Abstract
Exposure to an uncontrollable stressor elicits a constellation of physiological and behavioral sequel in laboratory rats that often reflect aspects of anxiety and other emotional disruptions. We review evidence suggesting that plasticity within the serotonergic dorsal raphe nucleus (DRN) is critical to the expression of uncontrollable stressor-induced anxiety. Specifically, after uncontrollable stressor exposure subsequent anxiogenic stimuli evoke greater 5-HT release in DRN terminal regions including the amygdala and striatum; and pharmacological blockade of postsynaptic 5-HT2C receptors in these regions prevents expression of stressor-induced anxiety. Importantly, the controllability of stress, the presence of safety signals, and a history of exercise mitigate the expression of stressor-induced anxiety. These stress-protective factors appear to involve distinct neural substrates; with stressor controllability requiring the medial prefrontal cortex, safety signals the insular cortex and exercise affecting the 5-HT system directly. Knowledge of the distinct yet converging mechanisms underlying these stress-protective factors could provide insight into novel strategies for the treatment and prevention of stress-related psychiatric disorders.
Introduction
Stressor exposure precedes numerous psychiatric conditions, but importantly, not all persons exposed to stress develop psychopathology. Many years and resources have been devoted to discovering the physiological, genetic and experiential factors that interact with stressor exposure to precipitate psychiatric diseases (Alleva & Francia, Citation2009; Hauger et al., Citation2009; Jovanovic & Ressler, Citation2010; Mehta & Binder, Citation2012; Parker & Maestripieri, Citation2011; Schmidt et al, Citation2008). An increasing effort has focused upon factors that correlate with stress “resistant” or “resilient” outcomes (Cabib et al., Citation2012; Dudley et al., Citation2011; Franklin et al., Citation2012; Russo et al., Citation2012; Scharf & Schmidt, Citation2012). Stress resistance and resilience have been defined by a number of different authors and most recently by Russo and colleagues as “the capacity of an individual to avoid negative social, psychological and biological consequences of extreme stress that would otherwise compromise their psychological or physical well being” (Russo et al., Citation2012). Stress resistance relates to the capacity of an organism to defend against or withstand the effects of harmful environmental stimuli whereas resilience is marked by recuperative processes that permit an organism or tissue to return to a basal condition (see Fleshner et al., Citation2011 for further discussion). After stressor exposure resilience and resistance could be phenotypically identical. Most of the existing research, including the work reviewed herein, has not attempted to isolate these phenomena. The perception of control over a stressor (Southwick et al., Citation2005; Southwick & Charney, Citation2012), the predictability of stress onset or offset (Jovanovic et al., Citation2010) and regular exercise (Greenwood & Fleshner, Citation2011) are factors that mitigate many of the consequences of stressors. The aim of this review is to describe these stress-protective factors and the neural mechanisms that are critical to each.
The literature discussed herein follows from the “learned helplessness” procedure in rats that has been extensively reviewed (Hammack et al., Citation2012; Maier et al., Citation2006; Maier & Watkins, Citation2005, Citation2010). In this procedure, one group of rats is exposed to a series of unpredictable tailshocks that may be terminated by a behavioral response, e.g. turning a wheel, while a second group of rats are “yoked” to the first group but without control over shock termination. An experimental control group is exposed to equal exposure to tail restraint or left undisturbed in the vivarium. In a typical experiment rats exposed to uncontrollable tailshock express changes in numerous behaviors for a period of 24–72 h after stress, including reduced social exploration (Christianson et al., 2008b; Short & Maier, Citation1993), enhanced fear (Baratta et al., Citation2007; Maier, Citation1990), instrumental learning deficits (Jackson et al., Citation1980; Seligman & Maier, Citation1967), and enhanced drug seeking behaviors (Will et al., Citation1998). Importantly, rats that were able to control shock offset behaved as if the stressor never occurred. These bidirectional “stressor controllability effects” have been the focus of much research.
