Abstract
Housing rodents in an enriched environment (EE) has been typically considered to have positive effects on well-being and cognitive functioning of the animals. However, in some strains of mice, EEs have also been reported to elicit aggression and to promote stress-related outcomes. In the current investigation, we examined whether environmental enrichment would elicit aggression among CD-1 male mice and thus sensitize responses to a subsequent mild stressor. It was first observed that mice housed in an EE for 2 weeks displayed more aggressive behaviors than did mice that had been housed in a standard environment (SE). In the second experiment, it was noted that after 4 weeks of EE or SE housing, mice exhibited comparable plasma corticosterone concentrations as well as levels of brain norepinephrine and its metabolite, 3-methoxy-4-hydroxyphenylglycol (MHPG), in the absence of a challenge. However, upon exposure to mild stressor (placement in a novel cage), relative to their SE counterparts, EE mice were more active and displayed higher plasma corticosterone concentrations and enhanced MHPG accumulation in the prefrontal cortex and hippocampus. It seems that enrichment in male CD-1 mice promotes aggression, and may sensitize biological processes, possibly increasing vulnerability to stressor-related outcomes.
Introduction
Stressful events influence neuroendocrine and brain neurotransmitter activity, and might thus contribute to the precipitation and exacerbation of psychopathologies, including anxiety and depression (Anisman et al. Citation2008; McEwen Citation2008). Some of the adverse effects of stressors, however, can be attenuated among animals housed in an enriched environment (EE) (Fox et al. Citation2006). In this respect, the beneficial effects of enrichment have been reported in relation to enhanced cognitive performance and increased expression of brain-derived neurotrophic factor (BDNF) mRNA (Falkenberg et al. Citation1992; van Praag et al. Citation2000), dendritic branching (Pham et al. Citation2002), and neurogenesis (Kempermann et al. Citation1998) in the hippocampus.
The impact of enrichment on emotionality (Roy et al. Citation2001) and markers of distress (Fox et al. Citation2006) appear to be less consistent. For example, resting corticosterone levels among enriched mice have been reported to be either increased (Van Loo et al. Citation2002; Marashi et al. Citation2003; Benaroya-Milshtein et al. Citation2004), decreased (Belz et al. Citation2003), or unchanged (Van de Weerd et al. Citation1997; Roy et al. Citation2001) compared to their standard-housed counterparts. Furthermore, enrichment in male mice was shown to promote aggression, causing wounding and stress-related physiological changes, thus creating a potentially stressful situation and reducing the well-being of these animals (Van Loo et al. Citation2002; Howerton et al. Citation2008).
Of the varied neurochemical changes elicited by stressors, elevated turnover of norepinephrine (NE) within the prefrontal cortex (PFC) and hippocampus is particularly reliable and has been related to several behavioral disturbances (Anisman et al. Citation2008). Specifically, in response to acute stressors the concentrations of NE and its metabolite, 3-methoxy-4-hydroxyphenylglycol (MHPG), increase appreciably, and if the stressor is sufficiently severe, NE concentrations may decline owing to the synthesis of the amine not keeping pace with its utilization (Anisman et al. Citation2008). Although stress-provoked NE and MHPG changes are relatively transient, the processes underlying monoamine functioning are subject to sensitization so that subsequent exposure to mild stressors may provoke more pronounced amine variations than in animals that had not previously been stressed. Indeed, it seems that even mild stressors that ordinarily have little effect may elicit marked monoamine variations in previously stressed animals (Anisman et al. Citation2003; Maier and Watkins Citation2005).
The current investigation examined whether housing male CD-1 mice in an EE would increase aggressive behaviors (Experiment 1). Moreover, we assessed whether relatively protracted housing in an EE would alter basal plasma corticosterone concentration, as well as NE and MHPG concentrations in the PFC and hippocampus, and whether this housing condition would exacerbate the effects of a mild stressor applied later (Experiment 2). Specifically, it was hypothesized that CD-1 male mice housed in an EE would display more aggressive behaviors than mice housed in a standard environment (SE). Furthermore, it was predicted that basal corticosterone and NE activity would be elevated among enriched mice, and that these outcomes would be enhanced in response to a mild stressor.
