Abstract
We examined whether female mink with low (LS) and high (HS) occurrence of stereotypic behaviour differ in their adrenocortical activity in baseline conditions or in response to immobilisation (Experiment 1), handling, adrenocorticotropic hormone (ACTH) challenge (Experiment 2) and excretion of circulating cortisol (Experiment 3). Faeces are the predominating excretory route of cortisol (83%), with peak concentrations after 4.2 h (urine: 3.4 h). Faecal cortisol metabolites (FCMs) reflected changes in relation to handling/ACTH challenge. In Experiment 1 (n = 162), HS mink had approximately 54% higher baseline level of FCM than LS mink (P < 0.001), with markedly elevated FCM on the days after an immobilisation stressor. In Experiment 2 (n = 48), LS and HS mink did not differ in adrenocortical activity after an ACTH challenge. However, HS mink reacted more in response to handling, evident in the FCM level 4–20 h after the handling (P = 0.001). In Experiment 3 (n = 16), the excretion of infused 3H-cortisol did not differ between LS and HS mink. Stereotypic behaviour is concurrent with higher baseline concentrations of FCM, which cannot be explained by a greater adrenocortical reactivity or a different excretion of the circulating cortisol. Instead, we conclude that mink with a high level of stereotypic behaviour have a greater perception of stress, or increased sensitivity to stressors at the pituitary level.
Introduction
In the assessment of stress experienced by individuals, hypothalamic–pituitary–adrenal (HPA)-axis responses are central, and can be included in the evaluation of animal welfare (Mormede et al. Citation2007). The active HPA-axis hormone in the circulation is cortisol in mink, cattle, sheep, horse, pig, fox, dog, cat, fish and primates, and corticosterone in mice, rat, rabbit and birds (Palme et al. Citation2005; Mormede et al. Citation2007). The baseline concentrations of these glucocorticoids (GC) in the blood are not easily obtained since the capture of one animal may arouse other not yet sampled animals housed nearby (Pedersen Citation1992), and the handling and blood sampling itself may act as a stressor, eliciting secretion of GC into the circulation. Therefore, examination of HPA-axis hormones in other biological fluids, such as urine and faeces sampled non-invasively, was introduced. In several species (e.g. pigs, sheep, dogs, elephants, hares), the main elimination route of GC is via the urine. However, excretion via faeces also occurs, and represents in some species (e.g. cats, rats, squirrels) the predominant excretory route for GC (Palme et al. Citation1996, Citation2005; Lepschy et al. Citation2007; Bosson et al. Citation2009; Dantzer et al. Citation2010). In a variety of species, the measurement of faecal cortisol/corticosterone metabolites was proven useful to evaluate adrenocortical activity (Möstl and Palme Citation2002; Touma and Palme Citation2005). In a preliminary experiment in mink, faecal cortisol metabolite (FCM) levels increased after injections of adrenocorticotropic hormone (ACTH; Malmkvist et al. Citation2004). Additionally, FCM levels were reduced following long-term enriched housing concurrent with a reduction in abnormal behaviour in comparison to a non-enriched control group (Hansen et al. Citation2007). Other studies have evaluated HPA-axis responses in mink based on non-invasive measurement of urinary cortisol (Bildsøe et al. Citation1991; Madej et al. Citation1992; Mason et al. Citation2001). Detailed information, however, about metabolism and excretion of circulating cortisol in mink has not been described previously.
Abnormal behaviour in farmed mink includes fur-chewing and stereotypic behaviour. Stereotypic behaviour is repeated in uniform patterns of movement without any obvious function. Stereotypic behaviour sometimes develops in animals kept in barren environments, and individuals that perform stereotypic behaviour were suggested to thereby reduce their adrenocortical response to stressors (Mason and Latham Citation2004). There is a genetic component in the proneness to perform these types of abnormal behaviour (fur chewing: Malmkvist and Hansen Citation2001; stereotypic behaviour: Jeppesen et al. Citation2004). It is possible to select for or against the performance of stereotypies, with an estimated heritability of 0.3 in mink (Hansen et al. Citation2010). Traditionally, the occurrence of abnormal behaviour is used as one indicator of reduced welfare in a particular environment (Mason Citation1991). Based on previous findings, however, the link between stereotypic behaviour and a stress response is equivocal. Zanella et al. (Citation1998) reported lower plasma cortisol concentrations in mink showing a high level of stereotypy, using a low number of euthanised animals. Bildsøe et al. (Citation1991) showed lower baseline levels of urinary cortisol metabolites in high stereotypy mink than in low stereotypy mink. In contrast to these results, higher concentration of FCM in mink from a high stereotyping breeding line was reported (Svendsen et al. Citation2007). There would be a complication in the use HPA-axis hormone metabolites in either faeces or urine as an indicator of stress load, if low and high stereotyping individuals differ in their cortisol metabolism and main excretion route. Therefore, aiming to investigate the link between stereotypic behaviour and stress further, we compared groups of female mink showing low (LS) or high (HS) stereotypy levels with regard to their adrenocortical activity in baseline conditions and in response to immobilisation (Experiment 1), handling and ACTH injection (Experiment 2), in addition to the route and time course of 3H-cortisol excretion (Experiment 3). We tested the following hypotheses: first, based upon results from previous studies using FCMs, we hypothesised that the basal HPA-axis output is higher in HS than in LS mink. Second, we tested whether a standardised acute stressor will lead to elevated adrenocortical responses of similar magnitude and duration in HS and LS mink. Finally, we hypothesised that HS mink have a higher sensitivity to ACTH at the adrenal cortex, or excrete cortisol from the circulation at a higher rate or in greater amount into faeces or urine, thus explaining the higher cortisol levels previously found in HS mink.
