Abstract
The ATP Binding Cassette transporter ABCB1 can export the neurotoxic peptide β-amyloid from endothelial cells that line the blood-brain barrier (BBB). This has the potential to lower cerebral levels of β-amyloid, but ABCB1 expression in the BBB appears to be progressively reduced in patients with Alzheimer’s disease. The surface density of many membrane proteins is regulated by ubiquitination catalyzed by ubiquitin E3 ligases. In brain capillaries of mice challenged with β-amyloid ex vivo, we show that the level of the ubiquitin ligase Nedd4 increases concomitant with reduction in Abcb1. In vitro we show that human ABCB1 is a substrate for human NEDD4-1 ligase. Recombinant ABCB1 was purified from Sf21 insect cells and incubated with recombinant NEDD4-1 purified from Escherichia coli. The treated ABCB1 had reduced mobility on SDS-PAGE, and mass spectrometry identified eight lysine residues, K271, K272, K575, K685, K877, K885, K887 and K1062 that were ubiquitinated by NEDD4-1. Molecular modelling showed that all of the residues are exposed on the surface of the intracellular domains of ABCB1. K877, K885 and K887 in particular, are located in the intracellular loop of transmembrane helix 10 (TMH10) in close proximity, in the tertiary fold, to a putative NEDD4-1 binding site in the intracellular helix extending from TMH12 (PxY motif, residues 996–998). Transient expression of NEDD4-1 in HEK293 Flp-In cells stably expressing ABCB1 was shown to reduce the surface density of the transporter. Together, the data identify this ubiquitin ligase as a potential target for intervention in the pathophysiology of Alzheimer’s disease.
Introduction
Alzheimer’s disease is characterized by the accumulation of β-amyloid within the brain. Reduced clearance of β-amyloid across the blood-brain barrier (BBB) is a contributory factor in this pathophysiology (Cirrito et al., Citation2005; Kuhnke et al., Citation2007; Zlokovic, Citation2008). Transcellular clearance across the endothelial cells of the BBB is thought to be a two-step process; at the abluminal membrane, the receptor LRP1 has been implicated in β-amyloid uptake from the cerebrospinal fluid (Deane et al., Citation2004), while at the luminal membrane, transport into the blood is mediated by the ATP Binding Cassette transporter, ABCB1 (also known as P-glycoprotein or MDR1) (Cirrito et al., Citation2005; Hartz et al., Citation2010; Vogelgesang et al., Citation2002). In patients with Alzheimer’s disease the level of ABCB1 expression and deposition of β-amyloid in the brain are inversely correlated, suggesting that progressive loss of ABCB1 expression allows β-amyloid to accumulate (Jeynes & Provias, Citation2011; Vogelgesang et al., Citation2002). This correlate is also true of the mouse model of Alzheimer’s disease that overexpresses human amyloid precursor protein (hAPP) (Cirrito et al., Citation2005; Karlnoski et al., Citation2009). In resected brain capillaries from wild-type mice, Abcb1 expression is reduced on challenge with the hAPP proteolytic fragment Aβ40 (Hartz et al., Citation2010). In the same study it was also shown that up-regulation of Abcb1 levels by activation of the PXR transcription factor can maintain Abcb1 levels, restore efflux and protect against β-amyloid accumulation in the brain.
The mechanism by which ABCB1 is lost from the BBB (with age and on challenge by Aβ40) is not known, but is likely to involve internalization of the transporter from the abluminal membrane. Internalization of proteins from the cell surface is commonly regulated by post-translational modification with ubiquitin, a 76-amino acid polypeptide that is attached covalently to lysine residues in the target protein. Ubiquitination requires the sequential activation of three enzymes: A ubiquitin-activating enzyme (E1), a ubiquitin-conjugating enzyme (E2), and a ubiquitin ligase (E3). Of these, it is the ubiquitin ligase that typically imparts specificity for the target protein and catalyzes the transfer of ubiquitin. NEDD4-1 is an ubiquitin E3 ligase that is expressed in many tissues. It comprises an amino terminal C2 domain, four WW domains and a carboxy-terminal HECT domain. The C2 domain binds membrane phospholipids which localizes NEDD4-1 to the periphery of cells in which it is expressed (Anan et al., Citation1998; Ingham et al., Citation2004). The class I, WW domains (named for the presence of pairs of highly conserved tryptophan residues 20–22 amino acids apart) bind to PxY motifs in the target protein although these ligases can also target proteins indirectly through an adaptor protein (Sudol et al., Citation1995; Sudol & Hunter, Citation2000), and the HECT domain catalyzes the transfer of ubiquitin from the activated E3 ligase to the target protein (Rotin & Kumar, Citation2009).