Materials and methods
Animals and housing procedures
For both experiments, naïve male CD-1 mice, aged 6–8 weeks, were obtained from Charles River Canada (St. Constant, QC, Canada) and housed (3–4/cage) upon arrival in either an EE or a SE. The EE consisted of large rat maternity polypropylene cages (50 × 40 × 20 cm) equipped with two running wheels, one red polypropylene shelter, one orange polypropylene shelter with an angled running wheel, as well as three yellow polypropylene tunnels and two cotton nestlets. The SE consisted of standard polypropylene cages (27 × 21 × 14 cm) with only one cotton nestlet. All mice were kept in the same room, on a 12 h light–dark cycle (lights on: 08:00h–20:00 h) in a temperature- (21°C) and humidity-controlled (63%) room and given ad libitum access to food and tap water.
In an attempt to minimize stress associated with novel objects (Lehmann and Herkenham Citation2011), enrichment items were not changed over the course of the experiment, except during cage cleaning once/week. In Experiment 1, home cage aggressive behaviors in SE and EE were scored daily for 2 weeks and commenced immediately upon arrival of the mice. In Experiment 2, mice were left undisturbed in their respective environments for 4 weeks, with the exception of routine cage cleaning once/week. All experimental procedures were approved by the Carleton University Animal Care Committee and met the guidelines set out by the Canadian Council on Animal Care.
Experiment 1: behavioral scoring
Mice were identified by tail markings. Frequency of aggressive interactions was scored daily over a 5-min interval in real time. A description of each aggressive encounter was also documented so that the interactions could be categorized as attacks, aggressive chasing, or aggressive grooming, all resulting in submissive behaviors in the targeted mouse. Attacks included biting, sometimes accompanied by tail rattling. Aggressive chasing included rushing or leaping at another mouse. Finally, aggressive grooming was defined as one mouse coming close to, or vigorously sniffing a second mouse (n = 31 mice/group).
Experiment 2: behavioral testing
Testing procedures were conducted between 08:30 and 13:00 h to minimize effects related to diurnal factors. After 4 weeks of living in their assigned environments, mice were brought to the testing area to acclimate for a 24 h period. On the test day, half of the mice in the EE and SE were exposed to a mild stressor that consisted of being taken from their home cage and placed individually in a clear novel cage (standard size: 27 × 21 × 14 cm) with fresh bedding. The remaining mice were not disturbed and stayed in their home cage (n = 10/group). During the novel cage stressor, motor activity was monitored through a MicroMax system (AccuScan Instruments Inc., OH, USA) composed of metal frames equipped with 16 pairs of infrared photobeam sensors (Model 20-MMAM), a distribution hub (Model 20-MMDB), and an analyzer (Model 20-MMA). The total number of beam interruptions during the 45-min period was used as the activity index.
Experiment 2: Blood collection and brain removal
Immediately after the 45-min novel cage exposure (or at corresponding times for mice in the no stressor conditions), mice were brought to a different room where they underwent rapid decapitation without anesthesia. Trunk blood was then immediately collected in tubes containing 10 mg ethylenediaminetetraacetic acid (EDTA), centrifuged, and the plasma stored at − 80°C for subsequent corticosterone determination.
Brains were rapidly removed, flash-frozen in isopropanol, and placed on dry ice for 20 s. Frozen brains were then placed on a stainless-steel brain matrix (2.5 × 3.75 × 2.0 cm) positioned on a block of ice that rested on dry ice. The matrix has a series of slots spaced 500 μm apart that guide razor blades to provide coronal brain sections. Once the brains were sliced, tissue from the PFC and hippocampus was collected following the mouse brain atlas of Franklin and Paxinos (Citation1997). Tissue punches were placed in 0.3 M monochloroacetic acid containing 10% methanol and internal standards and were stored at − 80°C for subsequent determination of NE and MHPG.