Materials and methods
Animals
We used female mink from two lines selected either against (low stereotypic behaviour level, LS) or for (high stereotypic behaviour level, HS) the occurrence of stereotypic behaviour for five generations. The mink were housed individually at the research farm of Aarhus University in wire cages (W: 30 cm, H: 45 cm, L: 91 cm) connected to a straw-covered wooden nest box (W: 28 cm, H: 20 cm, L: 23 cm). In total, 102 LS and 127 HS female mink were available. They had delivered and raised their first litter during the spring and summer of their first year of life. The mink were observed from the feed gangway running between two rows of cages. Each cage contained one mink. Six cages could be observed at a time. The observer stood still in front of the cages for 2 min and noted whether the mink performed stereotypic behaviour or not at the end of the 2 min period. Then the observer moved to the next set of six cages to be observed. The scanning observations were performed during 4 consecutive days by one observer. Fifteen scanning observations were performed each day evenly distributed between 09:00 and 14:00 h. Thus, the scanning interval was 20 min. Feeding was postponed to 14:00 h during observations. The frequency of stereotypic behaviour in 60 scanning observations conducted in mid October was 7.7% in HS-line mink and 0.8% in LS-line mink. In one or more of the 60 scanning observations, 63.0% of the line HS and 19.6% of the line LS mink performed stereotypic behaviour. Stereotypic behaviour was defined as a uniform pattern of movement without any obvious function, repeated a minimum of five times without interruption, e.g. somersaults or fixed pacing with head oriented towards the cage wall. For further information about these breeding lines, see Svendsen et al. (Citation2007) and Hansen et al. (Citation2010). Water was available ad libitum from a drinking nipple in each cage. The mink were fed commercial wet feed (approximately 190 kcal/100 g with protein 34%, fat 50% and carbohydrate 16% of convertible energy) daily on the top of the cage in amounts close to ad libitum. Feeding time was around 11:00 h.
Experiment 1: Baseline FCM levels and response to an immobilisation challenge
All 229 mink kept under standard farm conditions participated in Experiment 1. In the data analysis, however, we only included HS-line mink observed performing stereotypic behaviour in the HS group (n = 80) and LS-line mink observed free from stereotypic behaviour in the LS group (n = 82). We collected one fresh sample of faeces non-invasively for 6 days (Days 1, 2, 3, 4, 9 and 10) during early October between 14:00 and 20:00 h. We obtained samples from 86 to 98% of the LS and HS mink per sampling day. Faecal samples (0.5 g) were frozen within 3 h after delivery and stored at − 21°C until analysis. On Day 2 between 09:00 and 10:00 h, all mink were exposed to an immobilisation challenge, consisting of being restricted for 15 min in a wired trapping cage with a moveable bottom (W: 10 cm, H: 12 cm, L: 47 cm; Hedensted-Group, Hedensted, Denmark). Mink are trapped when they have to be moved around at the farm, e.g. at weaning; so the mink all have brief experience of the trapping cage, however, without being restricted by the moveable bottom locked in a lifted position. They experienced the immobilisation for the first time in their life as part of this experiment.
Experiment 2: Handling and ACTH challenge
For this experiment, 24 LS and 24 HS mink were used. They were chosen from among the HS mink that positively performed stereotypic behaviour, and from among the LS mink that did not perform stereotypic behaviour in the preceding 60 scanning observations. Half of the animals (12 LS and 12 HS) were exposed to handling in connection with the blood sampling only (control), whereas the other half (ACTH challenge) were injected i.m. with 0.15 ml synthetic ACTH (0.25 mg/ml Synacthen, Novartis, Funchal, Portugal) corresponding to 3.75 μg β1–24-corticotropin, immediately after the first blood sampling at their home cage. The mink were handled in alternating order, shifting between control-LS/HS and ACTH-LS/HS. Blood was sampled in late October by venepuncture of the vena cephalica at 0, 30, 60 and 120 min after capture in a wired trapping cage (Hedensted-Group). No anaesthetic was used, and all procedures were included in our permit for mink experimentation, approved by the Danish Animal Experiments Inspectorate in accordance with the Danish Ministry of Justice Law No. 382 (10 June 1987) and Acts 333 (19 May 1990) and 726 (9 September 1993) concerning animal experimentation and care of experimental animals. The delay from the first capture and the first blood sample was less than 50 s. The mink selected for this experiment were housed apart across two mink sheds in order to minimise handling-induced disturbance between individuals; after the first sampling at the home cage, they were transported to a separate room. During the 2-h blood sampling period, each mink was kept in the trapping cage, but it was only fully immobilised (by lifting the trap bottom) during each single collection of approximately 1–2 ml blood into tubes containing sodium heparin kept on ice until the separation of plasma. In addition, fresh faeces were sampled non-invasively during intervals relative to the beginning of the control/ACTH challenge: 24 h before and 0–4 (sampled in the morning before the onset of the challenge), 4–8, 8–20, 20–24 and 24–48 h after. Some mink did not deliver faeces in every interval, and some animals defecated several times in some of the intervals. We obtained an equal number of faecal samples from LS and HS mink (151 from each group). All samples (plasma and faeces) were stored at − 21°C until analysis.
Experiment 3: Excretion of infused 3H-cortisol
In Experiment 3, eight LS and eight HS mink were chosen from the original stock by the same criteria as the animals used in Experiment 2. Thus, the LS mink had no stereotypic behaviour, whereas the experimental HS mink were on average engaged in stereotypies in 41% (SD 4.6; range: 36–49%) of the scanning observations. The animals from Experiment 2 were not re-used. The 16 mink were weighed and moved from their original cage into a metabolic steel cage (dimension: 46 × 32 × 46 cm) with access to a straw-covered nest box (18 × 24 × 23 cm) 14 days prior to the onset of the sample collection in late November. The group of LS mink were heavier (LS: 1.5 ± 0.2 kg vs. HS: 1.2 ± 0.2 kg; t-test = 3.74, df = 14, P = 0.002).
In addition to natural lighting from windows, the housing facility had full light 08:00–20:00 h, dim red light 20:00–24:00 h and darkness 24:00–08:00 h.
We injected each animal on Day 0 (08:30–09:50 h) into a front leg vein with 1.0 ml (415 kBq) radioactive-labelled cortisol ([1,2,6,7-3H(N)]-Cortisol, NET-396, PerkinElmer Life and Analytical Sciences, Boston, MA, USA) dissolved in sterile 0.9% NaCl solution with 10% (v/v) ethanol. The injection area was shaved to make the procedure easier. The amount of 3H-cortisol given to each animal averaged (SD) 415 (8.7) kBq (dpm: 24.93 × 106 (2.1%); not different between LS and HS mink, t-test = 0.58, df = 14, P = 0.57), based on weighing of the syringes before/after injection.