In the current study, we test the hypothesis that human ABCB1 is a substrate for the NEDD4-1 ubiquitin ligase and also examine its effect on surface density of ABCB1 in cultured cells. The data suggest that the level of ABCB1 protein in the Alzheimer brain is subject to ubiquitin-mediated internalization catalyzed by a member of the NEDD4 family.
Methods
Materials
Dulbecco’s modified eagle medium (DMEM), DMEM/F-12, fetal bovine serum (FBS), phosphate buffered saline (PBS) and Sf-900™ II SFM medium were purchased from Life Technologies (Paisley, UK). n-Dodecyl-β-D-maltoside was purchased from Merck Serono (Feltham, UK). Lipids were purchased from Avanti Polar Lipids (Alabaster, AL). Ni-NTA agarose was from Qiagen (Manchester, UK). Plasmid pBacPAK9 was from Clontech (Saint-Germain-en-Laye, France) and pcDNA5/FRT and pIZ-V5His were from Life Technologies (Paisley, UK). The baculoviral genome of ProFold™-ER1 was sourced from AB vector (San Diego, CA). Flp-In™ 293 cells were purchased from Life Technologies (Paisley, UK). Mouse anti-ABCB1 antibodies C219 and 4E3 were from Cambridge Bioscience (Cambridge, UK) and AbD Serotec (Oxford, UK), respectively. HRP-conjugated and R-phycoerythrin-conjugated AF488 anti-mouse secondary antibodies were from Dako (Ely, UK). Antibodies against Nedd4 (corresponding to the WW2 domain, amino acids 395–462 of rat Nedd4) and β-actin were from Abcam (Cambridge, MA). Human Aβ40 and all other chemicals were from Sigma-Aldrich (Poole, UK, or St Louis, MO).
Animals
All animal protocols were approved by the Institutional Animal Care and Use Committees (IACUC) of the University of Minnesota (IACUC protocol #1110A05865; PI: Anika M.S. Hartz) and carried out in strict accordance with AAALAC regulations, the US Department of Agriculture Animal Welfare Act, and the Guide for the Care and Use of Laboratory Animals of the NIH. Male transgenic mice overexpressing human amyloid precursor protein (hAPP) [Tg2576 strain; 129S6.Cg-Tg(APPSWE)2576Kha] and corresponding wild-type mice were purchased from Taconic Farms (Germantown, NY). All mice were single-housed and kept under controlled environmental conditions (23 °C, 35% relative humidity; 12-h light/dark cycle).
Isolation of capillaries from mouse brain
Brain capillaries were isolated as described previously (Hartz et al., Citation2010). Mice were euthanized by CO2 inhalation and decapitated. Brains were removed, dissected, and homogenized in ice cold PBS buffer (2.7 mM KCl, 1.46 mM KH2PO4, 136.9 mM NaCl, and 8.1 mM Na2HPO4 supplemented with 5 mM D-glucose and 1 mM sodium pyruvate, pH 7.4). Ficoll® was added to the minced brain to a final concentration of 15% and the Ficoll®/brain homogenate mixture was centrifuged at 5800 g for 20 min at 4 °C. The capillary pellet was resuspended in 1% BSA, and the capillary suspension was passed over a glass bead column. Brain capillaries adhering to the glass beads were collected by gentle agitation in 1% BSA. Brain capillaries were washed with BSA-free PBS and used for experiments.
Western blotting
Protein expression levels in brain capillaries were analyzed by Western blotting as described previously (Hartz et al., Citation2010). Brain capillaries were homogenized in lysis buffer (Sigma, St Louis, MO) containing Complete® protease inhibitor (Roche, Mannheim, Germany). Homogenized samples were centrifuged at 10 000 g for 15 min at 4 °C and denucleated supernatants were centrifuged at 100 000 g for 90 min at 4 °C to obtain brain capillary membranes. Brain capillary membranes were resuspendend in buffer containing protease inhibitors and stored at −80 °C. Western blots were performed using the Invitrogen NuPage™ Bis-Tris electrophoresis and blotting system (Invitrogen, Carlsbad, CA). After electrophoresis and protein transfer, membranes were blocked and incubated overnight with the primary antibody indicated. Membranes were washed and incubated with horseradish peroxidase-conjugated ImmunoPure secondary IgG (1:15 000; Pierce, Rockford, IL) for 1 h. Proteins were detected using SuperSignal West Pico Chemoluminescent Substrate (Pierce, Rockford, IL) and visualized using a BioRad Gel Doc 2000™ gel documentation system (BioRad, Hercules, CA). QuantityOne 1-D software (version 4.6.9; Bio-Rad Laboratories) was used for densitometric analysis of band intensities. Data were normalized to β-actin and presented as means ± SEM. To evaluate differences between controls and treated groups, the data were analyzed by two-tailed unpaired Student’s t-test using Microsoft Excel™ 2010. Differences were considered to be statistically significant at p < 0.05.