Experiment 2: Corticosterone determination
A commercial radioimmunoassay (RIA) kit (ICN Biomedicals, Inc., Costa Mesa, CA, USA) was used to determine plasma corticosterone concentrations (in duplicate). Assays were performed in a single run to prevent inter-assay variability; the intra-assay variability was < 10%. The assay sensitivity was 1.7 ng/ml.
Experiment 2: Determination of NE and MHPG concentrations
High-performance liquid chromatography (HPLC) was used to determine the concentrations of NE and MHPG. Tissue punches were sonicated in solution obtained from a stock solution that contained 14.17 g monochloroacetic acid, 0.0186 g EDTA, 5.0 ml methanol, and 500 ml HPLC grade water. Following centrifugation, a 20 μl aliquot of the supernatant was passed at a flow rate of 1.5 ml/min (1400–1600 psi) through a system containing a M-600 pump (Milford, PA, USA), guard column, radial compression column (5 m, C18 reverse phase, 8 mm × 10 cm), and a three-cell coulometric electrochemical detector (ESA model 5100A). For separation, a mobile phase was used comprising 1.3 g heptane sulfonic acid, 0.1 g disodium EDTA, 6.5 ml triethylamine, and 35 ml acetonitrile. The mobile phase was then filtered using 0.22-mm filter paper, degassed, and the pH was adjusted to 2.5 with phosphoric acid. The area and height of the peaks were determined using a Hewlett-Packard integrator. A protein analysis kit (Fisher Scientific, Ottawa, ON, Canada) and a spectrophotometer (Brinkman, PC800 colorimeter) in conjunction with bicinchoninic acid were used to measure protein concentrations of each sample. Neurotransmitter concentrations were based on protein levels. The lower limit of detection for the monoamines and metabolites was 5.0 pg/ml.
Data analyses
For Experiment 1, aggressive outcomes were analyzed through a one-way analysis of variance (ANOVA) (housing: standard vs. enriched). In Experiment 2, locomotor activity was analyzed through a 2 (housing) × 3 (blocks of time: three periods of 15 min) mixed measures ANOVA and plasma corticosterone concentrations as well as NE and MHPG concentrations in the PFC and hippocampus were analyzed through 2 (housing) × 2 (stressor: no stressor vs. novel cage stressor) between-groups ANOVAs. Follow-up comparisons comprised t-tests with a Bonferroni correction to maintain the alpha level at 0.05. In addition, Pearson's correlation coefficients were determined between plasma corticosterone, NE and MHPG concentrations within PFC and hippocampus. Data are presented as mean ± SEM.
Results
Experiment 1
For ethical reasons, highly aggressive mice, defined as those that repeatedly attacked other mice to the extent that injury occurred or was imminent, were removed from both EE and SE cages. When this was done, a new dominant mouse typically emerged and on some occasions, this mouse became excessively aggressive and also had to be removed from the study. After just 5 days, of the 31 mice in each condition, more of the EE mice (11) than the SE mice (4) were removed for this reason from the study (χ2 = 4.31, p < 0.05), attesting to the aggressive behavior associated with the enriched conditions (). Because a high number of aggressive mice had been removed at the beginning of day 5, aggressive scores were analyzed for days 1–4 only. Specifically, each category of aggressive encounters was scored every day and then averaged. Analyses of aggressive encounters during the 5-min daily assessment period indicated that more aggressive encounters were scored in EE than SE mice (), but this difference was not significant, F (1, 6) = 1.32. Aggressive chases, however, were significantly more common in EE mice than in SE mice, F (1, 6) = 6.82, p < 0.05. Furthermore, EE mice displayed significantly more wounds than did their SE counterparts, F (1, 6) = 7.56, p < 0.05.
Table I. Mean ± SEM number of aggressive encounters in the home cage of EE and SE mice.
Experiment 2
As depicted in , EE mice appeared more active than their SE counterparts during exposure to the novel cage, although this effect was not significant F (1, 16) = 3.39, p = 0.08.