Here, we describe how stressor-induced sensitization of projections of the brainstem serotonin system mediates the behavioral consequences of uncontrollable stress and how control over stress, safety signals and voluntary exercise mitigate these behavioral and physiological changes. We suggest a number of parallels between the tailshock procedure used in our laboratories to various other preclinical animal models and human psychiatric conditions with the overall goal to provide insight into how basic research models could shed light on optimal strategies for the treatment and prevention of stress-related psychiatric conditions.
The dorsal raphe nucleus circuit
A circuit involving the serotonergic dorsal raphe nucleus (DRN) mediates many of the consequences of uncontrollable tailshocks. The first studies implicating DRN activity in the acquisition of tailshock effects on later behavior did so by manipulating the DRN at the time of stressor exposure. Indeed, activation of the DRN is both necessary and sufficient to produce the shuttlebox escape performance deficits and enhanced fear expression that follows uncontrollable tailshock. Inhibition of DRN 5-HT activity during uncontrollable tailshock by intra-DRN administration of a variety of pharmacological agents including a 5-HT1A agonist (Christianson et al., 2008b; Maier et al., Citation1995), an α-1 adrenoreceptor antagonist (Grahn et al., Citation2002), a benzodiazepine (Maier et al., Citation1994), an NMDA receptor antagonist (Maier et al., Citation1994), and a corticotrophin releasing hormone receptor 2 antagonist (Hammack et al., Citation2003) all blocked the typical effects of IS. From these results it was suggested that release of 5-HT from recurrent collateral terminals within the DRN caused a desensitization of 5-HT1A autoreceptors resulting in a sensitized state wherein the 5-HT neurons become hyper-responsive to subsequent stimuli. It followed that upon exposure to a stimulus that may typically evoke a small DRN response, a rat exposed to prior uncontrollable tailshock would mount a larger 5-HT response both within the DRN and in DRN projection regions.
Recently, Rozeske et al. (Citation2011a) used an in vitro electrophysiology and pharmacology approach to directly test the hypothesis that DRN sensitization occurs as a result of downregulation of the somatodendritic 5-HT1A autoreceptor. Application of 5-HT or 5-HT1A receptor agonists rapidly and reversibly inhibits 5-HT unit activity (Sprouse & Aghajanian, Citation1987). Thus, increasing concentrations of 5-HT or the 5-HT1A agonist ipsapirone were applied to acute slices 24 h after tailshock (). 5-HT reduced spontaneous firing rates in DRN neurons of all rats, but significantly greater concentrations were required after uncontrollable shock compared to either stress-naïve or rats that had received controllable shock (Rozeske et al., Citation2011a). Sensitivity to 5-HT recovered 7 d after uncontrollable tailshock, a point at which uncontrollable stress has no apparent effect on social exploration (; Christianson et al., Citation2013) or shuttle box escape (Maier, Citation2001). This study provided clear evidence that uncontrollable stress alters the sensitivity of DRN neurons to extracellular 5-HT1A receptor agonists with a time course that is consistent with the behavioral consequences. However, attempts to quantify a decrease in 5-HT1A protein and mRNA after stress have produced inconsistent results (Greenwood & Fleshner, Citation2011; Rozeske et al., Citation2011a), thus leaving the molecular basis for this phenomenon the focus of future research. Notably, Riad and colleagues demonstrated rapid internalization of DRN 5-HT1A autoreceptors following systemic fluoxetine, which raises extracellular 5-HT levels in the DRN (Descarries & Riad, Citation2012). Therefore, the temporary sensitization of the DRN produced by uncontrollable stress could be due to receptor trafficking, rather than changes in receptor protein synthesis or gene transcription. Regardless of the mechanism, it is likely that sensitization of the DRN is a common substrate underlying numerous effects of uncontrollable stress.