Plasmid, baculoviral vectors and cell lines
The human ABCB1-6His cDNA (described previously in Taylor et al., Citation2001) was modified by site-directed mutagenesis to introduce an AgeI restriction site in place of the translational-stop codon and subcloned into the vector pIZ-V5His to encode a 12-histidine carboxy-terminal tag. The ABCB1-12His cDNA was then subcloned into the pBacPAK9 baculoviral transfer vector to generate pBacPAK9-ABCB1-12His. The full cDNA was sequenced and the carboxy-terminus of the recombinant transporter confirmed as ABCB1-1277TKRQ-GHHHHHHTGHHHHHH1295. pcDNA3-NEDD4-1-mCherry was constructed using a full-length human NEDD4-1 IMAGE clone (clone 8862584, Source Bioscience) inserted into pcDNA3 with mCherry as an N-terminal fusion. Sf21 insect cells were co-transfected with pBacPAK9-ABCB1-12His and ProFold™-ER1 to generate the baculovirus ProFold-ABCB1-12His by homologous recombination in the cultured cells, as described by the supplier (AB vector; San Diego, CA). The ABCB1-12His cDNA was subcloned into the pcDNA5/FRT vector to generate pcDNA5/FRT-ABCB1-12His. Flp-In™ 293 cells (Life Technologies, Paisley, UK) were transfected with pcDNA5/FRT-ABCB1-12His. Transfectants were selected for hygromycin resistance and colonies cloned to generate a stable Flp-In-ABCB1-12His cell line.
Insect cell culture
Sf21 insect cells were cultured in suspension in Sf-900™ II SFM medium supplemented with penicillin G and streptomycin. Cultures were maintained at 27 °C in an orbital shaker at 120 rpm. Sf21 cells infected with ProFold-ABCB1-12His were cultured for 72 h prior to harvesting by centrifugation.
Mammalian cell culture
Flp-In-ABCB1-12His cells were grown as a monolayer in DMEM with Glutamax™, supplemented with 10% FBS and incubated in 5% CO2 in a humidified incubator at 37 °C. Maintenance of the integrated plasmid DNA was ensured by culturing in the presence of 100 μg/ml hygromycin B.
Purification of ABCB1
Crude membrane fractions were prepared from infected Sf21 insect cells as described previously (Martin et al., Citation2007). ABCB1 was solubilized in mixed micelles of n-dodecyl-β-D-maltoside and Escherichia coli lipids supplemented with cholesterol, and purified by Ni-NTA affinity chromatography by virtue of the engineered carboxy-terminal 12 × histidine tag, essentially as described previously (Ritchie et al., Citation2009), with the following modifications. Initial binding to the resin was performed in the presence of 10 mM imidazole. The resin was washed four times with 20 bed volumes of wash buffer containing incremental concentrations of imidazole (40, 80, 100 and 120 mM), and eluted with 500 mM imidazole. Buffer exchange to remove the imidazole was achieved by multiple concentration/dilution cycles using an Amicon® Ultra-4 Centrifugal Filter Unit (100 kDa cut-off; Merck Millipore, DE). Purification was monitored by SDS-PAGE. Proteins were separated on a 7.5% resolving gel and stained with colloidal blue.
Purification of NEDD4-1
GST-tagged human ubiquitin ligase NEDD4-1 was expressed in E. coli and purified by glutathione affinity chromatography, as described previously (Sullivan et al., Citation2007).
Ubiquitination reaction
Purified ABCB1 (20 μg in 50 μl final volume) was mixed with 5 μg of methylated ubiquitin (to limit modification to mono-ubiquitination), 100 ng of ubiquitin-activating enzyme (E1; Boston Biochem, Cambridge, MA), 100 ng of Ubc1 ubiquitin-conjugating enzyme (E2) (Sullivan et al., Citation2007) and 20 μl of NEDD4-1 at a concentration of 500 ng/μl in a reaction solution of 50 mM Tris pH 7.4, 10 mM ATP, 10 mM MgCl2 and incubated at 37 °C for 2 h. In a control sample, methylated ubiquitin was omitted. The reaction was stopped after 2 h by addition of 50 μl of SDS sample loading buffer. The sample was electrophoresed through an 8% Precise™ tris-glycine gel (Thermo Fisher Scientific, UK), blotted and ABCB1 detected with antibody, C219.
