Abstract
Stress often negatively impacts physical and mental health but it has been suggested that voluntary physical activity may benefit health by reducing some of the effects of stress. The present experiments tested whether voluntary exercise can reduce heart rate, core body temperature and locomotor activity responses to acute (novelty or loud noise) or repeated stress (loud noise). After 6 weeks of running-wheel access, rats exposed to a novel environment had reduced heart rate, core body temperature, and locomotor activity responses compared to rats housed under sedentary conditions. In contrast, none of these measures were different between exercised and sedentary rats following acute 30-min noise exposures, at either 85 or 98 dB. Following 10 weeks of running-wheel access, both groups displayed significant habituation of all these responses to 10 consecutive daily 30-min presentations of 98 dB noise stress. However, the extent of habituation of all three responses was significantly enhanced in exercised compared to sedentary animals on the last exposure to noise. These results suggest that in physically active animals, under some conditions, acute responses to stress exposure may be reduced, and response habituation to repeated stress may be enhanced, which ultimately may reduce the negative and cumulative impact of stress.
Introduction
Stress has been associated with the onset or exacerbation of a wide array of physical and psychological disturbances (Hammen et al. Citation1992; Sapolsky Citation1996; Chrousos and Gold Citation1998; Vanitallie Citation2002; McEwen Citation2004). Conversely, voluntary physical activity is associated with prevention or improvement in many of the same physical and psychological disorders linked to stress (Melzer et al. Citation2004; Oguma and Shinoda-Tagawa Citation2004; Forcier et al. Citation2006; Jedrziewski et al. Citation2007; Hillman et al. Citation2008). This has led several investigators to suggest that the benefits of exercise may, at least in part, provide protection from, or resistance to, some of the damaging effects of stress (Rosch Citation1985; Moraska and Fleshner Citation2001; Salmon Citation2001). Studies have typically not shown a consistent effect of voluntary physical exercise on glucocorticoid responses to acute stressors in humans (Sinyor et al. Citation1983; Sothmann et al. Citation1988; Blaney et al. Citation1990) or animals (Cox et al. Citation1985; Dishman et al. Citation1995, Citation1998; Fleshner Citation2000; Chennaoui et al. Citation2002; Droste et al. Citation2003; Lancel et al. Citation2003). However, more recent studies suggest that the type or intensity of the stressor may be important in determining the effects of physical activity on acute glucocorticoid release, with physically active animals exhibiting similar or greater glucocorticoid responses to higher intensity stressors, such as forced swimming, restraint or loud noise (98 dB), but diminished glucocorticoid responses to low intensity stressors such as exposure to a novel environment or lower intensity noise (85 dB) (Droste et al. Citation2007; Campeau et al. Citation2010). This regulation may be limited to the periphery or specific brain regions, as no statistically significant changes in free glucocorticoid levels were observed in the hippocampus following 4 weeks of voluntary activity, the only region examined (Droste et al. Citation2009).
With respect to other responses to acute stressors, most human studies have reported very moderate, if any, differences between more sedentary compared to more active individuals in acute stress-induced arterial catecholamine concentrations (Cleroux et al. Citation1985; Sothmann et al. Citation1987; Claytor et al. Citation1988; Sinyor et al. Citation1988; Sothmann et al. Citation1991, Citation1992). Animal studies have generally mirrored the above human outcomes with respect to overall peripheral catecholamine release in response to acute stress situations (Cox et al. Citation1985; Overton et al. Citation1991; Morimoto et al. Citation2000). However, there is evidence in rats that a history of voluntary physical activity results in a differential regulation of catecholamine content in various peripheral compartments (Fleshner Citation2000; Greenwood et al. Citation2003b), increased expression of phenylethanolamine N-methyltransferase expression in the left atrium of the heart (Bakos et al. Citation2008), and reduced central sympathetic drive (Greenwood et al. Citation2003b). In addition, there are limited data of rats to suggest that a history of voluntary activity can reduce cardiovascular responses to acute stressor exposure (Morimoto et al. Citation2000), an effect that has also been reported in humans. Despite a lack of difference in circulating catecholamine concentrations, there are some indications that the more active individuals show a smaller increase in blood pressure in response to an acute stressor (Cleroux et al. Citation1985) and faster heart rate recovery (Sinyor et al. Citation1983). Combined, these data indicate that there are quite complex effects of prior exercise on acute stress responses.
While understanding the effect of exercise on responses to acute stressor exposure is important, it is considered that repeated or chronic stressor exposure is particularly detrimental to health (Sapolsky Citation1996; Ising et al. Citation1999; McEwen Citation2000, Citation2004; Vanitallie Citation2002; Swaab et al. Citation2005; Calcagni and Elenkov Citation2006; Sinski et al. Citation2006). Relatively little is known about the effect of exercise on responses following repeated stressor exposure. However, we have previously shown that male rats that had access to a running wheel for 6 weeks before repeated exposure to loud noise (98 dB), displayed faster rates of, or more extensive habituation of plasma corticosterone responses, compared to sedentary rats (Sasse et al. Citation2008a). To our knowledge, the effect of exercise on other responses to repeated stressor exposure has not been studied.
The present study was designed to: (1) test the hypothesis that prior voluntary wheel running in rats diminishes autonomic and locomotor activity responses to acute low-intensity stressors (Droste et al. Citation2007; Campeau et al. Citation2010), as compared to either no change (Campeau et al. Citation2010) or higher responses (Droste et al. Citation2007) to higher intensity stressors, as reported previously for hypothalamic pituitary adrenal (HPA) axis responses; (2) test whether habituation of these responses to repeated stressor exposure would be enhanced, as reported previously for HPA axis responses (Sasse et al. Citation2008a). Heart rate, core body temperature, and locomotor activity responses were measured by radiotelemetry during acute exposure to a novel environment or white noise at low or moderate to high intensity (85 or 98 dB). These responses are known to adapt with repeated stressor exposures (Konarska et al. Citation1989, Citation1990; Dobrakovova et al. Citation1993; Campeau et al. Citation2002; Grissom et al. Citation2008; Masini et al. Citation2008), and the effect of prior voluntary wheel-running on habituation of these responses to 10 consecutive daily presentations of 98 dB noise was assessed. The results suggest that physical activity can reduce multiple responses to some, but not all, acute stressors, and in contrast to glucocorticoid release, this appears to depend on the type, but not the intensity, of the stressor. In addition, the extent of response habituation to 98 dB noise exposure was greater in exercised rats, suggesting that prior exercise may enhance adaptation across multiple stressor-induced responses.