Figure 1. Uncontrollable stress disrupts anxiety-like behavior and DRN 5-HT1A receptors for a period of 1-3 days. TOP: Rats were exposed to escapable/controllable (ES), inescapable/uncontrollable (IS) or no stress and then tested for social exploration either 1, 3 or 7 d later (originally published in Christianson et al., Citation2013). IS significantly reduced the time spent interacting with a juvenile conspecific when tested 1 or 3 d after stress (*ps < 0.05, compared to ES and no stress) but exploration returned to unstressed levels by 7 d. MIDDLE: Rats were exposed to ES, IS or not stress and acute DRN slices were prepared for extracellular recordings 24 h later. Bath application of increasing doses of 5-HT reduced unit responses (presented relative to pre-drug baseline). However, prior IS rendered 5-HT neurons less sensitive to inhibition by 5-HT. *p < 0.05 and significant main effect of IS. BOTTOM: Rats were exposed to IS and acute DRN slices were taken at different times after stress. Prior IS decreased sensitivity to the 5-HT1A receptor agonist ipsapirone only 1 d after stress. Middle and Bottom figures were recreated from (Rozeske et al., Citation2011a) with original data provided by the corresponding author.
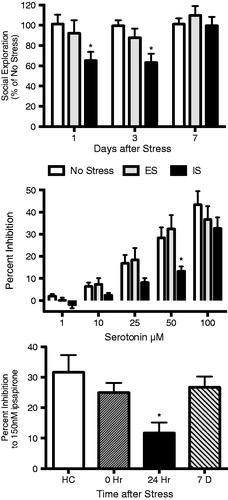
As noted above, one consequence of a sensitized DRN would be a potentiated release of 5-HT in DRN target regions upon subsequent stimulation of the DRN during stress such as associated with behavioral testing. The majority of experiments reviewed have utilized fear conditioning, shuttlebox escape learning, opiate administration or social interaction tests. During each of these cases, prior uncontrollable tailshock caused an increase in extracellular 5-HT in numerous DRN target regions (). This was first demonstrated in the basolateral amygdala. For these experiments, the post-shock freezing and shuttlebox testing typically commences with two mild footshocks, which likely excite the DRN. Twenty-four hours after uncontrollable tailshock, these two footshocks caused greater increase in extracellular 5-HT within the basolateral amygdala (Amat et al., 1998b), ventral hippocampus (Amat et al., 1998a) and dorsal striatum (Strong et al., Citation2011). Likewise, exposure to a juvenile also led to increased 5-HT release in the basolateral amygdala 24 h after tailshock (Christianson et al., Citation2010). Lastly, prior uncontrollable tailshock increased 5-HT release in the nucleus accumbens shell and medial prefrontal cortex to systemic administration of morphine (Bland et al., Citation2003a,Citationb).
Table 1. Effects of uncontrollable stress on serotonin release and behavior in forebrain targets of the DRN.
The DRN is topographically organized such that small populations of 5-HT neurons in the DRN have distinct ascending projections; thus, DRN 5-HT neurons are positioned to modulate a range of behaviors depending upon the efferent target (Hale et al., Citation2012). Site-specific administration of serotonergic compounds has revealed that 5-HT receptors in the amygdala modulate anxiety-like behaviors (Campbell & Merchant, Citation2003); in the prefrontal cortex, attention and drug seeking behaviors (Carli et al., Citation2006); and in the striatum, modulate goal-driven behaviors (Mitchell et al., Citation2007; Tanaka et al., Citation2009). In the last several years, the focus of our research has been to determine the mechanisms underlying the expression of “learned helplessness” in post-stress behavioral tests. Many of these studies have been designed to determine whether sensitized 5-HT release in DRN projection regions is necessary for the expression of uncontrollable stress effects. At the time we began these experiments, support was growing for a general role of 5-HT2C receptors in anxiety-like states (Millan, Citation2005). Importantly, systemic administration of SB242084, a brain penetrant, potent and selective 5-HT2C antagonist (Kennett et al., Citation1997), immediately before behavioral testing dose-dependently reversed the shuttle escape deficit (Strong et al., Citation2009) and reduction in social exploration (Christianson et al., Citation2010) that typically follow 24 h after uncontrollable stress. Accordingly, administration of SB242084 into the BLA just prior to social exploration tests reversed the stress-induced deficit in social exploration in a dose-dependent fashion without affecting social exploration per se (Christianson et al., Citation2010). Intra-dorsal striatum SB242084 completely blocked the instrumental shuttle-escape deficit (Strong et al., Citation2011). To our surprise, neither systemic nor intra-BLA SB242084 significantly affected shock elicited freezing (Strong et al., Citation2009, Citation2011) despite the fact that 5-HT2C agonists enhance fearful behaviors (Burghardt et al., Citation2007; Campbell & Merchant, Citation2003; Ravinder et al., Citation2013; Strong et al., Citation2009), including when they are administered into the BLA at concentrations known to alter other behavioral endpoints (Strong et al., Citation2011). Thus, non-5-HT2C postsynaptic 5-HT receptors in the BLA, or other DRN projection regions, may be critical for enhancement of fear produced by uncontrollable stressors.