Mass spectrometry
ABCB1 was excised from the tris-glycine gel and digested using trypsin. The tryptic fragments were dissolved in 0.1% formic acid and separated by reverse-phase liquid chromatography. Electrospray mass spectrometry was carried out on a LTQ Orbitrap Velos ETD mass spectrometer (Thermo Fisher Scientific) at Birmingham Science City Translational Medicine facility. The data were analyzed by SearchGUI (Vaudel et al., Citation2011) and peptide-shaker (Barsnes et al., Citation2011; Vaudel et al., Citation2011).
Flow cytometry
Flp-In-ABCB1-12His cells were transfected transiently with pcDNA3-NEDD4-1-mCherry as described previously (Dixon et al., Citation2000). The transfected cells and a mock transfected control population were harvested 48 h post transfection and washed with DMEM/F12 (Life Technologies, Paisley UK) supplemented with 1% FBS. The live cells were stained for surface ABCB1 using saturating amounts of the ABCB1-specific primary antibody 4E3 and R-phycoerythrin-conjugated goat anti-mouse AF488 secondary antibodies, as described previously (Zolnerciks et al., Citation2007). Fluorescence data were acquired on a FACScan flow cytometer (Becton Dickinson), gating for 10,000 cells of normal size and granularity. mCherry fluorescence was detected in FL-2 channel and AF488 was detected in the FL-1 channel. Data were analyzed using the FlowJo software package (Tree Star, OR).
Results
Challenge with Aβ40 increases Nedd4 and reduces Abcb1 expression in the BBB of mice
Intact brain capillaries were isolated from the brains of 3-month-old wild-type and hAPP mice and analyzed for Abcb1 and Nedd4-family ubiquitin ligase expression. Western analysis of capillary membranes showed the presence of Nedd4 in wild-type mice (). In capillaries from hAPP mice Nedd4 protein expression was increased by 32% ± 9.8 (p < 0.03; n = 3) after normalization to β-actin levels. Exposing isolated capillaries from wild-type animals to human Aβ40 also increased Nedd4 protein expression by 42% ± 5.7 (p < 0.002; n = 3) compared to the untreated control. The increase in Nedd4 expression was concomitant with a reduction in Abcb1 levels by 42% ± 0.5 (p < 0.001; n = 3) (). These changes in the expression levels of the two proteins were evident within 6 h suggesting that Nedd4 directly tags Abcb1 with ubiquitin to mediate Aβ-mediated reduction of the transporter in Alzheimer’s disease.
Figure 1. (A) Representative Western analysis of protein lysates prepared from isolated brain capillaries from matched wild-type and hAPP mice at age 3 months, and (B) capillaries of wild-type mice before and after challenge with 100 nM human Aβ40. (C) Purification profile by metal affinity chromatography of human ABCB1. Lanes 1: Membrane fraction (100 μg of protein or 0.25% of volume); 2: Solubilized membrane fraction (0.25%); 3: Column flow-through (0.25%); 4–13: Washes from 20–200 mM imidazole in 20 mM increments (each 4% of volume); 14: EDTA 50 mM (4% of volume). (D) Western analysis of purified ABCB1 before and after ubiquitination. NEDD4-1 reaction mixture in the absence (lane 1) and presence (lane 2) of methylated ubiquitin.