We collected all urine and faeces delivered during the experimental period of 5 days, that is − 20 to +84 h relative to the time of injection on Day 0. The sampling intervals were Day − 1: every 4th hour between 08:00 and 24:00 h; Day 0: continuously from 08:00 to 24:00 h; and Days 1–3: every hour from 08:00 to 16:00 h, then at 20:00 and 24:00 h. The exact injection and sampling times were registered for each animal, and the urine (n = 325) and faecal samples (n = 368) were weighed to the nearest 10 mg and stored at − 21°C until analysis. The number of samplings per animal (urine: 20 (3.1); faeces: 23 (2.7)) did not differ between LS and HS mink (urine: t-test = 0.55, df = 14, P = 0.59; faeces: t-test = 0.36, df = 14, P = 0.73). The amount of urine and faeces voided (mean (SD): 168 (57.3) g urine and 127 (25.6) g faeces) did not differ between LS and HS mink (urine: t-test = 1.45, df = 14, P = 0.17; faeces: t-test = 0.55, df = 14, P = 0.59).
Analysis of samples
Plasma cortisol
Cortisol concentrations were determined, after diethyl ether extraction of 0.25 ml of plasma samples, with a cortisol enzyme immunoassay (EIA), as previously described (Palme and Möstl Citation1997; Palme et al. Citation1999). The sensitivity of this method was 0.12 ng/ml, with intra- and inter-assay coefficients of variation of 8.9 and 11.1%, respectively.
Faecal cortisol metabolites
A total of 0.5 g of each well-homogenised faecal sample was extracted with 5 ml aqueous methanol (80%) and radioactivity or FCM measured in an aliquot of the supernatant (Schatz and Palme Citation2001). All samples were analysed in duplicate. FCM were measured with an 11-oxoaetiocholanolone EIA and an 11ß-hydroxyaetiocholanolone EIA. Details of the two EIAs including cross-reactions of the antibodies are given by Möstl et al. (Citation2002) and Frigerio et al. (Citation2004), respectively. The sensitivity of these methods was 5 and 6 ng/g, with intra- and inter-assay coefficients of variations of 13.8 and 15.7, and 9.1 and 13.1%, respectively. We present only FCM values measured by the 11ß-hydroxyaetiocholanolone EIA, as both measures were highly correlated, but the values and increases were higher with this EIA in Experiment 1. Consequently, only 11ß-hydroxyaetiocholanolone EIA was used in Experiment 2.
High performance liquid chromatography separations
To characterise faecal 3H-cortisol metabolites, peak concentration samples (n = 3 for both lines) were selected and high performance liquid chromatography (HPLC) immunograms were performed. Details of the reverse-phase HPLC (RP-HPLC) separation are given elsewhere (Teskey-Gerstl et al. Citation2000; Touma et al. Citation2003). Radioactivity was measured in the fractions and immunoreactivity was determined with the 11-oxoaetiocholanolone EIA (Möstl et al. Citation2002) and the 11ß-hydroxyaetiocholanolone EIA (Frigerio et al. Citation2004).
Statistical analysis
We used the procedure ‘Mixed’ in the software SAS (version 9.2, Statistical Analysis Systems Institute, Cary, NC, USA) for calculation. A probability level (P) of 0.05 was chosen as the limit of statistical significance. Models were reduced by stepwise removing insignificant terms (P>0.10) starting with the interactions. We used Satterthwaite's approximation of the degrees of freedom (df) in the statistical tests. The demand for dispersion and variance homogeneity was evaluated from plots of the final model residuals.
For the concentrations of cortisol and FCM in Experiments 1–3, linear mixed models with repeated measures were used, testing for the difference between LS and HS mink in the intercept and the development over time. The covariance structures resulted in the best model fit were chosen based on Akaike's Information Criterion (AIC; Littell et al. Citation1996). Three types of covariance structures were used: autoregressive (ar(1) in SAS) in Experiment 1, for FCM after handling in Experiment 2, and for the urine samples in Experiment 3; autoregressive with heterogeneous variance (arh(1) in SAS) for FCM after the ACTH challenge in Experiment 2 and for the faeces samples in Experiment 3; and compound symmetry with heterogeneous variance (csh in SAS) for the concentrations of plasma cortisol in Experiment 2. The requirement of parametric statistics for dispersion and variance homogeneity was in all cases satisfactorily met after logarithmic transformation of the concentrations.
In Experiment 2, the FCM concentrations relative to handling/ACTH injection at time = 0 were analysed using five intervals: (I) 24 h before capture (n = 12); (II) 0–4 h after capture (n = 7–9); (III) 4–20 h after capture (n = 8–10); (IV) 20–24 h after capture (n = 4–7); and (V) 48 h after capture (n = 9–11). In order to make the statistical analysis possible, it was necessary to group samples collected between 4 and 20 h after capture into one interval (III). In case of more than one sample from an individual per interval, the mean value was used in the analysis.
In Experiment 3, the data for ‘recovery’, ‘faecal excretion’ and ‘time lag until maximum radioactivity’ were analysed in a linear normal model, with group (LS and HS), body weight (range 835–1695 g) and the interaction between group and body weight in the initial models. Additionally, total amount of faeces and urine voided (range 194–498 g), and its interaction with group were included in the model for the recovery; the recovery (range 62–97%), its interaction with group, and the weight of faeces sampled (range 94–189 g) were included in the model of total faecal excretion; the recovery, its interaction with group, and the number of samples (range 15–23 for faeces and 12–24 for urine) were included in the model of time lag until maximum radioactivity (analysed separately for faeces and urine). Based upon the residuals, LS and HS mink appeared to have a non-equal variance in the delay time until maximum radioactivity in faeces was reached. Therefore, the model considered variance heterogeneity between LS and HS mink (using the GROUP option in SAS; Littell et al. Citation1996).
