anti-TGF-β1 treatment produced partial reversal of morphologic changes induced by WSCM. In addition, the expression of E-cadherin and CK5/8 was significantly increased, with only weak expression of vimentin, a-SMA and Snail. Migration assay was also consistent with the above result, the inhibition of TGF-β1 can reverse the BPH-1 migratory potential induced by WSCM. These results suggest that anti-TGF-β1 treatment was able to inhibit EMT induced by WSCM in BPH-1 cells. This partial inhibitory effect may be due to the fact that a relatively low concentration of the anti-TGF-β1 antibody (1 μg/ml) was used in this study. Nevertheless, our results indicate that TGF-β1 may play a key role in the stromal environment-induced EMT process in BPH-1 cells.
The TGF-β1-Smad-dependent pathway is one of key pathways of EMT (Moustakas & Heldin, Citation2007). TGF-β1 signals via two distinct receptor serine/threonine kinases: type I and II receptors. After ligand binding, the type II receptor transphosphorylates the type I receptor, which then phosphorylates cytoplasmic Smad2/3 proteins. Activated Smad2/3 then forms complexes with Smad4, which, upon entry to the nucleus, bind to chromatin and regulate the expression of genes that play critical roles in the control of EMT (Massagué et al., Citation2005). Several previous studies showed that EMT positively associated with Smad3 levels (Sjöblom et al., Citation2006). We also found () that phosphorylated-Smad3 expression was lower after anti-TGF-β1 treatment which was associated with partial aboligation of WSCM-induced EMT. These results implicate that it is possible that monoclonal anti-TGF-β1 antibody was able to inhibit the biological activity of TGF-β1 possibly through Smad3 signalling which in turn reverses the WSCM-induced EMT in BPH-1 cells. Previously a similar study on the role of TGF-β on prostate cells showed that it was able to induce EMT in tumourigenic derivatives of the BPH-1 line but not the non-tumourigenic parental line (Ao et al., Citation2006). However, a recent study on the BPH-1 cell line showed that TGF-β1 was able to induce EMT and this phenotype was associated with upregulation of the SNAI2/Slug as well as ZEB1 transcription factors (Slabáková et al., Citation2011), which agrees with our results presented in this study.
Conclusions
In summary, we explored the impact of stromal microenvironment on epithelial cells in vitro to provide molecular basis for EMT regulation in the prostate. We showed that supernatant of the prostatic stromal cell line WPMY-1 was able to induce EMT in BPH-1 cells, and this process was mediated by TGF-β1 possibly through Smad-dependent signalling. Although our results suggest molecular targets for future clinical treatment of BPH, further investigations are needed to confirm the role of TGF-β1 pathway.
Declaration of interest
The authors report no conflicts of interest. The authors alone are responsible for the content and writing of the paper.
References
- Alonso-Magdalena P, Brössner C, Reiner A, Cheng G, Sugiyama N. 2009. A role for epithelial-mesenchymal transition in the etiology of benign prostatic hyperplasia. Proc Natl Acad Sci USA 106:2859–2863
- Ao M, Williams K, Bhowmick N, Hayward S. 2006. Transforming growth factor-{beta} promotes invasion in tumorigenic but not in nontumorigenic human prostatic epithelial cells. Cancer Res 66:8007–8016
- Comijn J, Berx G, Vermassen P, Verschueren K, van Grunsven L. 2001. The two-handed E box binding zinc finger protein SIP1 downregulates E-cadherin and induces invasion. Mol Cell 7:1267–1278
- Cunha GR. 1994. Role of mesenchymal-epithelial interactions in normal and abnormal development of the mammary gland and prostate. Cancer 74:1030–1044
- Gerdes MJ, Larsen M, Dang TD, Ressler SJ, Tuxhorn JA. 2004. Regulation of rat prostate stromal cell myodifferentiation by androgen and TGF-β1β1. Prostate 58:299–307
- Guarino M. 1995. Epithelial-to-mesenchymal change of differentiation. From embryogenetic mechanism to pathological patterns. Histol Histopathol 10:171–184
- Huang X, Lee C. 2003. Regulation of stromal proliferation, growth arrest, differentiation and apoptosis in benign prostatic hyperplasia by TGF-β1beta. Front Biosci 8:s740–s749
- Lu T, Lin WJ, Izumi K. 2012. Targeting androgen receptor to suppress macrophage-induced EMT and benign prostatic hyperplasia (BPH) development. Mol Endocrinol 26:1707–1715
- Kalluri R, Weinberg RA. 2009. The basics of epithelial-mesenchymal transition. J Clin Invest 119:1420–1428
- Massagué J, Seoane J, Wotton D. 2005. Smad transcription factors. Genes Dev 19:2783–2810
- Moustakas A, Heldin CH. 2007. Signaling networks guiding epithelial-mesenchymal transitions during embryogenesis and cancer progression. Cancer Sci 98:1512–1520
- Rohr HP, Bartsch G. 1980. Human benign prostatic hyperplasia: A stromal disease? New perspectives by quantitative morphology. Urology 16:625–633
- Siejka A, Schally AV, Barabutis N. 2014. The effect of LHRH antagonist cetrorelix in crossover conditioned media from epithelial (BPH-1) and stromal (WPMY-1) prostate cells. Horm Metab Res 46:21–26
- Sjöblom T, Jones S, Wood LD, Parsons DW, Lin J. 2006. The consensus coding sequences of human breast and colorectal cancers. Science 314:268–274
- Slabáková E, Pernicová Z, Slavíčková E, Staršíchová A, Kozubík A, Souček K. 2011. TGF- beta 1-induced EMT of non-transformed prostate hyperplasia cells is characterized by early induction of SNAI2/Slug. The Prostate 70:1332–1343
- Tuxhorn JA, Ayala GE, Smith MJ, Smith VC, Dang TD, Rowley DR. 2002. Reactive stroma in human prostate cancer induction of myofibroblast phenotype and extracellular matrix remodeling. Clin Cancer Res 8:2912–2923
- Untergasser G, Madersbacher S, Berger P. 2005. Benign prostatic hyperplasia: age-related tissue-remodeling. Exp Gerontol 40:121–128
- van der Pluijm G. 2011. Epithelial plasticity, cancer stem cells and bone metastasis formation. Bone 48:37–43
- Voulgari A, Pintzas A. 2009. Epithelial-mesenchymal transition in cancer metastasis: Mechanisms, markers and strategies to overcome drug resistance in the clinic. Biochim Biophys Acta 1796:75–90
- Webber MM, Trakul N, Thraves PS, Bello-DeOcampo D, Chu WW. 1999. A human prostatic stromal myofibroblast cell line WPMY-1: A model for stromal-epithelial interactions in prostatic neoplasia. Carcinogenesis 20:1185–1192
- Wong YC, Yu SQ, Wang XH, Han BM, Zhao FJ. 2011. Differences in phenotype and gene expression of prostate stromal cells from patients of varying ages and their influence on tumour formation by prostate epithelial cells. Asian J Androl 6:1–10
- Zavadil J, Bottinger EP. 2005. TGF-β1beta and epithelial-to-mesenchymal transitions. Oncogene 24:5764–5774
- Zeisberg M, Neilson EG. 2009. Biomarkers for epithelial-mesenchymal transitions. J Clin Invest 119:1429–1437