Materials and methods
Male Sprague–Dawley rats (n = 16) weighing 175–200 g and approximately 2 months of age were acquired from Harlan Laboratories (Indianapolis, IN, USA). They were housed in a dedicated colony facility and initially housed in groups of 4–5 per clear polycarbonate cage (48 × 27 × 20 cm) containing wood shavings, and covered with wire lids providing food (rat chow) and water ad libitum. Rats were group housed for at least 7 days after arrival from the supplier, before implantation of the radiotelemetry devices (see below). They were kept on a controlled light–dark cycle (lights on 07:00 h– off at 19:00 h), under controlled humidity (30–40%), and temperature (20–22°C) conditions. All procedures were performed between 08:00 and 16:00 h, which provided the time required to allow heart rate and core body temperatures to reach basal values following transport to the experimental room or to test each of the two consecutive squads of eight rats given our limited number (8) of ER-4000 Energizer receivers (see description below). All procedures were reviewed and approved by the Institutional Animal Care and Use Committee of the University of Colorado and conformed to the United States of America National Institutes of Health Guide for the Care and Use of Laboratory Animals.
Surgery
Radiotelemetry ‘E-mitter’ transponders (Philips Respironics, Bend, OR, USA) were surgically implanted under halothane anesthesia (1–2.5% [vol/vol] in pure oxygen) and aseptic conditions. Briefly, abdominal and thoracic areas of the rats were shaved, disinfected, and a 1.5-cm midline abdominal incision was made to insert an E-mitter Transponder (E4000) in the abdominal cavity (Masini et al. Citation2008). A 1-cm incision was made over the right clavicle, through which the negative lead of the E-mitter was threaded subcutaneously (10 G trocar), and stitched to the muscle layer using stainless steel thread. A similar incision was made on the left side, just anterior to the diaphragm, where the positive E-mitter lead was stitched to muscle layer. This 45–60° leads angle in the transverse plane of the heart is required to measure heart rate accurately, which was tested before closing the incisions. The abdominal muscle was then closed with absorbable (3–0) thread, and the skin incisions closed with silk thread (3–0). Upon recovery from anesthesia and for the remainder of the study, rats were singly housed in smaller clear polycarbonate cages (43 × 22 × 20 cm).
Voluntary exercise
After 7 days recovery from surgery, rats were divided into two groups (n = 8 per group). Those assigned to the Exercise (Ex) condition were given unlimited access to a stainless steel running wheel (34.5 cm wheel diameter × 9.7 cm width, Thermo Fisher Scientific, Rochester, NY, USA) attached to the lid of their cages for the duration of the experiment. Running distances were continuously monitored by computer using the VitalView Automated Data-Acquisition System (Philips Respironics). Sedentary (Sed) control rats were individually housed in the same colony room in cages of similar size without running wheels. Rats were housed under these conditions for 6 weeks prior to any stress testing. All rats were weighed immediately prior to the beginning of individual housing to match the groups based on body weights (mean ± SEM, Sed = 256.2 ± 4.1 g; Ex = 258.2 ± 4.6 g; n, 8/group). A body weight difference of approximately 20 g (heavier in the sedentary group) emerged relatively quickly (within 1–2 weeks) following wheel access and was maintained for the duration of the study (data not shown), as reported previously (Sasse et al. Citation2008a).
Measurement of heart rate, body temperature, and activity
Locomotor activity, core body temperature and heart rate responses were measured simultaneously by placement of the rat's home cage (or novel environment) over the ER-4000 Energizer receiver (Philips Respironics). The energizers/receivers captured these responses from the E-mitter Transponder, without additional manipulations. Total activity counts, heart rate (beats per minute), and core body temperature (°C) responses were sampled at 1 min intervals during experimental sessions. The associated VitalView Data Acquisition Software (version 4.1) digitized and saved data for offline analyses. The measures analyzed were changes (δ) in heart rate, body temperature, and total activity against baseline values computed immediately prior to stressor exposure (see below). Changes were computed for each rat by subtracting the baseline of each measure for each rat from the value for each one-min sample during/after the stressor exposure. For the acute stressor exposures, the average of the 60-min period immediately prior to novel environment or 85 dB noise exposure was used as a baseline measure. The baseline for repeated 98 dB noise exposure was calculated by averaging the values obtained during the 10-min period immediately prior to loud noise exposure. Total behavioral activity counts reflected all horizontal and vertical movements, as well as vigorous grooming. Heart rate, temperature, and activity changes were examined in 5-min blocks of time by averaging the 5 one-min samples for each subject.
Experiment 1: Acute novel environment stress
This experiment was performed after rats had been housed under Ex or Sed conditions for 6 weeks. The experiment was performed over 2 days, with 4 rats from each group tested on each day, as graphically depicted in . The afternoon prior to the experiment, each rat was placed, within its home cage, on a sensor pad within the colony room. The following day, approximately 3 h after lights on, individual rats were transferred to a novel environment, consisting of an empty circular white plastic household bucket (base diameter 25 cm, height 36 cm). The bucket was immediately placed on the sensor pad within the colony room. Heart rate, core body temperature, and locomotor activity were monitored for 3 h while the rats remained in the buckets. Rats were then returned to their home cage. The amplitude of the HPA axis responses obtained in this novel environment, in our laboratory, is approximately half of that obtained for responses to 95–105 dB white noise (Campeau et al. Citation2010).