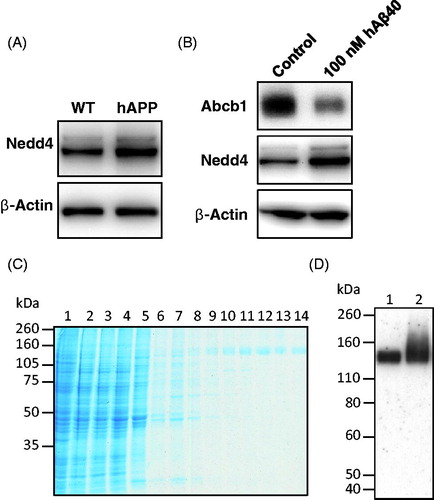
Human ABCB1 is a substrate of NEDD4-1
To test whether human ABCB1 is a substrate for human NEDD4-1 ubiquitin ligase we engineered a 12 × histidine tag onto the carboxy-terminus of the transporter. The recombinant transporter was expressed in insect cells, membranes were fractionated, and the proteins solubilized using non-denaturing n-Dodecyl-β-D-maltoside. The solubilized recombinant ABCB1 was purified by nickel-affinity chromatography (). In the preparative purification the final wash included 120 mM imidazole (lane 9), and the bound ABCB1 was eluted with 500 mM imidazole (equivalent to the combined lanes 10–14). The recovered protein was 94% pure and we have shown previously that it can be reconstituted into liposomes to regain drug-stimulated ATPase activity thus the native fold is preserved in n-dodecyl-β-D-maltoside (Ritchie et al., Citation2009). However, for the current purpose, the purified protein was buffer exchanged to remove the imidazole and incubated with NEDD4-1 in a reaction mixture that also contained ubiquitin E1 and E2 enzymes and recombinant ubiquitin. The mobility of the treated ABCB1 protein, but not the control sample from which ubiquitin was omitted, was retarded on SDS-PAGE suggesting that the treated ABCB1 is modified directly with ubiquitin and that NEDD4-1 recognizes ABCB1 as a substrate ().
Identification of the ubiquitination sites
Treated and untreated ABCB1 were excised from a polyacrylamide gel and analyzed by linear ion trap quadrupole with orbitrap mass spectrometry. The data confirmed the purification of ABCB1 and covered 82% of the protein sequence. Fragments and individual amino acids missing from the analysis covered amino acids 114–136, I147, 211–231, 292–329, 405–416, 460–467, 544–547, 579–580, K681, 703–734, 833–864, K888, 1015–1047, 1184–1192 and 1221–1222. Eight lysine residues (K271, K272, K575, K685, K877, K885, K887, K1062) were found to be ubiquitinated specifically in the sample treated with NEDD4-1 (). The positions of seven of the eight modified lysines were mapped onto a homology model of the closed conformation of the transporter based largely on the Sav1866 crystal structure from Staphylococcus aureus (Zolnerciks et al., Citation2014), and also on the open conformation described by the model of Abcb1a from Mus musculus (in which all eight lysines are conserved; Aller et al., Citation2009). K685 could not be modelled because it is in the linker region of ABCB1 between nucleotide-binding domain 1 (NBD1) and transmembrane domain 2 (TMD2). This region was not resolved in the Abcb1a crystal structure and is absent from Sav1866 (Sav1866 is a half transporter with one TMD and one NBD which functions as a homodimer, whereas ABCB1 is a four-domain polypeptide). All of the seven lysines mapped are located on the exposed surface of the cytosolic moiety and are largely on the same face of the transporter (, green spheres). Three of the lysine residues, K877, K885 and K887 are located in the intracellular loop extending from transmembrane helix 10 (TMH10) and in very close proximity to a unique PxY motif (P996-D997-Y998) in the intracellular loop of TMH12 which may function as a binding site for NEDD4-1 (, magenta spheres). It is possible that all eight lysines are accessible to NEDD4-1 docked at this binding site, because K685 must also be on the same face, close to the first resolved residue in the amino-terminal region of TMD2 indicated by the yellow spheres on the blue domains in .
Figure 2. Cartoon representation of two conformations of ABCB1. (A) Homology model based on Sav1866 (pdb 2HYD) from S. aureus. (B) Model of mouse Abcb1a (pbd 35GU). In each case the domains are coloured from the amino terminus as follows: TMD1, red; NBD1, orange; TMD2, blue; NBD2, cyan. The side chains of the putative NEDD4-1 binding motif (PxY) are shown as magenta spheres (P996 and Y998). The ubiquitinated lysines are shown as green spheres. The linker region linking NBD1 to TMD2 could not be modelled in either conformation therefore the precise position of K685 is not known but must be close to the first resolved residue in the amino-terminal region of TMD2, which in the closed conformation is W698, and in the open conformation is equivalent to L688 of human ABCB1. The C-alphas of W698 and the equivalent of L688 are shown as yellow spheres in the blue domain.
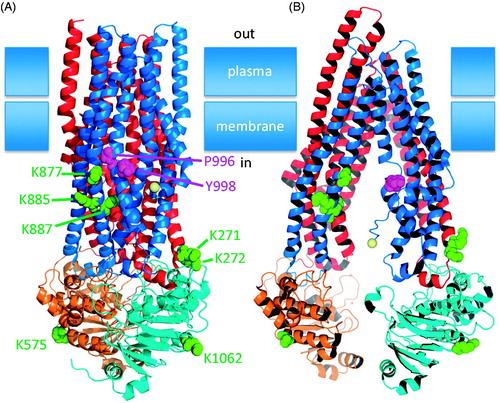
Table 1. ABCB1 peptides ubiquitinated by NEDD4-1.