Stressor controllability and the medial prefrontal cortex circuit
As noted above, behavioral control over tailshock prevents the behavioral and neurochemical effects that normally follow uncontrollable shock exposure. In addition, the experience of control over tailshock produces an enduring (at least 32 d; Kubala et al., Citation2012) protection against the behavioral and DRN-activating effects of later uncontrollable stressors including tailshock, social defeat and forced swim (Amat et al., Citation2006, Citation2010; Christianson et al., 2008b, Citation2013; Rozeske et al., Citation2012). Because DRN activation is critical to the effects of uncontrollable stress, it was hypothesized that factors that protect against those effects would prevent DRN sensitization. Both uncontrollable and controllable tailshock activate regions that send excitiatory input to the DRN (Amat et al., Citation2001), but only uncontrollable tailshock results in a strong activation of 5-HT neurons within the DRN. Animals with behavioral control over shock do not show sustained activation of 5-HT neurons in the DRN as determined by microdialysis (Amat et al., Citation2005; Maswood et al., Citation1998) and quantification of Fos protein in serotonergic neurons (Grahn et al., Citation1999). Interestingly, at the onset of the shock session, regardless of controllability, extracellular 5-HT concentrations in the DRN, which reflect the activity of the 5-HT released by recurrent collaterals (Matos et al., Citation1996), almost triple, but 5-HT levels in animals with behavioral control return to baseline at about the same time that the escaping animal becomes proficient with the escape response contingency. This differential effect of stressor controllability would be produced if the controllable stressor were to provide inhibition to the DRN.
The DRN does not receive the necessary somatosensory inputs to detect or process whether behavioral control over shock is present. However, the DRN receives input from the prelimbic (PL) region of the medial prefrontal cortex (mPFC). The PL is capable of detecting the escape contingency (see below) and is positioned to regulate the brainstem response to stress because of direct projections to the DRN. The PL efferents to the DRN are glutametergic (Gabbott et al., Citation2005) and synapse preferentailly on GABA interneurons (Jankowski & Sesack, Citation2004). As expected from this anatomy, activation of descending PL pyramidal neurons enhances GABAergic inhibition within the DRN and decreases 5-HT neuronal activity (Celada et al., Citation2001). Based on the anatomical relationship between the PL and DRN, the hypothesis was tested that the behavioral and neurochemical protection afforded by controllable stress would depend upon activity in the PL during stressor exposure. Microinjection of the GABAA agonist muscimol into the mPFC prior to controllable tailshock completely blocked the stress protective benefit of stressor controllability. Thus, when the mPFC was inhibited, 5-HT neurons in the DRN responded as if the shock was uncontrollable and rats subsequently exhibited exaggerated fear, shuttle escape failures (Amat et al., Citation2005), reduced social exploration (Christianson et al., Citation2009) and potentiated drug seeking (Rozeske et al., Citation2009). In other words, turning off the mPFC during controllable tailshock made the subject appear as if it experienced uncontrollable stress on both behavioral and physiological measures assessed following controllable tailshock. Baratta et al (Citation2009) demonstrated using a retrograde tracing technique that controllable tailshock led to a significant increase in the number of Fos immunoreactive neurons in the PL that project to the DRN when compared to the same population of neurons in rats that received either uncontrollable tailshock or no stress. Uncontrollable tailshock did not