Figure 1. Home cage motor activity over 15-min blocks of time in enriched (EE) and standard (SE) mice that had been exposed to a novel cage stressor for 45 min. Data are mean ± SEM, and analyzed by a 2 (housing) × 3 (blocks of time: three periods of 15 min) mixed measures ANOVA, n = 9/group.
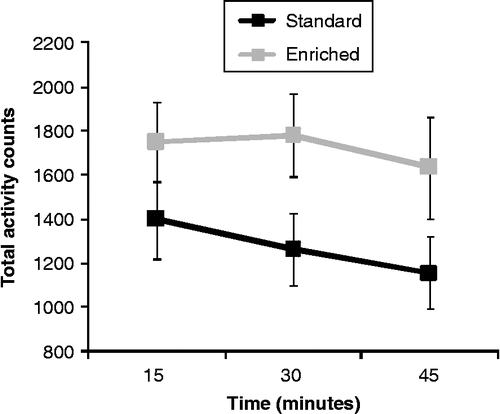
Plasma corticosterone concentrations varied as a function of the interaction between housing and stressor, F (1, 37) = 5.88, p < 0.05. Follow-up comparisons of the simple effects comprising this interaction indicated that after 30 days of EE and SE housing, hormone concentrations in the absence of a further stressor were comparable in the two conditions. However, placement in the novel cage markedly increased plasma corticosterone concentration in EE mice compared with their SE counterparts, p < 0.01 (). In contrast, after the 45-min novel cage stressor, plasma corticosterone concentrations were not changed in SE mice.
Figure 2. Plasma corticosterone concentrations (μg/dl) collected immediately after a 45-min novel cage stressor experience (novel cage stressor) or at a corresponding time in controls (no stressor) among mice that had been housed in enriched (EE) or standard (SE) conditions. Data are mean ± SEM. ** p < 0.01 vs. SE mice; 2 × 2 between-groups ANOVA, n = 10/SE no-stressor group, n = 10/SE novel cage stressor group, n = 8/EE no stressor group, n = 9/EE novel cage stressor group.
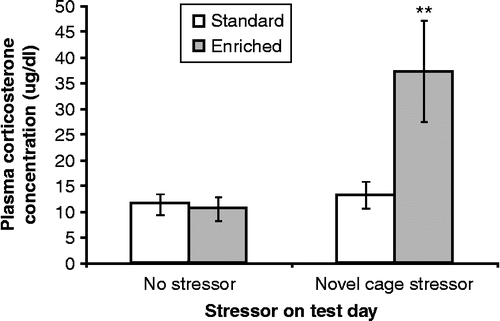
Variations in MHPG concentrations (top panels) in the PFC and hippocampus were strikingly similar (, respectively). In both the PFC and hippocampus, MHPG accumulation varied as a function of the housing × stressor interaction, F (1, 36) = 3.96 and 3.87, p = 0.05 and 0.058, respectively. Based on a priori predictions, follow-up comparisons of MHPG changes were conducted of the simple effects comprising these interactions. These analyses paralleled those evident with respect to corticosterone and indicated that in the absence of a further treatment, MHPG concentrations within the two brain structures were comparable in EE and SE mice. After the 45-min novel cage stressor, however, MHPG concentrations in both regions were elevated in EE mice relative to SE mice (all p < 0.05). Unlike MHPG variations, concentrations of the monoamine NE within the PFC and hippocampus (, middle panels) were not significantly affected by the housing or stressor conditions.
Figure 3. Concentrations of MHPG and NE and MHPH/NE ratios within the PFC collected immediately after a 45-min novel cage stressor experience (novel cage stressor) or at a corresponding time in controls (no stressor) among mice that had been housed in enriched (EE) or standard (SE) conditions. Data are mean ± SEM. *p < 0.05 and **p < 0.01 vs. SE mice; 2 × 2 between-groups ANOVAs, n = 10/SE no stressor group, n = 10/SE novel cage stressor group, n = 7/EE no stressor group, n = 9/EE novel cage stressor group.