NEDD4-1 expression decreases surface ABCB1 levels
To investigate the effect of NEDD4-1 on the surface density of ABCB1 we generated a stable cell line in which a single copy of the ABCB1-12His cDNA under the control of the CMV promoter was inserted into the genome of Flp-In HEK293 cells. The Flp-In-ABCB1-12His cells express a uniform level of ABCB1 allowing the surface density of ABCB1 to be measured and the effect of introduced NEDD4-1 to be tested. Surface density of ABCB1 was measured in live cells by flow cytometry after staining with saturating concentrations of anti-ABCB1 antibody (4E3) which recognizes an extracellular epitope of the transporter. Flp-In-ABCB1-12His cells were transfected transiently with pcDNA3-NEDD4-1-mCherry, or mock transfected, and stained with 4E3. Two-colour flow cytometry showed that the cherry-coloured cells that express NEDD4-1 had less ABCB1 on the cell surface ().
Figure 3. Flow cytometric analysis of ABCB1 in the plasma membrane of Flp-In-ABCB1-12His cells. Left dotplot, cells were gated for normal size and granularity (the same gate was used for all populations); Middle dotplot, two colour analysis of mock-transfected cells; Right dotplot, two colour analysis of cells transfected transiently with pcDNA3-NEDD4-1-mCherry. Each dot represents a single cell and the density of cells is colour coded from blue (few cells) to red (many cells). The numbers represent the percentage of cells in a given gate, and the coloured letters define the analytical gates used to plot the histogram. The histogram shows ABCB1 expression in NEDD4-1-expressing (orange and red) and non-expressing cells (blue and green). Histogram colour coding is consistent with the lettering in the dotplots.
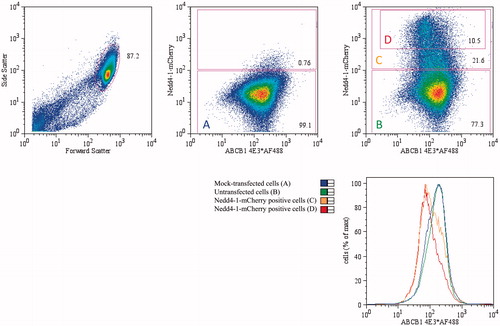
Discussion
Transgenic mice engineered to overexpress human amyloid precursor protein develop cognitive impairment with age that correlates with the accumulation of Aβ40 and Aβ42 in the brain (Hartz et al., Citation2010; Hsiao et al., Citation1996). ABCB1, a primary active, drug pump of the luminal membrane of the BBB endothelium is an important component of the chemical barrier and has been shown to efflux Aβ40 and Aβ42 into the blood stream. In mouse BBB capillaries ex vivo, challenge with Aβ40 significantly lowers the level of total Abcb1 within a few hours (Hartz et al., Citation2010). We show here that ubiquitin ligases of the Nedd4 family are also elevated in this tissue over the same timeframe, implicating their involvement in ubiquitin-mediated degradation of the transporter. To test this further we purified recombinant human ABCB1 and NEDD4-1 and showed that the transporter is indeed a substrate for the ubiquitin ligase. Eight lysine residues of ABCB1 were ubiquitinated by NEDD4-1 and these mapped essentially onto one face of the transporter. In particular, a cluster of lysines (K877, K885, K887 and K685) are in close proximity to a PxY motif, which forms a kink in the intracellular α-helix extending into the cytosol from TMH12. We propose that this PxY motif is a likely binding site for the WW domains of NEDD4-1. The inverse correlation between the expression of the transporter and the ubiquitin ligase was recapitulated in cell culture in which we showed that the density of human ABCB1 in the plasma membrane was reduced when NEDD4-1 was transiently-expressed in Flp-In-ABCB1-12His cells.