Results
Experiment 1: FCM baseline and response to an immobilisation challenge
illustrates the daily concentrations of FCM (measured by an 11ß-hydroxyaetiocholanolone EIA) before and after an immobilisation challenge. The concentration of FCM was higher in HS than in LS mink (F1,158 = 43.9, P < 0.001), including at Day 1 (baseline; HS: 79 (10.5) vs. LS: 51 (11.5) ng/g; P = 0.002). In HS mink, the FCM concentration was higher than baseline during Days 2 and 4 after the immobilisation challenge (post hoc testing of difference between days, P < 0.048, but NS at Days 3, 9 and 10; 0.05 < P < 0.10), whereas LS mink fluctuated around baseline concentrations of FCM on Days 2–10 (NS different relative Day 1, P>0.12). Thus, the immobilisation challenge increased the difference in FCM concentrations between HS and LS mink: from being on average of 54% higher before to 75–144% higher after the immobilisation in HS relative to LS mink (). Spearman rank-order correlations between individual FCM concentrations on each of the days in Experiment 1 and individual frequencies of stereotypic behaviour revealed a significant negative correlation coefficient in line HS mink at Day 2 (rs = − 0.20, P = 0.038; n = 110). All other coefficients were low and non-significant. Carlstead and Brown (Citation2005) suggested using variation in faecal corticoid concentrations obtained over time rather than just the mean as an indicator of chronic stress (but did not apply repeated measure analysis in their study). In order to investigate whether the SD or CV differed between LS and HS mink, we pooled all FCM data collected per individual over the sampling days. To explore the potential influence on the amount of stereotypic behaviour observed, this was included in the model together with the line (LS and HS). This simplified analysis confirmed the finding of higher FCM mean values in HS than in LS mink (across all sampling days: 106.3 (7.5) vs. 54.8 (7.4) ng/g, F1,159 = 23.9, P < 0.001), with no additional explanatory value of the amount of stereotypic behaviour on mean values (F1,159 = 0.7, P = 0.41) nor on the SD (F1,159 = 2.4, P = 0.12). The relative variation, CV (SD/mean) did, however, increase with the amount of stereotypic behaviour performed (F1,159 = 10.5, P = 0.002). For the mink performing most stereotypic behaviour (at the 75% quartile or above in our data) CV averaged 85 (5.9%; n = 43), whereas the CV averaged 64 (4.29%; n = 82) for mink not performing stereotypic behaviour.
Figure 1. FCMs (ng/g) in low stereotypic (LS, n = 71–80 per day) and high stereotypic (HS, n = 69–76 per day) mink females sampled once daily at 14:00–18:00 h, given as mean ± SEM. All mink were exposed to an immobilisation challenge for 15 min at Day 2 between 09:00 and 10:00 h (indicated by the arrow). The concentration of FCM was higher in HS than in LS mink (F-test, P < 0.001), also at Day 1 (pairwise post test, P = 0.002). Significant differences from within-group baseline (Day 1) are marked with an asterisk.
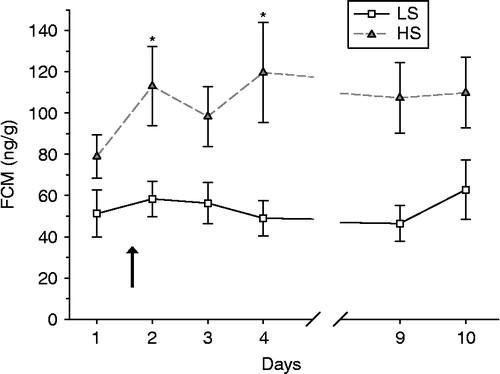
Experiment 2: Handling and ACTH challenge
At capture (time 0), there was no difference between the plasma concentration of cortisol between the LS and HS mink (F1,46 = 0.0, P = 0.91, n = 48). Plasma cortisol concentration increased significantly in response to both handling alone and in combination with ACTH injection (), without any difference between the LS and HS mink (handling: F1,21.9 = 0.4, P = 0.53; ACTH: F1,21.9 = 0.1, P = 0.82). The ACTH injection resulted in approximately 45% higher peak values at 30 min (mean (SD): 27 (2.4) ng/ml) compared to the concentration in mink exposed to the handling only (19 (1.7) ng/ml). At the last sampling (t = 120 min), LS and HS mink in both treatments had plasma cortisol concentrations approximately six times higher than baseline ().
Figure 2. Response after handling without and with ACTH injection at time = 0 given as mean ± SEM concentration of (a) plasma cortisol and (b) FCM in low (LS, n = 12) and high (HS, n = 12) stereotypic mink females. Plasma cortisol increased above baseline, with no difference between LS and HS mink (F-test, P>0.53). In FCM, HS mink reacted more than LS mink during 4–20 h (F-test, P = 0.001) after handling, but not after ACTH challenge (F-test, P = 0.74). Concentrations significantly greater than baseline are marked with asterisks.
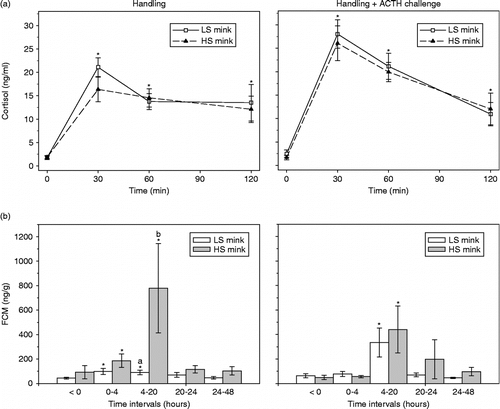
In the day before handling, the FCM did not differ significantly (F1,46 = 0.1, P = 0.71) between LS (54 (9.1) ng/g; n = 24) and HS mink (71 (27.9) ng/g; n = 24). The baseline values resembled the findings in Experiment 1 for LS and HS mink. The temporal development in FCM, sampled 24 h before and up to 48 h after capture and repeated blood sampling (), did not differ between LS and HS mink (group × interval interaction, F4.50 = 5.3, P = 0.08). HS mink reacted, however, significantly more than LS mink to the handling (FCM 4–20 h after capture, HS mink: 778 (364.7) ng/g vs. LS mink: 90 (19.3) ng/g; P = 0.001; ).