Experiment 2: Acute noise stress, 85 dB
After the acute novel environment test, rats were familiarized daily for 10 days to transport to a separate testing room further along the corridor, and placement in the acoustic isolation chambers for approximately 1 h, as depicted in . Before transport, all rats were individually transferred to a different plastic ‘shoebox’ cage, with the same dimensions as the home cages, in order to prevent the Ex group from having access to the running wheel during noise exposure on the test day. The rats were returned to their home cages, with access to a running wheel as appropriate, upon return to the colony room. The same cage and isolation chamber were used for each rat on each day of familiarization and on the test day. Each acoustic chamber consisted of a ventilated, double wooden (2.5 cm plywood board) enclosure, with the outer enclosure lined internally with 2.5 cm insulation (Celotex™). The internal dimensions of the inner enclosure were 59.7 cm (w) × 38.1 cm (d) × 38.1 cm (h), which allowed placement of a polycarbonate rat home cage. Each inner enclosure was fitted with a single 15 × 23 cm Optimus speaker (#12-769–120W RMS) fixed in the middle of the ceiling. Lighting was provided by a fluorescent lamp (15 W) covered with red tissue paper located in the upper left corner of the chamber. Noise was produced by a General Radio (#1381) solid-state random-noise generator with the bandwidth set at 2–50 kHz. The output of the noise generator was fed to power amplifiers (Pyramid Studio Pro #PA-600X), the outputs of which were fed to the speakers. Noise intensity was measured each morning prior to noise presentations by placing a Radio Shack Realistic Sound Level Meter (A scale; #33-2050) in each acoustic chamber at several locations and taking an average of the different readings. The insulated double-enclosure design of the acoustic chambers was sufficient to prevent any measurable noise transfer between chambers. On the test day, 1–2 h after lights on, rats were transported to the testing room as previously experienced during the familiarization phase. Rats were placed individually, within their cages, on top of a sensor pad within the acoustic isolation chambers. After a 4-h equilibration period, rats were exposed to 85 dB white noise for 30 min. After the end of noise exposure, heart rate, body temperature, and locomotor activity were monitored for a further 2.5 h. An intensity of 85 dB was chosen as a second low-intensity stressor. This noise intensity was the lowest that leads to a significant increase in ACTH and corticosterone levels, and produced a sub-maximal hormonal response (Burow et al. Citation2005). In addition, we have shown that 6 weeks of prior wheel running reduces the HPA axis response to 85 dB noise (Campeau et al. Citation2010). This experiment was performed over 2 days, with four rats in each group tested on each day.
Experiment 3: Repeated audiogenic stress, 98 dB
Approximately 2 weeks after the acute noise stress test, and after 10 weeks under Ex or Sed conditions, the effect of repeated noise exposure on heart rate, core body temperature, and locomotor activity was assessed. Moderate levels of continuous loud noise have been shown to produce nearly complete habituation of corticosterone responses and behavioral activity within 10–15 consecutive daily 30 min exposures in male Sprague–Dawley rats (Campeau et al. Citation2002).
For 3 days prior to day 1 of testing, all rats were re-familiarized to transport to the testing room and placed into their respective acoustic chambers for 30 min where they were exposed only to the background noise (60 dB) produced by the ventilation fans. For this experiment, rats remained in their home cages during the re-familiarization and testing, but the wheel was removed from the Ex group, and replaced with a wire lid, so that the test cages were equivalent for both Ex and Sed groups. The wheel was replaced into the home cages once the animals were returned to the colony after testing.
Then, for 10 consecutive days, rats were transported to the testing room as previously experienced during the pre-familiarization phase, as shown in . As for the acute noise exposure, rats were placed individually, within their cages, on top of a sensor pad within the acoustic isolation chambers. After a 2-h equilibration period, rats were exposed to 98 dB white noise for 30 min. After the end of noise exposure, heart rate, body temperature, and locomotor activity were monitored for a further 60-min. 98 dB loud noise was chosen because it is an intermediate intensity, which is strong enough to allow habituation of heart rate and body temperature responses to be observed, but not so strong as to prevent habituation of these responses (Masini et al. Citation2008). Additionally, we have previously observed that 6 weeks of prior wheel running results in an enhancement of glucocorticoid response habituation to 98 dB noise (Sasse et al. Citation2008a). Responses were recorded daily throughout the entire session (pre-, during, and post-noise exposures). Upon termination of each session, all rats were immediately returned to the main housing facility. All 16 rats were tested on the same 10 days, but were tested in two cohorts, with four animals from each group in each cohort. The first cohort was transported to the testing room 1–2 h after lights on, whereas the second cohort was transported to the testing room approximately 5 h after lights on, with the different cohorts alternated daily, so that time of day could not be a significant confound. The post-stress response monitoring times were reduced from 2.5 h (Exps 1 and 2) to 60 min to accommodate the testing of the two animal cohorts daily.
Data analysis
A repeated measures analysis of variance (ANOVA) on averaged daily running distances computed for each week of the study was carried out in the free-wheel running rats to assess running behavior over time. For all experiments, individual rats' heart rate, body temperature, and active behaviors were averaged in 5-min blocks for statistical analyses, timed with stressor exposures (or similar time for the control rats), with the first averaged block during stressor (or control) exposure (0–5 min) being defined as time 5 min in all figures. Heart rate, body temperature, and behavioral changes (δ) were computed by subtracting baseline values (see below) from each averaged 5-min time blocks for each rat for the acute novelty (Exp. 1), 85 dB noise exposures (Exp. 2), and for the 1st, 5th, and 10th repeated 98 dB noise exposures (Exp. 3). For Exp. 1, repeated measures ANOVAs included 24 time blocks (2 h) during novel environment exposures, and Group (Ex or Sed). For Exp. 2, similar repeated measures ANOVAs were conducted on the six time blocks (30 min) during the acute 85 dB noise or control exposures (with Group as a between subjects factor). For Exp. 3, repeated measures ANOVAs with exposure day (1st, 5th, and 10th), Time (12 blocks of 5 min–1 h during 98 dB or control exposure and recovery) as within-subjects factors, and Group (Ex and Sed) as a between-subjects factor were used to analyze temperature, heart rate, and behavioral activity changes (δ). Additional ANOVAs were performed to assess potential differences in baseline heart rate, body temperature, and behavioral activity from the averages of the 10 min period immediately before novelty, noise, or control exposures. Significant interaction effects on the repeated measures ANOVAs in Exp. 3 were followed by additional repeated measures ANOVAs on each day (time as a within-subjects factor, and Group as a between-subjects factor) to identify more specifically the significant differences between groups. When specific differences were detected for a given daily measure (all Exps), specific time points (associated with peak response times) were tested with appropriate Bonferroni correction to statistically assess group differences. It should be emphasized that all statistical tests were performed on change values (δ), but all the figures are presented as actual values to allow simultaneous visualization of baseline, stress responses, and recovery values for each measure. Additional areas under the curve values from individual deltas during stressor exposure in Exp. 1 (2 h), stressor exposure and 30 min recovery for Exp. 2 (1 h total), and stressor exposure and 30 min recovery for Exp. 3 (1 h total), were computed and evaluated statistically with one-way ANOVAs, with Group as a between-subjects factor in Exps 1 and 2, and repeated measures ANOVAs with days (within-subjects variable) and group (between-subjects factor) for Exp. 3. Statistically significant differences were set at P ≤ 0.05.
Results
As routinely reported (Fediuc et al. Citation2006; Greenwood et al. Citation2009), rats given access to running wheels increased their average daily running distances over the first 4 weeks of access, which declined slowly thereafter, as shown in . This result was statistically revealed by a significant repeated measures ANOVA on the running distances of the free-wheel running rats (F(11,77) = 3.09, p = 0.002), with a significant quadratic component (F(1,7) = 5.70, p = 0.048), indicating the rise and fall of running distances over time (no other contrasts significant).