The density of most, if not all, proteins in the plasma membrane likely involves a combination of factors including synthesis (transcription and translation), maturation (folding and post-translational modification), trafficking to the membrane, recycling to and from endosomal compartments, and degradation. Of most relevance to the current study is the reported long half-life of the ABCB1 protein. Various studies in different cell types have measured the half-life of ABCB1 and reported wide ranging values from 72 h (Richert et al., Citation1988; Yoshimura et al., Citation1989), 24 h (Mickley et al., Citation1989) to the shortest report of 14–17 h (Muller et al., Citation1995). In comparison, the processing and trafficking of nascent ABCB1 to the plasma membrane is relatively fast with the nascent protein reported to have a half-life of 45 min on the pathway to maturity (Yoshimura et al., Citation1989). If these characteristics also describe ABCB1 in BBB endothelial cells then the rapid reduction in ABCB1 protein level observed on challenge with Aβ40 would require a significant lowering of the half-life. The data presented herein suggest the following mechanism as most likely; Aβ40 induces the expression and activation of NEDD4-1, which binds to the PxY motif of ABCB1. The ligase ubiquitinates the transporter thus tagging it for internalization from the membrane and degradation. Integral membrane proteins internalized from the plasma membrane are most commonly degraded in the lysosome. This would be consistent with the reported co-localization of ABCB1 and the lysosomal marker LAMP-2 (Fu & Roufogalis, Citation2007); however, we cannot rule out at present a proteasomal degradation pathway which has also been reported for ABCB1 (Katayama et al., Citation2013; Zhang et al., Citation2004).
A number of questions remain to be addressed. For example, the mechanism of induction and regulation of NEDD4-family ligases in BBB endothelial cells, and whether ubiquitination also inhibits the function of the transporter, remain unknown. Nevertheless, the proposed mechanism fits well with the available data and suggests NEDD4-family ubiquitin ligases may present a therapeutic target to help retain ABCB1 expression at the BBB in Alzheimer brains, and mediate clearance of Aβ40 and Aβ42 from the brain compartment.
Acknowledgements
The project was supported by the Medical Research Council UK (grant number MC_U120088463 awarded to Kenneth J. Linton) and the National Institute on Aging (grant number 1R01AG039621 awarded to Anika M. S. Hartz). The content is solely the responsibility of the authors and does not necessarily represent the official views of the National Institute on Aging or the National Institutes of Health.
Declaration of interest
The authors report no conflicts of interest. The authors alone are responsible for the content and writing of the paper.
References
- Aller SG, Yu J, Ward A, Weng Y, Chittaboina S, Zhuo R, et al. 2009. Structure of P-glycoprotein reveals a molecular basis for poly-specific drug binding. Science 323:1718–1722
- Anan T, Nagata Y, Koga H, Honda Y, Yabuki N, Miyamoto C, et al. 1998. Human ubiquitin-protein ligase Nedd4: expression, subcellular localization and selective interaction with ubiquitin-conjugating enzymes. Genes to Cells 3:751–763
- Barsnes H, Vaudel M, Colaert N, Helsens K, Sickmann A, Berven FS, Martens L. 2011. compomics-utilities: an open-source Java library for computational proteomics. BMC Bioinformatics 12:70
- Cirrito JR, Deane R, Fagan AM, Spinner ML, Parsadanian M, Finn MB, et al. 2005. P-glycoprotein deficiency at the blood-brain barrier increases amyloid-beta deposition in an Alzheimer disease mouse model. J Clin Investigation 115:3285–3290
- Deane R, Wu Z, Sagare A, Davis J, Du Yan S, Hamm K, et al. 2004. LRP/amyloid beta-peptide interaction mediates differential brain efflux of Abeta isoforms. Neuron 43:333–344
- Dixon PH, Weerasekera N, Linton KJ, Donaldson O, Chambers J, Egginton E, et al. 2000. Heterozygous MDR3 missense mutation associated with intrahepatic cholestasis of pregnancy: evidence for a defect in protein trafficking. Human Molec Genetics 9:1209–1217
- Fu D, Roufogalis BD. 2007. Actin disruption inhibits endosomal traffic of P-glycoprotein-EGFP and resistance to daunorubicin accumulation. Am J Physiol Cell Physiol 292:C1543–C1552