Following ACTH injection, LS and HS mink did not respond differently in FCM over time (group × interval interaction NS, F4,20.7 = 2.0, P = 0.14; ), and the mean concentration of FCM did not differ between LS and HS mink (F1,15.3 = 0.1, P = 0.74). Time since ACTH injection affected the FCM concentration (F4,13.8 = 8.2, P < 0.001), with the highest concentration of FCM in samples collected between 4 and 20 h after injection compared to the other intervals (pair-wise post hoc testing, P < 0.004).
Experiment 3: Excretion of infused 3H-cortisol
There was no significant difference between LS and HS mink in the total recovery (range 62–98% of the amount injected), the proportion of recovered radioactivity excreted in faeces (range 77–89%), the time lag until maximum concentration of radioactivity was reached in faeces (range 3.1–6.8 h) and in urine (range 1.8–4.7 h; ). The variation in time lag until maximum concentration of radioactivity in faeces was not significantly different between LS and HS mink (P = 0.066).
Table I. Total recovery of injected radioactivity, amount (%) excreted via the faeces, the time lag until peak concentrations of radioactivity in faeces and urine after intravenous injection of 3H-cortisol in low (LS; n = 8) and high (HS; n = 8) stereotyping female mink.
There was no difference between LS and HS mink in their temporal profiles of cortisol excretion into faeces (P>0.3) or urine (P>0.5; ). No significant group effect was present on the intercept (faeces: P = 0.36, urine: P = 0.85) or in any of the regression coefficients (P>0.30) tested. The final significant models of radioactivity excreted following a 3H-cortisol injection included a common intercept over both groups, and the time regression coefficients averaged over groups.
Figure 3. Time course of excretion of 3H-cortisol metabolites in (a) faeces (kBq/g) and (b) urine (kBg/ml) of low stereotypic (LS, n = 8) and high stereotypic (HS, n = 8) female mink after injection at 0 h. Data are given as hourly median with 25 and 75% quartiles for the first 16 h and per every 4th hour for the rest of the period. There was no difference between LS and HS mink for time profiles for cortisol excretion into faeces (F-test, P>0.30) or urine (F-test, P>0.50). Note the different scaling of the y-axis for faeces and urine concentration.
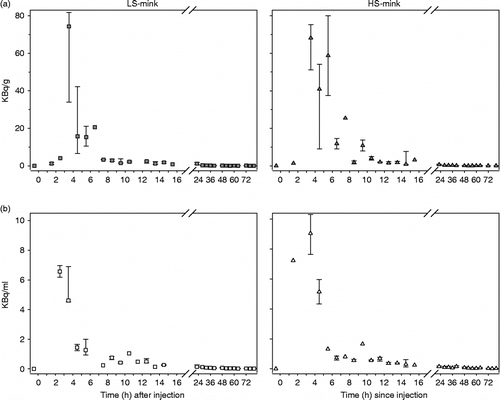
High performance liquid chromatography
After the RP-HPLC separations, there were several prominent radioactive peaks in the faecal extracts of females of both lines. All of them eluted before fraction 40 (), resembling conjugated steroids. Inter-individual differences in the proportion of the different cortisol metabolites occurred. There was nearly no native cortisol present. Both EIAs reacted not only with some of the main metabolites (), but also with a number of less polar 3H-cortisol metabolites, which were present in smaller amounts.
Figure 4. RP-HPLC radioimmunogram of peak radioactive faecal extracts from female mink. Samples shown are representative of a high stereotypic female (upper panel) and a low stereotypic female (lower panel) mink injected with 3H-cortisol. Radioactivity (solid line) and immunoreactivity with an 11-oxoaetiocholanolone EIA (- -) and an 11ß-hydroxoaetiocholanolone EIA (…) were determined in the different fractions. Elution times of respective standards are marked with open triangles (E2-diSO4, oestradiol disulphate; E1G, oestrone glucuronide; E1S, oestrone sulfphate, cortisol and corticosterone).
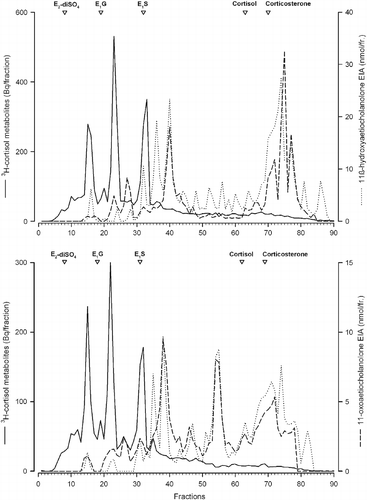
Discussion
Excretion of cortisol in mink
Faeces are the predominating excretory route of cortisol in female American mink, accounting for 83% of the recovered radioactivity after a 3H-cortisol injection. This proportion of GC excretion via the faeces is higher than in most other species (Palme et al. Citation2005), but comparable to the findings in rats (Rattus norvegicus f. dom; 75%; Lepschy et al. Citation2007) and cats (Felis silvestris f. cattus; 82–86%; Graham and Brown Citation1996; Schatz and Palme Citation2001). The carnivorous mink have a short intestinal length and a rapid transit time (between 2 and 4 h) for food (Hansen Citation1978; Bleavins and Aulerich Citation1981). The time lag from injection to peak concentration (faeces: 4 h) is shorter in mink than so far reported in any other species having faeces as an important GC excretion route (e.g. 7 h in Columbian ground squirrel, Spermophilus columbianus, Bosson et al. Citation2009; 11 h in North American red squirrel, Tamiasciurus hudsonicus, Dantzer et al. Citation2010; 15 h in laboratory rat, Lepschy et al. Citation2007; 22 h in domestic cat; Graham and Brown Citation1996; Schatz and Palme Citation2001).
In mice, time of day influenced the time lag to peak concentrations in faeces; following corticosterone injection early in the light phase, the time lag was 8–10 h and was reduced to 4–6 h following injection early in the daily dark phase. This difference in time lag was presumed to be a consequence of reduced gut-passage time induced by increased animal activity in the dark phase (Touma et al. Citation2003). In addition, baseline plasma GC concentrations peak shortly before the onset of activity, suggested as an adaptation to support increased locomotor activity (Malisch et al. Citation2008). We did not test the daily pattern of excretion in our study. HS and LS mink were injected during the morning hours, which is soon after a period of naturally high activity (around dawn and dusk in mink; Malmkvist Citation1997). We found no evidence for reduced gut-passage time or time lag in the highly active HS mink. The different levels of activity between the groups did not affect the temporal pattern of urine and faeces voided, or the already short time lag to peak concentration.