Figure 2. Mean distance run per 24 h ( ± SEM) calculated weekly over the 12 weeks of the study in rats (n = 8) given voluntary access to running wheels. As is typically observed in Sprague–Dawley rats, the daily average distance run increased initially until the fourth/fifth week of running, and gradually decreased thereafter. Repeated measures ANOVA on mean running distances was significant (p = 0.002), as was the quadratic component (p = 0.048).
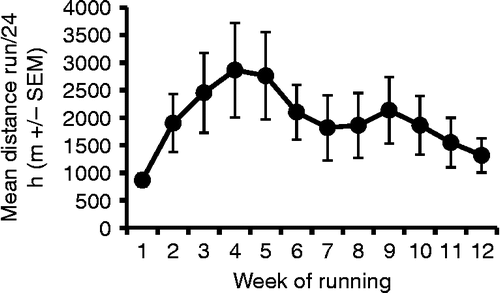
Experiment 1: Acute novel environment stress
One rat in each group had unreliable readings from the E-mitter, and were excluded from the analysis. There were no significant differences in baseline measures between Ex and Sed rats for heart rate (F(1,12) = 0.02, p = 0.90), body temperature (F(1,12) = 0.52, p = 0.49) or activity (F(1,12) = 0.17, p = 0.67). Exposure to the novel environment resulted in a rapid increase in all three measures, peaking at 5–10 min for activity and heart rate, and at approximately 30 min for body temperature (). Repeated measures analysis of the first 2 h after introduction to the novel environment revealed that Sed rats had a significantly greater increase in heart rate (time × group interaction, F(23,276) = 4.17, p < 0.001, and group effect, F(1,12) = 7.08, p = 0.021), core body temperature (time × group interaction, F(23,276) = 2.78, p < 0.001, and group effect, F(1,12) = 5.50, p = 0.037), and activity (time × group interaction, F(23,276) = 1.99, p = 0.005, and group effect, F(1,12) = 11.92, p = 0.005) when compared with Ex rats. Additional post-hoc analyses of mean delta heart rate by t-tests in the 10–55 min time blocks indicated significantly higher values from the 15- to 55-min blocks (all p < 0.005, Bonferroni correction) in the Sed group. Similar post-hoc analyses of mean body temperature deltas from the 15–60 min time blocks indicated significantly higher values from the 25- to 60-min blocks (all p < 0.005, Bonferroni correction) in the Sed group. Post-hoc analyses in the 5–50 min time blocks indicated significantly higher locomotor activity in the Sed group between 5–15 and 25–50 min blocks (all p < 0.005, Bonferroni correction). As shows, the area under the curve deltas for the heart rate (F(1,12) = 7.08, p = 0.021), core body temperature (F(1,12) = 5.50, p = 0.037), and activity (F(1,12) = 11.92, p = 0.005) were all significantly different between the Ex and Sed rats.
Figure 3. Mean ( ± SEM) heart rate (Panel A), body temperature (Panel B), and behavioral activity counts (Panel C) during novel environment (white bucket–black bars on x-axis) exposure in sedentary (black squares) and free-wheel running (open circles) rats (n = 7/group). The solid bar represents the time that the rats were exposed to the novel environment. Significant statistical differences (repeated measures ANOVA) were obtained between exercised and sedentary rats on all three response measures in response to the novel environment (p < 0.05). BPM: beats per minute; Temp.: temperature. Asterisks indicate that the groups were significantly different at the time points spanning the thin horizontal bars (t-tests, p < 0.005, Bonferroni correction).

Table I. Areas under the curve (mean ± SEM) for the heart rate, core body temperature (Temp.), and locomotor activity during stress presentation and recovery from stress for Experiments (Exps) 1, 2, and 3. Exp. 1: acute novel environment stress; Exp. 2: acute noise stress, 85 dB; and Exp. 3: repeated audiogenic stress, 98 dB.
Experiment 2: Acute audiogenic stress, 85 dB
One rat in each group had unreliable readings from the E-mitter, and were excluded from the analysis. As observed in Exp. 1, there were no significant differences in baseline measures between Ex and Sed rats for heart rate (F(1,12) = 0.33, p = 0.58), body temperature (F(1,13) = 0.11, p = 0.75) or activity (F(1,13) = 0.20, p = 0.66). A 30-min exposure to 85 dB white noise resulted in a rapid increase in all three measures, peaking at 5–10 min for activity and heart rate, and at approximately 30 min for body temperature (). In contrast to the effect of an acute novel environment exposure, there were no significant differences between Ex and Sed rats for any of these responses (heart rate–time × group interaction, F(5,60) = 1.15, p = 0.35, and group effect, F(1,12) = 2.70, p = 0.13; core body temperature–time × group interaction, F(5,60) = 0.48, p = 0.79, and group effect, F(1,12) = 0.016, p = 0.90; and activity–time × group interaction, F(5,60) = 1.36, p = 0.25, and group effect, F(1,12) = 0.33, p = 0.58). also shows the area under the curve deltas for Exp. 2. ANOVAs indicated no effects of exercise for the heart rate (F(1,12) = 0.48, p = 0.50), core body temperature (F(1,12) = 0.16, p = 0.70), and activity (F(1,12) = 0.07, p = 0.80) measures.
Figure 4. Mean ( ± SEM) heart rate (Panel A), body temperature (Panel B), and behavioral activity counts (Panel C) during acute loud noise exposure (85 dB – black bars on x-axis) in sedentary (black squares) and free-wheel running (open circles) rats (n = 7/group). The solid black bar represents the time that the rats were exposed to noise. BPM: beats per minute; Temp.: temperature. No statistically significant differences were obtained between exercised and sedentary rats on any of the responses induced by a 30-min 85 dB noise session (repeated measures ANOVAs, all p′s>0.05).