- Hartz AM, Miller DS, Bauer B. 2010. Restoring blood-brain barrier P-glycoprotein reduces brain amyloid-beta in a mouse model of Alzheimer’s disease. Molec Pharmacol 77:715–723
- Hsiao K, Chapman P, Nilsen S, Eckman C, Harigaya Y, Younkin S, et al. 1996. Correlative memory deficits, Abeta elevation, and amyloid plaques in transgenic mice. Science 274:99–102
- Ingham RJ, Gish G, Pawson T. 2004. The Nedd4 family of E3 ubiquitin ligases: Functional diversity within a common modular architecture. Oncogene 23:1972–1984
- Jeynes B, Provias J. 2011. An investigation into the role of P-glycoprotein in Alzheimer’s disease lesion pathogenesis. Neurosci Lett 487:389–393
- Karlnoski RA, Rosenthal A, Kobayashi D, Pons J, Alamed J, Mercer M, et al. 2009. Suppression of amyloid deposition leads to long-term reductions in Alzheimer’s pathologies in Tg2576 mice. J Neurosci 29:4964–4971
- Katayama K, Noguchi K, Sugimoto Y. 2013. FBXO15 regulates P-glycoprotein/ABCB1 expression through the ubiquitin-proteasome pathway in cancer cells. Cancer Sci 104:694–702
- Kuhnke D, Jedlitschky G, Grube M, Krohn M, Jucker M, Mosyagin I, et al. 2007. MDR1-P-Glycoprotein (ABCB1) mediates transport of Alzheimer’s amyloid-beta peptides – implications for the mechanisms of Abeta clearance at the blood-brain barrier. Brain Pathol 17:347–353
- Martin CA, Longman E, Wooding C, Hoosdally SJ, Ali S, Aitman TJ, et al. 2007. Cd36, a class B scavenger receptor, functions as a monomer to bind acetylated and oxidized low-density lipoproteins. Protein Sci 16:2531–2541
- Mickley LA, Bates SE, Richert ND, Currier S, Tanaka S, Foss F, et al. 1989. Modulation of the expression of a multidrug resistance gene (mdr-1/P-glycoprotein) by differentiating agents. J Biolog Chem 264:18031–18040
- Muller C, Laurent G, Ling V. 1995. P-glycoprotein stability is affected by serum deprivation and high cell density in multidrug-resistant cells. J Cellular Physiol 163:538–544
- Richert ND, Aldwin L, Nitecki D, Gottesman MM, Pastan I. 1988. Stability and covalent modification of P-glycoprotein in multidrug-resistant KB cells. Biochemistry 27:7607–7613
- Ritchie TK, Grinkova YV, Bayburt TH, Denisov IG, Zolnerciks JK, Atkins WM, Sligar SG. 2009. Chapter 11: Reconstitution of membrane proteins in phospholipid bilayer nanodiscs. Meth Enzymol 464:211–231
- Rotin D, Kumar S. 2009. Physiological functions of the HECT family of ubiquitin ligases. Nat Rev Mol Cell Biol 10:398–409
- Sudol M, Chen HI, Bougeret C, Einbond A, Bork P. 1995. Characterization of a novel protein-binding module – the WW domain. FEBS Lett 369:67–71
- Sudol M, Hunter T. 2000. NeW wrinkles for an old domain. Cell 103:1001–1004
- Sullivan JA, Lewis MJ, Nikko E, Pelham HR. 2007. Multiple interactions drive adaptor-mediated recruitment of the ubiquitin ligase rsp5 to membrane proteins in vivo and in vitro. Molecul Biol Cell 18:2429–2440
- Taylor AM, Storm J, Soceneantu L, Linton KJ, Gabriel M, Martin C, et al. 2001. Detailed characterization of cysteine-less P-glycoprotein reveals subtle pharmacological differences in function from wild-type protein. Br J Pharmacol 134:1609–1618
- Vaudel M, Barsnes H, Berven FS, Sickmann A, Martens L. 2011. SearchGUI: An open-source graphical user interface for simultaneous OMSSA and X!Tandem searches. Proteomics 11:996–999
- Vogelgesang S, Cascorbi I, Schroeder E, Pahnke J, Kroemer HK, Siegmund W, et al. 2002. Deposition of Alzheimer’s beta-amyloid is inversely correlated with P-glycoprotein expression in the brains of elderly non-demented humans. Pharmacogenetics 12:535–541
- Yoshimura A, Kuwazuru Y, Sumizawa T, Ikeda S, Ichikawa M, Usagawa T, Akiyama S. 1989. Biosynthesis, processing and half-life of P-glycoprotein in a human multidrug-resistant KB cell. Biochim et Biophys Acta 992:307–314
- Zhang Z, Wu JY, Hait WN, Yang JM. 2004. Regulation of the stability of P-glycoprotein by ubiquitination. Molec Pharmacol 66:395–403
- Zlokovic BV. 2008. The blood-brain barrier in health and chronic neurodegenerative disorders. Neuron 57:178–201
- Zolnerciks JK, Akkaya BG, Snippe M, Chiba P, Seelig A, Linton KJ. 2014. The Q loops of the human multidrug resistance transporter ABCB1 are necessary to couple drug binding to the ATP catalytic cycle. FASEB J 28:4335–4346
- Zolnerciks JK, Wooding C, Linton KJ. 2007. Evidence for a Sav1866-like architecture for the human multidrug transporter P-glycoprotein. FASEB J 21:3937–3948