induce Fos in this population of neurons. This result implies that the critical activity blocked by intra-mPFC muscimol is in the PL-DRN circuit. A number of experiments further implicate the mPFC as critical to the stress-protective effect of behavioral control. Pharmacological activation of the mPFC with the chloride channel antagonist picrotoxin during an uncontrollable stressor prevented the interference in shuttle escape, reduction in social exploration, and increases in 5-HT transmission that the uncontrollable stressor would normally produce (Amat et al., Citation2008; Christianson et al., Citation2009). After an initial experience with control, inactivation of the mPFC at the time of a later uncontrollable stressor eliminated the protective effects of the prior controllable stressor (Amat et al., Citation2006). Control appears to cause plasticity-like changes within the mPFC in the form of increased circuit and intrinsic excitability. Evidence for neural plasticity in the PL was first reported in the retrograde tracing study of Baratta and colleagues. Rats were first exposed to controllable tailshock and then one week later exposed to uncontrollable tailshocks. As noted above, uncontrollable tailshock alone does not activate PL projections to the DRN; however, these neurons expressed Fos after uncontrollable tailshock if the rat had prior controllable tailshock (Baratta et al., Citation2009). These results clearly indicated that the PL was changed by the experience of controllable tailshock. One potential mechanism for the change in activity would be a change in the excitability in this population of neurons or their inputs. In a first attempt to characterize the intrinsic excitability of PL neurons after controllable tailshock Varela and colleagues conducted whole-cell patch clamp recordings of PL layer V pyramidal neurons of rats after either controllable or uncontrollable tailshock, or no stressor exposure. In the study, neurons from rats exposed to controllable stress exhibited larger action potentials indicated by significantly greater action potential amplitude and faster rise rates and larger after depolarizations when compared to the action potentials from rats with either uncontrollable or no prior stressor exposure. These changes are thought to reflect increased Na+ and T-type Ca+ conductances after controllable stress (Varela et al., Citation2012). The majority of the aforementioned microinjection studies targeted both the PL and the infralimbic (IL) cortex. However, the PL and IL are known to have distinguishable roles in a variety of behavioral tasks (Dalley et al., Citation2004). Recent, unpublished experiments from our laboratories have injected separately to either PL or IL in some of the aforementioned paradigms, and these effects only occur with PL injections. This is consistent with the anatomy as the DRN receives virtually all of its cortical innervation from PL, with only sparse projections from IL (Vertes, Citation2004).
In considering the various functions and circuits in which the PL is known to participate (Dalley et al., Citation2004), recent research indicates that instrumental learning is encoded by corticostriatal circuitry in which the PL specifically plays a prominent role (Dayan & Balleine, Citation2002). In concert with the posterior dorsal medial striatum (pDMS) the PL forms an “action/outcome system” that mediates instrumental learning and then regulates subsequent action selection (Balleine & O’Doherty, Citation2010). This system allows the organism to acquire response-outcome associations and to use contingency information about the relationship between its actions and outcomes. If either the PL or pDMS is inactivated, instrumental learning and instrumental responses can still occur, but the learned response is then insensitive to the value of the outcome (the reward) (Yin & Knowlton, Citation2006).