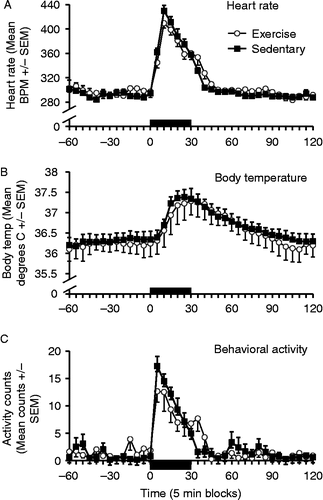
Experiment 3: Repeated audiogenic stress, 98 dB
Two rats in each group had unreliable readings for heart rate or body temperature (partial or total E-mitter failures) by this time, so these rats were excluded from the data analyses and figures. Based on six rats per group, demonstrates greatest increases in heart rate, body temperature, and locomotor activity on the first noise exposure, which became smaller (habituated) over repeated exposures (days 5 and 10). Although Ex and Sed rats displayed similar responses upon the first loud noise exposure, Ex rats showed a more complete habituation by the 10th loud noise exposure, compared to Sed rats. Repeated measures ANOVA on heart rate deltas indicated significant day × time (F(22,220) = 13.44, p < 0.001), and time × group (F(11,110) = 2.18, p = 0.02) interactions in addition to the simple effects (not shown), suggesting habituation of the heart rate deltas over repeated exposures. The overall time × group interaction strongly suggested that habituation varied depending on the exercise status of the rats. Additional repeated measures ANOVAs of heart rate deltas on each of the days analyzed only reported a significant time × group interaction on day 10 (F(11,121) = 3.20, p = 0.001), indicating higher heart rate in Sed rats. The heart rate deltas were significantly different around the peak heart rate response (5- and 10-min time blocks, (F(1,12) = 7.79 and 12.85, all p < 0.01, Bonferroni correction)). Similar results were obtained for core body temperature deltas, with significant day × time (F(22,220) = 7.42, p < 0.001), and time × group (F(11,110) = 2.46, p = 0.009) interactions. Repeated measures ANOVAs on each day reported again a significant time × group interaction only on day 10 (F(11,121) = 3.49, p < 0.001). ANOVAs around the peak temperature responses (25–30 min time blocks) revealed a significantly greater hyperthermic response in the Sed compared to the Ex rats in the 25-min block (F(1,12) = 7.30, p = 0.02, Bonferroni correction). Total locomotor activity deltas also displayed significant day × time (F(22,220) = 5.26, p < 0.001), and time × group (F(11,121) = 2.93, p = 0.002) interactions. This interaction can be explained by a significant difference between the Sed and Ex rats within the first 5 min time block of noise exposure, the peak period of the locomotor response, on day 10 (F(1,12) = 10.83, p < 0.01, Bonferroni correction). These differences in heart rate, core body temperature, and locomotor habituation between Sed and Ex rats on the last noise exposure could not be explained by differential basal values for any of these responses on any day (all p values >0.05 for each 10-min period immediately prior to loud noise exposures). However, repeated measures analyses of areas under the curves for the three measures did not return any significant interaction or group effects (heart rate day × group interaction F(2,20) = 0.008, p = 0.93, or group effect (F(1,10) = 0.51, p = 0.491), temperature day × group interaction (F(2,20) = 0.485, p = 0.623), or group effect (F(1,10) = 1.36, p = 0.271), and activity day × group interaction (F(2,20) = 0.20, p = 0.98), or group effect (F(1,10) = 0.001, p = 0.97).
Figure 5. Mean ( ± SEM) heart rate (Panel A – top row), body temperature (Panel B – middle row), and behavioral activity counts (Panel C – bottom row) during the first (day 1 – left column), fifth (day 5 – middle column), and 10th (day 10 – right column) loud noise (30 min, 98 dB – black bars on x-axis) exposures in sedentary (black squares) and voluntary exercise (open circles) rats (n = 6/group). The solid bar represents the time that the rats were exposed to noise. BPM: beats per minute; Temp.: temperature. Statistically significant differences (repeated measures ANOVA) were obtained between exercised and sedentary rats on all three response measures on the 10th loud noise exposure (all p < 0.05). Asterisks indicate significant difference between sedentary and exercised groups (t-tests, p < 0.02, Bonferroni correction), at the indicated time points.
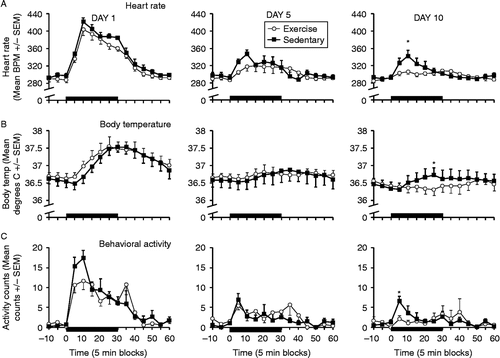
Discussion
The results of this study support the hypothesis that physical activity can reduce responses to an acute stressor under some conditions, with heart rate, body temperature, and locomotor activity responses to a novel environment reduced in rats with 6 weeks of prior access to a running wheel, compared to those housed under sedentary conditions. However, prior exercise did not reduce these acute responses under all stressor conditions, with heart rate, body temperature, and locomotor activity responses to either 85 or 98 dB noise exposure similar between exercised and sedentary rats, indicating that the type of stressor may be an important factor in determining whether these acute responses are modified by prior exercise. In addition, 10 weeks of prior access to a running wheel enhanced the extent or final level of heart rate, body temperature, and activity response habituation to repeated loud noise (98 dB) exposures, consistent with the idea that exercise can provide some resilience to the effects of repeated stressor exposure. The observed differences between the sedentary and free-wheel running rats in either the acute response to novelty or response habituation to repeated loud noise were not due to differential basal levels of any of the responses measured across groups or days, suggesting that it is the reactivity to stress exposure that may be specifically modulated by exercise under certain conditions. The regulation of the measured responses in the current study are likely a reflection of centrally mediated and coordinated autonomic and behavioral responses triggered by exposure to challenging and potentially threatening situations, elevating autonomic tone, and behavioral preparedness, and allowing animals to respond to uncertain, novel situations, more effectively.