We used only females in the present study. Among other species with faeces as the predominant excretion route for CG, sex differences in faecal excretion were reported in mice (higher in males than in females, oestrus status not stated; Touma et al. Citation2003), but not documented in the cat (2m/2f in Schatz and Palme Citation2001) and the red squirrel outside the reproductive periods (4m/4f in Dantzer et al. Citation2010). Lepschy et al. (Citation2007) suggested that female rats may be more variable in their FCM excretion owing to the presence of different gonadal steroids that rapidly change over the oestrus cycle. In mink, the female oestrous cycle is controlled by the photoperiod (Hansson Citation1947; Enders Citation1952), with one yearly delivery of offspring taking place late April in the Northern hemisphere. Female FCM levels are elevated around the time of delivery (Malmkvist and Palme Citation2008). For the experimental mink, the lactation period ended definitively 8 weeks after delivery, when they were removed from their litter (around 1 July). Thus, our experiments were all performed well outside the fixed yearly reproductive cycle, at least 3 months after the weaning of kits and before the next period of oestrus. Therefore, reproductive hormone changes are not likely to influence the behaviour and adrenocortical activity in our study.
EIA for measuring FCM
FCM measured with an 11ß-hydroxyaetiocholanolone EIA reflected changes in relation to handling stress and ACTH challenge in female mink. The cortisol metabolite concentration exceeded pre-treatment levels approximately eightfold within 4–8 h after the ACTH injection. Thus our study validates a group specific EIA to measure adrenocortical activity in American mink. In addition, we used a radiometabolism experiment and RP-HPLC to characterise the FCM. Polar metabolites prevailed in mink faeces. As metabolites are excreted via the bile as conjugates, the short gut-passage time may prevent their further metabolism by bacteria. As was found in other species investigated, cortisol was heavily metabolised with nearly no native cortisol present in faeces (Palme et al. Citation2005). The 11ß-hydroxyaetiocholanolone EIA (and also the 11-oxoaetiocholanolone EIA) reacted not only with some of the main metabolites (lower degree), but also with some minor, less polar metabolites (higher cross-reactivity). Further studies to investigate sex differences and seasonal variation could be interesting. For example, periods of feed restriction increased baseline urinary cortisol level and activity (Bildsøe et al. Citation1991), and body weight loss during the winter period was correlated with increased plasma cortisol and stereotypies or running wheel activity in mink (Hansen and Damgaard Citation2009).
Stereotypic behaviour and adrenocortical activity
Svendsen et al. (Citation2007) worked with earlier generations of the breeding lines used in the present experiments and showed that mink from the HS line had higher baseline levels of FCM than mink from the LS line. This is confirmed by the present Experiment 1, which also showed that the increase in FCM after immobilisation was greater and more persistent in HS mink. The prolonged reaction to a single acute stressor in HS mink is surprising. This result may not be exclusively attributed to the immobilisation procedure. During the daily sampling of faeces, collected from grids above the manure cutter below cages, we also introduce a deviation from the daily routines at the farm. Therefore, we cannot exclude that this low intensity disturbance may contribute to the longer than expected FCM elevation after the single immobilisation challenge. The LS mink, however, showed no reaction to the procedures, indicating that the HS mink indeed are more sensitive to the effects of immobilisation stress combined with the disturbance by the daily sampling routine. Stereotypic behaviour may induce additional effects; illustrated for example by the body weight of HS mink averaging 80% of that of LS mink (Experiment 2), even though both groups were kept in the same types of cages, under the same management with a close to ad libitum feeding regime.
At the day of immobilisation in Experiment 1, we found a negative correlation between the performance of stereotypic behaviour and the concentration of FCM, when considering all mink in the HS line. This supports the hypothesis that stereotypic behaviour can reduce the adrenocortical reaction to stress (Mason and Latham Citation2004). Our study was, however, not designed to examine this particular hypothesis. In addition, we found no significant general correlations on other sampling days. The amount of stereotypic behaviour increased the relative variation in FCM (% CV), pooling all days in Experiment 1, not just attributable to their higher mean values. Thus, the HS mink were less consistent than the LS mink regarding their FCM output in our study.
Experiment 2 confirmed that HS mink have higher FCM levels than LS mink after the stressful experience of 2 h confinement in a trap combined with four blood samplings. This stressor, however, was less reflected in FCM of the LS mink than in the HS mink. By contrast, LS and HS mink showed an identical increase in FCM level in the challenge condition with an ACTH injection, indicating that adrenocortical functioning is similar. Therefore, we suggest that the reason for different FCM levels between the two lines is a lower perception or appraisal of stress in the LS than in the HS mink. Similarly, fearful mink that react with fear across a range of situations (Malmkvist and Hansen Citation2002) secreted more cortisol during handling and blood sampling stress than did confident mink (Malmkvist et al. Citation2003), whereas the two groups had an equal capacity for cortisol secretion in response to an ACTH challenge (Hansen Citation1997). The link between stereotypic behaviour and fearfulness remains to be studied in detail. One study reported, however, that stereotyping mink females were less fearful (reacted with more sniffing and less flight) than non-stereotyping females during a standardised human test (Hansen and Jeppesen Citation2006). Thus, the increased FCM response in HS mink cannot simply be explained by a higher level of fear towards humans. Other mechanisms could be involved, such as stereotypic mink characterised by, e.g. a longer duration of responding, increased activity or intensified stereotypic behaviour in response to stress.