Learning an action/outcome contingency is precisely what is required for the rat with control over stress. That is, the probability of tailshock termination is higher if the rat turns the wheel than if the rat does not turn the wheel. The requirement for the PL-pDMS in this type of learning has been studied with positive reinforce such as food; whether or not the same circuitry mediates negative reinforcement such as occurs in the case of stressor controllability is unknown. A major goal of ongoing research is to determine if, in fact, control over an aversive event engages the PL and pDMS as part of engaging the action/outcome learning corticostriatal circuit. This approach may appear to be a stark contrast to readers familiar with the original theory of learned helplessness (Maier & Seligman, Citation1976) in which it was suggested that the learning occurred in the subjects treated with uncontrollable tailshock (hence the name learned helplessness). The current data, however, imply that, although uncontrollable stress impairs subsequent contingency learning, it is not the learning by the uncontrollably stressed rats that defines the crucial difference between subjects given controllable or uncontrollable tailshock. Instead, the critical learning occurs in the subjects with control over stress, and this learning involves the mPFC. Had these data been available at the time of the discovery of stressor controllability phenomenon, the founders may have more aptly named the phenomenon “learned controllability”, to reflect the fact that the enduring effects that reflect learning occur primarily in the controllably stressed group. Indeed, this language may have avoided a common assumption in the literature that all consequences of uncontrollable stress are part of learned helplessness phenomenon, as “learned controllability” emphasizes the critical distinction between groups in experiments employing escape-yoke designs (Greenwood et al., Citation2010; Maier & Watkins, Citation2005 for similar arguments).
Safety signals and the insular cortex
While the preceding section suggested that control over stress affords stress protection by recruiting the PL-pDMS action/outcome learning system, we have tested alternative hypotheses. The action/outcome system became obvious only after a significant effort was made in pursuit of a very old hypothesis in the stressor controllability literature. Shortly after reporting the effects of uncontrollable and controllable stress on gastric ulceration, Weiss replicated the controllability phenomenon by providing rats with a cessation signal at the end of the tailshock (Weiss, Citation1971). An idea gained traction in the 1980s that upon performance of the instrumental response, an internal cue would predict the inter shock interval in which the occurrence of a shock was unlikely for a period of time. This sort of stimulus is regarded as a “conditioned inhibitor” – a stimulus that predicts when an unconditioned stimulus will not occur and so will inhibit the reflex associated with the US (Rescorla, Citation1969). In the context of a stress experiment, conditioned inhibitors are called safety signals because they predict the periods of relative safety (Christianson et al., Citation2012). A safety signal is not merely a neutral stimulus, one that does not produce fear; it is an inhibitor of fear. Thus, if it is presented in a situation where there is some level of ambient fear such as intermittent tailshocks, it will reduce fear. Since Weiss’ first demonstration, numerous stressor effects have been prevented or reduced by providing safety signals (Jackson & Minor, Citation1988; Maier & Keith, Citation1987; Mineka et al., Citation1984; Minor et al., Citation1990; Weiss, Citation1971). It was hypothesized that since controllable stress involved the mPFC, which is also involved in several forms of behavioral inhibition including fear inhibition (Quirk & Beer, Citation2006), that the “active ingredient” of stress controllability was the generation of safety signals. Thus, rats were provided with a 5 s visual safety signal at the beginning of each shock free period during a session of inescapable tailshock. Twenty-four hours later, rats with the safety signal behaved identically to non-stressed rats in a social exploration test (Christianson et al., 2008a). Surprisingly, inhibition of the mPFC had no apparent influence on this phenomenon.
Alternative structures were not readily apparent as mediators of the stress-protective safety signal effects; candidate structures would require sensory inputs and outputs to stress-responsive circuits. The caudal granular insular cortex (cgIC; for a discussion of insular cortex nomenclature in rat please see Benison et al., Citation2011; Rodgers et al., Citation2008) emerged as a leading candidate because it receives both auditory and somatosensory inputs (Remple et al., Citation2003; Shi & Cassell, Citation1998a), is associated with a somatotopically organized body representation (Benison et al., Citation2007), exhibits convergent responses to simultaneous multisensory stimulation (Rodgers et al., Citation2008), receives intracortical and thalamocortical connectivity (Shi & Cassell, Citation1998b) and projects to the amygdala (McDonald et al., Citation1999; Shi & Cassell, Citation1998a). Thus, the anatomical and physiological evidence suggested that the cgIC might integrate sensory information for the computation required for detecting safety and affect downstream stress responsive structures, namely the amygdala. Indeed, pre-training lesions and temporary inactivation of the cgIC prevented the stress-protective effect of the safety signal on subsequent social exploration (Christianson et al., 2008a, Citation2011). This finding introduced a novel role for the insular cortex in stress protection and opened an exciting new area of research into how safety signals and the insula alter the expression of fear.