It has been reported that cardiovascular (heart rate and blood pressure) responses to either a cage switch or restraint stressor exposure were smaller in rats that had free wheel access for the previous 10 weeks, compared with sedentary rats (Morimoto et al. Citation2000). However, as described in the introduction here, a prior history of physical activity has not been reported to universally reduce all responses to all acute stressor exposures. Our data are in general agreement with these prior observations, with free-wheel running rats exhibiting smaller heart rate, body temperature, and activity responses to an acute novel environment exposure, but not acute noise exposures, at either 85 or 98 dB, compared with sedentary rats. This is in contrast to the effect of prior physical activity on HPA axis indices, with ACTH and corticosterone responses to both novelty and 85 dB noise reduced by 6 weeks of prior voluntary wheel running (Campeau et al. Citation2010). These and other observations of HPA axis responses have led us to hypothesize that responses to relatively low-intensity stressors, as previously measured by stressor-induced levels of ACTH and corticosterone, are modulated by prior voluntary physical activity, whereas the response to higher intensity stressors (such as 98 dB noise or restraint) is similar or greater (Droste et al. Citation2007; Sasse et al. Citation2008a, Citation2008b; Campeau et al. Citation2010). It appears that this hypothesis cannot be extended to heart rate, body temperature, and locomotor activity responses measured in the present study, because the responses to a relatively weak-intensity stressor (85 dB noise, as previously characterized by ACTH and corticosterone release) were not modified by prior voluntary physical activity. As discussed above, whereas the HPA axis responses to a novel environment and 85 dB noise are lower than that to 98 dB noise (Campeau et al. Citation2010), heart rate, body temperature, and locomotor responses were quite similar in amplitude to all three acute stressors in the current study. These data suggest that stressor intensity may be less relevant for these measures, and differences between exercised and sedentary rats for these responses may depend more on the qualitative differences between stressor modality. Alternatively, the intensity range or specific stimulus characteristics of the stressor situations employed might have been too narrow to consistently elicit differential responding in the physically active as compared to the more sedentary animals. One note of caution to the above interpretation arises from the fact that the same animals experienced all three stress situations in the current study, and possible interactions with prior stress experience may have reduced putative exercise effects on autonomic and behavioral measures in response to stressor exposure in the second and third experiments. This possibility could explain the different results previously obtained with HPA axis activity to an acute 85 dB loud noise because these rats only experienced a single stress episode (Campeau et al. Citation2010). Future studies will be required to test this possibility.
In addition to the effects of voluntary wheel running on the acute responses to stressor exposure, we have previously reported that habituation of the corticosterone response to repeated loud noise exposures was enhanced in exercised compared with sedentary rats (Sasse et al. Citation2008a). Similarly, differences in plasma ACTH concentrations were observed after the second day of a footshock paradigm in exercised compared to sedentary rats (Dishman et al. Citation1998). The present data extend these findings, and show that the extent of habituation of heart rate, body temperature, and locomotor activity responses to repeated loud noise (98 dB) exposure are enhanced in exercised rats, consistent with the idea that multiple responses to repeated stressor exposure can be modulated by prior physical activity. In the current paradigm, noise presentation occurred on a daily basis. However, there is a difference between short- vs. long-term habituation to the repeated stressor exposure. That is, despite similar levels of HPA axis, body temperature, and the heart rate response habituation obtained following the presentation of the last repeated stressor in relatively rapid succession (30 min), or with longer interstressor intervals (24 h), only the longer stressor interval produced significant long-term (48 h) habituation of these responses (Masini et al. Citation2008). It would be of interest to determine if short- vs. long-term habituation, which likely involve quite different mechanisms, are differentially regulated in physically active compared to sedentary rats. In addition, it seems that not all the responses elicited by acute stressors, and their habituation to repeated exposures follow the same rate and reach the same extent, but appear to be highly variable. In the current study, within-subjects heart rate, core body temperature, and locomotor activity were all significantly reduced by the fifth loud noise exposure. Similar co-variations of multiple within-subjects stress-elicited responses to repeated homotypic challenges have also been reported (De Boer et al. Citation1988; Dobrakovova et al. Citation1993; Grissom et al. Citation2008; Masini et al. Citation2008). However, other studies have reported significant dissociations in the rate or extent of habituation between different responses (Chen and Herbert Citation1995; Pitman et al. Citation1995; Stamp and Herbert Citation1999; Carter et al. Citation2004; Martin et al. Citation2004; Bhatnagar et al. Citation2006). As with the variable effects of voluntary exercise on different stressor types, the reasons for the differential patterns of results on habituation of multiple within-subjects stress-elicited responses are not easily explained.
The specific mechanisms whereby prior physical activity modifies these and other responses to stressor exposure are not known. However, multiple instances of modulation of cognitive functions, including learned helplessness (Moraska and Fleshner Citation2001; Greenwood et al. Citation2003a), fear conditioning (Greenwood et al. Citation2009), and other learning paradigms (Fordyce and Wehner Citation1993; Radak et al. Citation2001, Moncek et al. Citation2004; Kramer et al. Citation2006), argue in favor of direct central nervous system influences by physical activity. Furthermore, as exemplified by the enhancement of stress habituation and other learning processes discussed above, physical activity may importantly influence various forms of plasticity in the central nervous system. This hypothesis is in agreement with findings of plasticity-related protein regulation by physical activity (Ding et al. Citation2006a, Citation2006b). However, this hypothesis does not explain the regulation of some, but not other, acute stressor responses, or the lack of regulation of several instances of neuroendocrine response habituation to different stressors (restraint and ferret odors) between voluntary exercised vs. sedentary rats (Sasse et al. Citation2008b). Therefore, any mechanism(s) suggested to mediate the effects of exercise will have to contend with the variety of conditions demonstrated to be susceptible or resistant to prior physical activity. It is currently unknown which stress conditions and their associated responses will be susceptible to prior physical activity. Nonetheless, our data suggest that under certain conditions, a history of physical activity can reduce acute responses, and enhance the extent of habituation of these responses to repeated stressor exposures.
Declaration of interest: The authors report no conflicts of interest. The authors alone are responsible for the content and writing of the paper.
References
- Bakos J, Bobryshev P, Tillinger A, Kvetnansky R, Jezova D. 2008. Phenylethanolamine N-methyltransferase gene expression in the heart and blood pressure response to oxytocin treatment in rats exposed to voluntary wheel running. Ann N Y Acad Sci. 1148:302–307.
- Bhatnagar S, Vining C, Iyer V, Kinni V. 2006. Changes in hypothalamic–pituitary–adrenal function, body temperature, body weight and food intake with repeated social stress exposure in rats. J Neuroendocrinol. 18:13–24.
- Blaney J, Sothmann M, Raff H, Rart B, Horn T. 1990. Impact of exercise training on plasma adrenocorticotropin hormone response to a well learned vigilance task. Psychoneuroendocrinology. 15:453–462.
- Burow A, Day HE, Campeau S. 2005. A detailed characterization of loud noise stress: Intensity analysis of hypothalamo–pituitary–adrenocortical axis and brain activation. Brain Res. 1062:63–73.
- Calcagni E, Elenkov I. 2006. Stress system activity, innate and T helper cytokines, and susceptibility to immune-related diseases. Ann N Y Acad Sci. 1069:62–76.