In contrast to the FCM results, we found no difference in plasma cortisol concentration between lines in response to handling alone and in combination with the ACTH challenge. The discrepancy between plasma cortisol and FCM results cannot be explained by differences in the process of excretion of cortisol, as excretion of radio-labelled cortisol was identical in the two lines (Experiment 3). Instead, the results may reflect the different sampling methods used. The immediate, circulating concentrations of cortisol are determined in blood samples, whereas FCM represents a delayed, time-integrated measure of adrenocortical activity. We noted that, at the end of the blood sampling period, LS and HS mink in both treatments had approximately six times higher than baseline concentration of plasma cortisol. Therefore, the FCM (sampled for a longer time interval, elevated 4–20 h after capture) data could indicate that stereotypic mink take longer to return to normal circulating cortisol levels after the period of stress. We have no additional measures of plasma cortisol during the days after the stressor in the experiment, which would be useful to test this hypothesis; the results in Experiment 1 (FCM data only) support that a prolonged response may prevail in HS mink several days after a handling stressor. It should also be noted that the stressors used in Experiments 1 and 2 were not identical.
Stereotypic behaviour was concurrent with higher baseline concentrations of FCM, which cannot be explained by a higher adrenal sensitivity for cortisol secretion or a different excretion of the circulating cortisol. Instead, the HS mink reacted with more adrenocortical activity towards stressors. Similar links between stress, adrenocortical activity and the occurrence of stereotypic behaviour were suggested in studies of other caged mammals. For example, high amounts of stereotypic behaviour were correlated with elevated FCM concentrations in transgenic Alzheimer's mice (Ambrée et al. Citation2006). The propensity to perform stereotypic behaviour may also be increased in individuals sensitive to stress leading to heightened adrenocortical activity; later stereotypic behaviour was associated with elevated plasma corticosterone level after weaning in laboratory mice (Würbel and Stauffacher Citation1997). Likewise, the corticosterone increase induced by enrichment removal predicted stereotypic behaviour in male (but not in female) mice (Latham and Mason Citation2010). In most cases, the mechanisms behind the correlation between stereotypic behaviour and adrenocortical activity are not well documented. Based on the present work, however, we conclude that low stereotyping female mink have low adrenocortical activity due to a lower sensitivity to stressors at the pituitary or higher levels in the brain.
Acknowledgements
Our thanks goes to Dr Stig Purup (Aarhus University, AU) in relation to obtaining the permits for the radioactive study, Dr Birthe M. Damgaard, Betty Skov, Birthe Houbak, Mogens Olesen (AU) and Tine Simonsen (Copenhagen University) for assistance during sampling; Alexandra Kuchar, Monika Höring (University of Veterinary Medicine, UVM) for HPLC and EIAs and Helen Szöllösi (UVM) for measuring radioactivity. The experiment comply with the ‘Principles of animal care’, publication No. 86–23, revised 1985 of the National Institute of Health, and with the current Danish laws, including permit of performing animal experimentation. Permit for working with open radioactive sources within this experiment was given by the National Board of Health, Denmark.
Declaration of interest: The authors report no conflicts of interest. The authors alone are responsible for the content and writing of the paper.
References
- Ambrée O, Touma C, Görtz N, Keyvani K, Paulus W, Palme R, Sachser N. 2006. Activity changes and marked stereotypic behaviour precede Aβ pathology in TgCRND8 Alzheimer mice. Neurobiol Aging. 27:955–964.
- Bildsøe M, Heller KE, Jeppesen LL. 1991. Effects of immobility stress and food restriction on stereotypies in low and high stereotyping female ranch mink. Behav Process. 25:179–189.
- Bleavins MR, Aulerich RJ. 1981. Feed consumption and food passage time in mink (Mustela vison) and Europen ferrets (Mustela putorius furo). Lab Anim Sci. 31:268–269.
- Bosson CO, Palme R, Boonstra R. 2009. Assessment of the stress response in Columbian ground squirrels: Laboratory and field validation of an enzyme immunoassay for fecal cortisol metabolites. Physiol Biochem Zool. 82:291–301.
- Carlstead K, Brown JL. 2005. Relationships between patterns of fecal corticoid excretion and behaviour, reproduction, and environmental factors in captive black (Diceros bicornis) and white (Ceratotherium simum) rhinoceros. Zoo Biol. 24:215–232.
- Dantzer B, McAdam AG, Palme R, Fletcher QE, Boutin S, Humphries MM, Boonstra R. 2010. Fecal cortisol metabolite levels in free-ranging North American red squirrels: Assay validation and the effects of reproductive condition. Gen Comp Endocrinol. 167:279–286.
- Enders RK. 1952. Reproduction in the mink (Mustela vison). Proc Am Phil Soc. 96:691–755.
- Frigerio D, Dittami J, Möstl E, Kotrschal K. 2004. Excreted corticosterone metabolites co-vary with air temperature and air pressure in male Greylag geese (Anser anser). Gen Comp Endocrinol. 137:29–36.
- Graham LH, Brown JL. 1996. Cortisol metabolism in the domestic cat and implications for noninvasive monitoring of adrenocortical function in endangered felids. Zoo Biol. 15:71–82.
- Hansen BK, Jeppesen LL, Berg P. 2010. Stereotypic behaviour in farm mink (Neovison vison) can be reduced by selection. J Anim Breed Genet. 172:64–73.
- Hansen NE. 1978. The influence of sulphuric acid preserved herring on the passage time through the gastro-intestinal tract in mink. Ziet Tierphysiol Tierem Futtermitt. 40:285–291.
- Hansen SW. 1997. Selection for tame and fearful behaviour in mink and the effect on the HPA-axis. In: Hemsworth PH, Spinka M, Kostal L. editors. Proceeding of the 31st International Congress of the International Society of Applied Ethology. Prague: Research Institute of Animal Production72.
- Hansen SW, Damgaard BM. 2009. Running in a running wheel substitutes for stereotypies in mink (Mustela vison) but does it improve their welfare?. Appl Anim Behav Sci. 118:76–83.
- Hansen SW, Jeppesen LJ. 2006. Temperament, stereotypies and anticipatory behaviour as measures of welfare in mink. Appl Anim Behav Sci. 99:172–182.
- Hansen SW, Malmkvist J, Palme R, Damgaard BM. 2007. Do double cages and access to occupational materials improve the welfare of farmed mink?. Anim Welf. 16:63–76.
- Hansson A. 1947. The physiology of reproduction in mink (Mustela vison, Shreb.) with special reference to delayed implantation. Acta Zool Stockholm. 28:1–136.