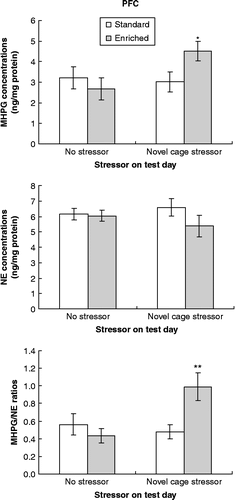
Figure 4. Concentrations of MHPG and NE and MHPH/NE ratios within the hippocampus collected immediately after a 45-min novel cage stressor experience (novel cage stressor) or at a corresponding time in controls (no stressor) among mice that had been housed in enriched (EE) or standard (SE) conditions. Data are mean ± SEM. *p < 0.05 vs. SE mice, # p < 0.05 vs. mice that had not been stressed on test day; 2 × 2 between-groups ANOVAs, n = 10/SE no stressor group, n = 10/SE novel cage stressor group, n = 7/EE no stressor group, n = 9/EE novel cage stressor group.
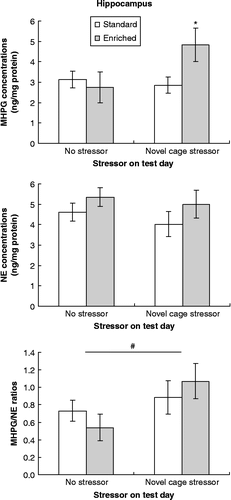
Within the PFC, MHPG/NE ratio (, bottom panel) varied as a function of the housing × stressor interaction, F (1, 36) = 7.29, p < 0.01. Follow-up comparisons of the simple effects comprising this interaction showed that NE turnover among SE mice was not significantly affected by the novel cage stressor, whereas among EE mice a significant increase in MHPG/NE ratio was apparent in stressed relative to non-stressed mice. Thus, after the novel cage stressor, the MHPG/NE ratio was increased in EE mice compared to SE mice (p < 0.01). Within the hippocampus, in contrast, MHPG/NE ratio was increased only by the stressor administered on the test day, F (1, 36) = 3.95, p < 0.05, irrespective of whether mice had been housed in SE or EE (, bottom panel).
Correlation analyses performed on all groups indicated that plasma corticosterone concentrations were positively correlated with MHPG concentrations in both the PFC (r = 0.43, p < 0.01) and hippocampus (r = 0.33, p < 0 .05), likely suggesting that these reflected general stress responses. In addition, MHPG concentrations in the PFC were negatively correlated with NE concentrations in the same brain region (r = − 0.36, p < 0.05). Although these data are correlational, they are consistent with the view that high levels of utilization elicited by a stressor, reflected by MHPG accumulation, favored the concentration of NE declining. Interestingly, when the correlation analyses included only EE mice exposed to the novel cage stressor, the correlations between MHPG and NE concentrations within the PFC were particularly strong (r = − 0.85, p < 0.01).
Discussion
Consistent with the previous reports (Howerton et al. Citation2008), environmental enrichment in the current investigation promoted high levels of aggression among male CD-1 mice, as reflected by the increased number of aggressive chases that occurred in EE mice, the elevated number of highly aggressive EE mice that were removed during the observation period of Experiment 1, and the wounding observed exclusively in the EE mice. It was not possible to conduct an analysis between social status (i.e. submissive/dominant mice) corticosterone and monoamine concentrations due to the unstable social hierarchies observed, especially as several overly aggressive mice were necessarily removed from the study. A less stable social hierarchy has previously been reported in EE mice compared to their SE counterparts and has been associated with both higher levels of stress and aggression (Haemisch et al. Citation1994). It is thus possible that in the present investigation, the unstable hierarchy apparent in EE cages was stressful and contributed to increased levels of aggression in enriched mice.