- Campeau S, Dolan D, Akil H, Watson SJJr. 2002. c-Fos mRNA induction in acute and chronic audiogenic stress: Possible role of the orbitofrontal cortex in habituation. Stress. 5:121–130.
- Campeau S, Nyhuis TJ, Sasse SK, Kryskow EM, Herlihy L, Masini CV, Babb JA, Greenwood BN, Fleshner M, Day HEW. 2010. Hypothalamic pituitary adrenal axis responses to low intensity stressors are reduced following voluntary wheel running in rats. J Neuroendocrinol. 22:872–888.
- Carter RN, Pinnock SB, Herbert J. 2004. Does the amygdala modulate adaptation to repeated stress?. Neuroscience. 126:9–19.
- Chen X, Herbert J. 1995. Regional changes in c-Fos expression in the basal forebrain and brainstem during adaptation to repeated stress: Correlations with cardiovascular, hypothermic and endocrine responses. Neuroscience. 64:675–685.
- Chennaoui M, Gomez Merino D, Lesage J, Drogou C, Guezennec CY. 2002. Effects of moderate and intensive training on the hypothalamo–pituitary–adrenal axis in rats. Acta Physiol Scand. 175:113–121.
- Chrousos GP, Gold PW. 1998. A healthy body in a healthy mind – and vice versa – The damaging power of ‘uncontrollable’ stress. J Clin Endocrinol Metab. 83:1842–1845.
- Claytor RP, Cox RH, Howley ET, Lawler KA, Lawler JE. 1988. Aerobic power and cardiovascular response to stress. J Appl Physiol. 65:1416–1423.
- Cleroux J, Peronnet F, De Champlain J. 1985. Sympathetic indices during psychological and physical stimuli before and after training. Physiol Behav. 35:271–275.
- Cox RH, Hubbard JW, Lawler JE, Sanders BJ, Mitchell VP. 1985. Cardiovascular and sympathoadrenal responses to stress in swim-trained rats. J Appl Physiol. 58:1207–1214.
- De Boer SF, Slangen JL, van der Gugten J. 1988. Adaptation of plasma catecholamine and corticosterone responses to short-term repeated noise stress in rats. Physiol Behav. 44:273–280.
- Ding Q, Vaynman S, Akhavan M, Ying Z, Gomez-Pinilla F. Insulin-like growth factor I interfaces with brain-derived neurotrophic factor-mediated synaptic plasticity to modulate aspects of exercise-induced cognitive function. Neuroscience. 2006a; 140:823–833.
- Ding Q, Vaynman S, Souda P, Whitelegge JP, Gomez-Pinilla F. Exercise affects energy metabolism and neural plasticity-related proteins in the hippocampus as revealed by proteomic analysis. Eur J Neurosci. 2006b; 24:1265–1276.
- Dishman RK, Warren JM, Youngstedt SD, Yoo H, Bunnell BN, Mougey EH, Meyerhoff JL, Jaso-Friedmann L, Evans DL. 1995. Activity-wheel running attenuates suppression of natural killer cell activity after footshock. J Appl Physiol. 78:1547–1554.
- Dishman RK, Bunnell BN, Youngstedt SD, Yoo HS, Mougey EH, Meyerhoff JL. 1998. Activity wheel running blunts increased plasma adrenocorticotrophin (ACTH) after footshock and cage-switch stress. Physiol Behav. 63:911–917.
- Dobrakovova M, Kvetnansky R, Oprsalova Z, Jezova D. 1993. Specificity of the effect of repeated handling on sympathetic-adrenomedullary and pituitary-adrenocortical activity in rats. Psychoneuroendocrinology. 18:163–174.
- Droste SK, Gesing A, Ulbricht S, Muller MB, Linthorst AC, Reul JM. 2003. Effects of long-term voluntary exercise on the mouse hypothalamic–pituitary–adrenocortical axis. Endocrinology. 144:3012–3023.
- Droste SK, Chandramohan Y, Hill LE, Linthorst AC, Reul JM. 2007. Voluntary exercise impacts on the rat hypothalamic–pituitary–adrenocortical axis mainly at the adrenal level. Neuroendocrinology. 86:26–37.
- Droste SK, Collins A, Lightman SL, Linthorst ACE, Reul JMHM. 2009. Distinct, time-dependent effects of voluntary exercise on circadian and ultradian rhythms and stress responses of free corticosterone in the rat hippocampus. Endocrinology. 150:4170–4179.
- Fediuc S, Campbell JE, Riddell MC. 2006. Effect of voluntary wheel running on circadian corticosterone release and on HPA axis responsiveness to restraint stress in Sprague–Dawley rats. J Appl Physiol. 100:1867–1875.
- Fleshner M. 2000. Exercise and neuroendocrine regulation of antibody production: Protective effect of physical activity on stress-induced suppression of the specific antibody response. Int J Sports Med. 21 Suppl 1: S14–S19.
- Forcier K, Stroud LR, Papandonatos GD, Hitsman B, Reiches M, Krishnamoorthy J, Niaura R. 2006. Links between physical fitness and cardiovascular reactivity and recovery to psychological stressors: A meta-analysis. Health Psychol. 25:723–739.
- Fordyce DE, Wehner JM. 1993. Physical activity enhances spatial learning performance with an associated alteration in hippocampal protein kinase C activity in C57BL/6 and DBA/2 mice. Brain Res. 619:111–119.
- Greenwood BN, Foley TE, Day HEW, Campisi J, Hammack SH, Campeau S, Maier SF, Fleshner M. Freewheel running prevents learned helplessness/behavioral depression: Role of dorsal raphe serotonergic neurons. J Neurosci. 2003a; 23:2889–2898.
- Greenwood BN, Kennedy S, Smith TP, Campeau S, Day HE, Fleshner M. Voluntary freewheel running selectively modulates catecholamine content in peripheral tissue and c-Fos expression in the central sympathetic circuit following exposure to uncontrollable stress in rats. Neuroscience. 2003b; 120:269–281.
- Greenwood BN, Strong PV, Foley TE, Fleshner M. 2009. A behavioral analysis of the impact of voluntary physical activity on hippocampus-dependent contextual conditioning. Hippocampus. 19:988–1001.
- Grissom N, Kerr W, Bhatnagar S. 2008. Struggling behavior during restraint is regulated by stress experience. Behav Brain Res. 191:219–226.
- Hammen C, Davila J, Brown G, Ellicott A, Gitlin M. 1992. Psychiatric history and stress: Predictors of severity of unipolar depression. J Abnorm Psychol. 101:45–52.