- Jeppesen LL, Heller KE, Bildsøe M. 2004. Stereotypies in female farm mink (Mustela vison) may be genetically transmitted and associated with higher fertility due to effects on body weight. Appl Anim Behav Sci. 86:137–143.
- Latham N, Mason G. 2010. Frustration and perseveration in stereotypic captive animals: Is a taste of enrichment worse than none at all?. Behav Brain Res. 211:96–104.
- Lepschy M, Touma C, Hruby R, Palme R. 2007. Non-invasive measurement of adrenocortical activity in male and female rats. Lab Anim UK. 41:372–387.
- Littell RC, Miliken GA, Stroup WW, Wolfinger RD. 1996. SAS system for mixed models. Cary, NC: SAS Institute.
- Madej A, Forsberg M, Edquist L. 1992. Urinary-excretion of cortisol and estrone sulfate in pregnant mink fed PCB and fractions of PCB6. Ambio. 21:582–585.
- Malisch JL, Breuner CW, Gomes FR, Chappell MA, Garland TJr. 2008. Circadian pattern of total and free corticosterone concentrations, corticosteroid-binding globulin, and physical activity in mice selectively bred for high voluntary wheel-running behaviour. Gen Comp Endocrin. 156:210–217.
- Malmkvist J. 1997. Why do farm mink fur chew NJF report no. 116, Proceeding from NJF congress no 280. Helsinki: NJF211–216.
- Malmkvist J, Hansen SW. 2001. The welfare of farmed mink (Mustela vison) in relation to behavioural selection: A review. Anim Welf. 10:41–52.
- Malmkvist J, Hansen SW. 2002. Generalization of fear in farm mink, Mustela vison, genetically selected for behaviour towards humans. Anim Behav. 64:487–501.
- Malmkvist J, Hansen SW, Damgaard BM. 2003. Effect of the serotonin agonist buspirone on behaviour and hypothalamic–pituitary–adrenal axis in confident and fearful mink. Physiol Behav. 78:229–240.
- Malmkvist J, Palme R. 2008. Periparturient nest building: Implications for parturition, kit survival, maternal stress and behaviour in farmed mink (Mustela vison). Appl Anim Behav Sci. 114:270–283.
- Malmkvist J, Palme R, Hansen SW, Damgaard BM. 2004. Cortisol og corticoide nedbrydningsprodukter i minkfæcesFaglig årsberetning 2003. Holstebro: Kopenhagen Fur7–15 [In Danish, with abstract in English].
- Mason GJ. 1991. Stereotypies and suffering. Behav Proc. 25:103–115.
- Mason GJ, Cooper J, Clarebrough C. 2001. Frustrations of fur-farmed mink. Nature. 410:35–36.
- Mason GJ, Latham NR. 2004. Can't stop, won't stop: Is stereotypy a reliable animal welfare indicator?. Anim Welf. 13:S57–S69.
- Mormede P, Andanson S, Auperin B, Beerda B, Guemene D, Malmkvist J, Manteca X, Manteuffel G, Prunet P, van Reenen CG, Richard S, Vessier I. 2007. Review: Exploration of the hypothalamic–pituitary–adrenal function as a tool to evaluate animal welfare. Physiol Behav. 92:317–399.
- Möstl E, Maggs JL, Schrötter G, Besenfelder U, Palme R. 2002. Measurement of cortisol metabolites in faeces of ruminants. Vet Res Commun. 26:127–139.
- Möstl E, Palme R. 2002. Hormones as indicators of stress. Domest Anim Endocrinol. 23:67–74.
- Palme R, Fischer P, Schildorfer H, Ismail MN. 1996. Excretion of infused 14C-steroid hormones via faeces and urine in domestic livestock. Anim Reprod Sci. 43:43–63.
- Palme R, Möstl E. 1997. Measurement of cortisol metabolites in faeces of sheep as a parameter of cortisol concentration in blood. Z Saugetierkd – Int J Mammal Biol. 62 Suppl 2: 192–197.
- Palme R, Rettenbacher S, Touma C, El-Bahr SM, Möstl E. 2005. Stress hormones in mammals and birds: Comparative aspects regarding metabolism, excretion and noninvasive measurement in fecal samples. Trends Comp Endocrinol Neurobiol Ann NY Acad Sci. 1040:162–171.
- Palme R, Robia CH, Messmann S, Hofer J, Möstl E. 1999. Measurement of faecal cortisol metabolites in ruminants: A non-invasive parameter of adrenocortical function. Wien Tierärztl Mschr. 86:237–241.
- Pedersen V. 1992. Handling of silver foxes at different ages pre-weaning and post-weaning and effects on later behaviour and stress-sensitivity. Norw J Agric Sci. 9:529–535.
- Schatz S, Palme R. 2001. Measurement of faecal cortisol metabolites in cats and dogs: A non-invasive method for evaluating adrenocortical function. Vet Res Commun. 25:271–287.
- Svendsen PM, Hansen BK, Malmkvist J, Hansen SW, Palme R, Jeppesen LL. 2007. Selection against stereotypic behaviour may have contradictory consequences for the welfare of farm mink (Mustela vison). Appl Anim Behav Sci. 107:110–119.
- Teskey-Gerstl A, Bamberg E, Steineck T, Palme R. 2000. Excretion of corticosteroids in urine and faeces of hares (Lepus europaeus). J Comp Physiol B. 170:163–168.
- Touma C, Möstl E, Sachser N, Palme R. 2003. Effect of sex and time of day on metabolism and excretion of corticosterone in urine and feces of mice. Gen Comp Endocrinol. 130:267–278.
- Touma C, Palme R. 2005. Mesuring fecal glucocorticoid metabolites in mammals and birds: The importance of validation. Ann NY Acad Sci. 1046:54–74.
- Würbel H, Stauffacher M. 1997. Age and weight at weaning affect corticosterone level and development of stereotypies in ICR-mice. Anim Behav. 53:891–900.
- Zanella AJ, Mason G, Schmidt P. 1998. Selective breeding can affect the performance of abnormal behaviour and neurophysiological indicators in mink. In: Veissier I, Boissy A. editors. Proceedings of the 32nd Congress of the International Society for Applied Ethology. Clearmont-Ferrand: INRA89.