Housing mice in enriched conditions did not alter basal circulating corticosterone or NE utilization and turnover within the PFC and hippocampus. However, when confronted with a mild stressor, these stress markers appeared sensitized in mice that had been previously housed in EE conditions. In addition, EE mice appeared slightly more active when they were placed in the novel environment. It thus seems that EE CD-1 male mice might be more reactive to the effects of a subsequent stressor than mice that had been housed in standard conditions. This might be particularly pertinent considering that sensitization of neuroendocrine and neurochemical processes has been implicated in the development and the recurrence of anxiety- and depression-like symptoms (Post Citation1992; Anisman et al. Citation2003).
Interestingly, within the PFC, NE utilization and turnover in EE mice were elevated after mild stressor exposure and actual transmitter concentrations appeared slightly lower compared to their SE counterparts. Consistent with the notion that a stressor might reduce NE concentrations if utilization levels are excessive, prefrontal MHPG accumulation was inversely related to NE concentrations, and this was especially notable among EE mice. In the present investigation, the stressor administered on the test day was relatively mild, however, if a more intense stressor (e.g. social defeat) had been used, prefrontal NE functioning might have been overly taxed (i.e. allostatic overload; McEwen Citation2008) leading to significant amine reductions. Interestingly, NE turnover in the hippocampus was influenced only by the novel cage stressor and was not affected by whether the mice had been housed in EE or SE conditions. This raises the possibility that the PFC, at least in relation to NE neurotransmission, might be more sensitive to aggression, as previously suggested (Audet and Anisman Citation2010).
Mice have been reported to have a strong liking for enrichment items, especially for running wheels, and to be willing to work to access this resource (Olsson and Dahlborn Citation2002). As already suggested, in the present investigation, aggression promoted by enrichment may be due, in part, to competition for highly preferred or valued components of the EE so that certain animals may have been denied access to these resources (Nevison et al. Citation1999). The unstable hierarchy and high levels of aggression in the current investigation among male CD-1 mice were likely stressful and hence resulted in sensitized corticosterone and NE neuronal processes so that the subsequent mild challenge elicited enhanced hormone and NE activity. As previously suggested (Howerton et al. Citation2008), it is possible that enriched conditions involving group-housing, at least in CD-1 male mice, might not constitute a positive environment (as competitive access to resources promoted aggression rather than play behaviors). It is possible, however, that by increasing the duration of the enrichment period, more stable social hierarchies would have been established, thus eliciting more positive outcomes. This said, in the present investigation CD-1 mice were placed in standard or enriched cages upon their arrival from the breeding farm. Thus the advantageous effects of enriched housing, without the adverse effects comprising aggressive behaviors, might have been evident had the enrichment been instituted at weaning or if a less aggressive strain of male mice had been used.
Acknowledgements
This research was supported by the Canadian Institutes of Health Research (CIHR). H.A. holds a Canadian Research Chair in Neuroscience. R.M. is funded by the Natural Sciences and Engineering Council of Canada (NSERC). M-C.A. is funded by Fonds de la recherche en santé du Québec (FRSQ).
Declaration of interest: The authors report no conflicts of interest. The authors alone are responsible for the content and writing of the paper.
References
- Anisman H, Merali Z, Hayley S. 2003. Senstitization associated with stressors and cytokine treatments. Brain Behav Immun. 17:86–93.
- Anisman H, Merali Z, Hayley S. 2008. Neurotransmitter, peptide and cytokine processes in relation to depressive disorder: Comorbidity between depression and neurodegenerative disorders. Prog Neurobiol. 85:1–74.
- Audet MC, Anisman H. 2010. Neuroendocrine and neurochemical impact of aggressive social interactions in submissive and dominant mice: Implications for stress-related disorders. Int J Neuropsychopharmacol. 13:361–372.
- Belz EE, Kennell JS, Czambel RK, Rubin RT, Rhodes ME. 2003. Environmental enrichment lowers stress-responsive hormones in singly housed male and female rats. Pharmacol Biochem Behav. 76:481–486.