- Hillman CH, Erickson KI, Kramer AF. 2008. Be smart, exercise your heart: Exercise effects on brain and cognition. Nat Rev Neurosci. 9:58–65.
- Ising H, Babisch W, Kruppa B. 1999. Noise-induced endocrine effects and cardiovascular risk. Noise Health. 1:37–48.
- Jedrziewski MK, Lee VM-Y, Trojanowski JQ. 2007. Physical activity and cognitive health. Alzheimer's Dement. 3:98–108.
- Konarska M, Stewart RE, McCarty R. 1989. Habituation of sympathetic-adrenal medullary responses following exposure to chronic intermittent stress. Physiol Behav. 45:255–261.
- Konarska M, Stewart RE, McCarty R. 1990. Habituation of plasma catecholamine responses to chronic intermittent restraint stress. Psychobiology. 18:30–34.
- Kramer AF, Erickson KI, Colcombe SJ. 2006. Exercise, cognition, and the aging brain. J Appl Physiol. 101:1237–1242.
- Lancel M, Droste SK, Sommer S, Reul JM. 2003. Influence of regular voluntary exercise on spontaneous and social stress-affected sleep in mice. Eur J Neurosci. 17:2171–2179.
- Martin SC, Steiger JL, Gravielle MC, Lyons HR, Russek SJ, Farb DH. 2004. Differential expression of gamma-aminobutyric acid type B receptor subunit mRNAs in the developing nervous system and receptor coupling to adenylyl cyclase in embryonic neurons. J Comp Neurol. 473:16–29.
- Masini CV, Day HE, Campeau S. 2008. Long-term habituation to repeated loud noise is impaired by relatively short interstressor intervals in rats. Behav Neurosci. 122:210–223.
- McEwen BS. 2000. Allostasis and allostatic load: Implications for neuropsychopharmacology. Neuropsychopharmacology. 22:108–124.
- McEwen BS. 2004. Protection and damage from acute and chronic stress: Allostasis and allostatic overload and relevance to the pathophysiology of psychiatric disorders. Ann N Y Acad Sci. 1032:1–7.
- Melzer K, Kayser B, Pichard C. 2004. Physical activity: The health benefits outweigh the risks. Curr Opin Clin Nutr Metab Care. 7:641–647.
- Moncek F, Duncko R, Johansson BB, Jezova D. 2004. Effect of environmental enrichment on stress related systems in rats. J Neuroendocrinol. 16:423–431.
- Moraska A, Fleshner M. 2001. Voluntary physical activity prevents stress-induced behavioral depression and anti-KLH antibody suppression. Am J Physiol Regul Integr Comp Physiol. 281:R484–R489.
- Morimoto K, Tan N, Nishiyasu T, Sone R, Murakami N. 2000. Spontaneous wheel running attenuates cardiovascular responses to stress in rats. Pflugers Arch Eur J Physiol. 440:216–222.
- Oguma Y, Shinoda-Tagawa T. 2004. Physical activity decreases cardiovascular disease risk in women: Review and meta-analysis. Am J Prev Med. 26:407–418.
- Overton JM, Kregel KC, Davis-Gorman G, Seals DR, Tipton CM, Fisher LA. 1991. Effects of exercise training on responses to central injection of CRF and noise stress. Physiol Behav. 49:93–98.
- Pitman D, Natelson B, Ottenweller J, McCarty R, Pritzel T, Tapp W. 1995. Effects of exposure to stressors of varying predictability on adrenal function in rats. Behav Neurosci. 109:767–776.
- Radak Z, Kaneko T, Tahara S, Nakamoto H, Pucsok J, Sasvari M, Nyakas C, Goto S. 2001. Regular exercise improves cognitive function and decreases oxidative damage in rat brain. Neurochem Int. 38:17–23.
- Rosch PJ. 1985. Exercise and stress reduction. Compr Ther. 11:10–15.
- Salmon P. 2001. Effects of physical exercise on anxiety, depression, and sensitivity to stress: A unifying theory. Clin Psychol Rev. 21:33–61.
- Sapolsky RM. 1996. Why stress is bad for your brain. Science. 273:749–750.
- Sasse SK, Greenwood BN, Masini CV, Nyhuis TJ, Fleshner M, Day HEW, Campeau S. Chronic voluntary wheel running facilitates corticosterone response habituation to repeated audiogenic stress exposure in male rats. Stress. 2008a; 11:425–437.
- Sasse SK, Masini CV, Nyhuis TJ, Day HEW, Campeau SSimilar rates of HPA axis response habituation to repeated predator odor or restraint stress observed between voluntarily exercised and sedentary male ratsSociety for Neuroscience. Washington, DC: 2008 Neuroscience Meeting Planner2008b782–783.
- Sinski M, Lewandowski J, Abramczyk P, Narkiewicz K, Gaciong Z. 2006. Why study sympathetic nervous system?. J Physiol Pharmacol. 57 Suppl 11: 79–92.
- Sinyor D, Peronnet F, Brisson G, Seraganian P. 1988. Failure to alter sympathoadrenal response to psychological stress following aerobic training. Physiol Behav. 42:293–296.
- Sinyor D, Schwartz S, Peronnet F, Brisson G, Seraganian P. 1983. Aerobic fitness level and reactivity to psychosocial stress: Physiological, biochemical, and subjective measures. Psychosom Med. 65:205–217.
- Sothmann MS, Gustafson AB, Garthwaite TL, Horn TS, Hart BA. 1988. Cardiovascular fitness and selected adrenal hormone responses to cognitive stress. Endocr Res. 14:59–69.
- Sothmann MS, Hart BA, Horn TS. 1991. Plasma catecholamine response to acute psychological stress in humans: Relation to aerobic fitness and exercise training. Med Sci Sports Exerc. 23:860–867.
- Sothmann MS, Hart BA, Horn TS. 1992. Sympathetic nervous system and behavioral responses to stress following exercise training. Physiol Behav. 51:1097–1103.
- Sothmann MS, Horn TS, Hart BA, Gustafson AB. 1987. Comparison of discrete cardiovascular fitness groups on plasma catecholamine and selected behavioral responses to psychological stress. Psychophysiology. 24:47–54.
- Stamp JA, Herbert J. 1999. Multiple immediate-early gene expression during physiological and endocrine adaptation to repeated stress. Neuroscience. 94:1313–1322.
- Swaab DF, Bao AM, Lucassen PJ. 2005. The stress system in the human brain in depression and neurodegeneration. Ageing Res Rev. 4:141–194.
- Vanitallie TB. 2002. Stress: A risk factor for serious illness. Metabolism. 51:40–45.