- Benaroya-Milshtein N, Hollander N, Apter A, Kulkulansky T, Raz N, Wilf A, Yaniv I, Pick CG. 2004. Environmental enrichment in mice decreases anxiety, attenuates stress responses and enhances natural killer cell activity. Eur J Neurosci. 20:1341–1347.
- Falkenberg T, Mohammed AK, Henriksson B, Persson H, Winblad B, Lindefors N. 1992. Increased expression of brain-derived neurotrophic factor mRNA in rat is associated with improved spatial memory and enriched environment. Neurosci Lett. 138:153–156.
- Fox C, Merali Z, Harrison C. 2006. Therapeutic and protective effect of environmental enrichment against psychogenic and neurogenic stress. Behav Brain Res. 175:1–8.
- Franklin KBJ, Paxinos G. 1997. A Stereotaxic Atlas of the Mouse Brain. San Diego, CA: Academic Press.
- Haemisch A, Voss T, Gartner K. 1994. Effects of environmental enrichment on aggressive behaviour, dominance hierarchies, and endocrine states in male DBA/2J mice. Physiol Behav. 56:1041–1048.
- Howerton CL, Garner JP, Mench JA. 2008. Effects of a running wheel-igloo enrichment on aggression, hierarchy linearity, and stereotypy in group-housed male CD-1 (ICR) mice. Appl Anim Behav Sci. 115:90–103.
- Kempermann G, Kuhn GH, Gage FH. 1998. Experience-induced neurogenesis in the senescent dentate gyrus. J Neurosci. 18:3206–3212.
- Lehmann ML, Herkenham M. 2011. Environmental enrichment confers stress resiliency to social defeat through an infralimbic cortex-dependent neuroanatomical pathway. J Neurosci. 31:6159–6173.
- Maier SF, Watkins LR. 2005. Stressor controllability and learned helplessness: The roles of the dorsal raphe nucleus, serotonin, and corticotropin-releasing factor. Neurosci Biobehav Rev. 29:829–841.
- Marashi V, Barnekow A, Ossendorf E, Sachser N. 2003. Effects of different forms of environmental enrichment on behavioural, endocrinological, and immunological parameters in male mice. Horm Behav. 43:281–292.
- McEwen BS. 2008. Central effects of stress hormones in health and disease: Understanding the protective and damaging effects of stress and stress mediators. Eur J Pharmacol. 583:174–185.
- Nevison CM, Hurst JL, Barnard CJ. 1999. Strain-specific effects of cage enrichment in male laboratory mice (Mus Musculus). Anim Welfare. 8:361–379.
- Olsson AS, Dahlborn K. 2002. Improving housing conditions for laboratory mice: A review of ‘environmental enrichment.’. Lab Anim. 36:243–270.
- Pham TM, Winblad B, Granholm AC, Mohammed AH. 2002. Environmental influences on brain neurotrophins in rats. Pharmacol Biochem Behav. 73:167–175.
- Post RM. 1992. Transduction of psychosocial stress into the neurobiology of recurrent affective disorder. Am J Psychiatry. 149:999–1010.
- Roy V, Belzung C, Delarue C, Chapillon P. 2001. Environmental enrichment in BALB/c mice effects in classical tests of anxiety and exposure to a predatory odor. Physiol Behav. 74:313–320.
- Van de Weerd HA, Van Loo PLP, Van Zutphen LFM, Koolhaas JM, Baumans V. 1997. Nesting material as environmental enrichment has no adverse effects on behaviour and physiology of laboratory mice. Physiol Behav. 62:1019–1028.
- Van Loo PLP, Kruitwagen CLJJ, Koolhaas JM, Van de Weerd HA, Van Zutphen LFM, Baumans V. 2002. Influence of cage enrichment on aggressive behaviour and physiological parameters in male mice. Appl Anim Behav Sci. 76:65–81.
- van Praag H, Kempermann G, Gage FH. 2000. Neural consequences of environmental enrichment. Nat Rev Neurosci. 1:191–198.