While control over stress operates by inhibition of the DRN, safety signals do not (Christianson et al., 2008a). Thus, an alternative mechanism was considered: safety signals would reduce the amount of fear conditioning that occurs during stress exposure. The expression of fearful behaviors, such as freezing, is the product of a serial neural circuit. Briefly, sensory information is relayed from the thalamus and sensory cortex to the lateral amygdala, which excites the basal amygdala and consequent outputs of the medial nucleus of the central amygdala and bed nucleus of the stria terminalis. These outputs excite the periaqueductal gray and freezing occurs. While this is an oversimplified schema, it nevertheless captures a wealth of data (Kim & Jung, Citation2006). Providing safety signals reduced both the time spent freezing during a series of unpredictable footshocks and the number of Fos immunoreactive neurons in the lateral amygdala, basolateral amygdala and bed nucleus of the stria terminalis (lateral ventral region, Christianson et al., Citation2011). Importantly, inhibition or lesion of the amygdala or bed nucleus of the stria terminalis prevented the effects of uncontrollable tailshock on numerous behavioral endpoints (Christianson et al., Citation2011; Hammack et al., Citation2004; Maier et al., Citation1993). These results suggest that safety signals offer stress protection by inhibiting fear expression and the signaling cascade in the amygdala that responds to danger.
The double-dissociation of circuitries underlying stressor controllability and safety signals introduced two important, unresolved questions. First, do safety signals reproduce the constellation of stress-protective effects that occur when the stressor is controllable? Although few reports directly compared controllable stress to safety signals, safety signals prevent stress-induced analgesia (Maier & Keith, Citation1987), fear conditioning (Mineka et al., Citation1984), shuttle escape failures (Minor et al., Citation1990) and social exploration deficits (Christianson et al., 2008a) that typically follow uncontrollable, but not controllable tailshock. Perhaps the most striking stress-protective effect of controllable stress is the behavioral immunization phenomenon in which a single session of controllable stress protects against the behavioral and physiological consequences of an uncontrollable stressor experienced later (Amat et al., Citation2006). Exposure to uncontrollable stress with a safety signal does not appear to have this sort of effect (J. P. Christianson and S. F. Maier, unpublished data). It is possible that safety signals offer protection from a limited array of stressor consequences that are under the control of the insular cortex and amygdala. The second issue relates to 5-HT. Safety signals do not inhibit stressor-induced DRN activity as does control over stress (Christianson et al., 2008a). The importance of DRN sensitization to the expression of uncontrollable stress effects described in this review suggests that providing a safety signal either (1) does not alter DRN response to stress but does prevent changes in 5-HT1A autoreceptors that lead to sensitization, (2) decreases or inhibits inputs to the DRN that are driven during social exploration or other post stress tests or (3) renders DRN efferents (such as the amygdala) less sensitive to modulation by 5-HT. The first possibility has not been tested but is unlikely given that the cgIC does not innervate the DRN (Sato et al., Citation2012). The second possibility is tenable. Corticotrophin releasing hormone expressing regions of the bed nucleus innervate the DRN (Dong et al., Citation2001) and activation of DRN CRHR2 receptors is critical to the expression of learned helplessness (Hammack et al., Citation2003). Although the existing studies have only examined Fos immunoreactivity in the amygdala and bed nucleus, differential activity during stress with safety signals may induce plasticity within these structures such that subsequent anxiogenic stimuli, such as a novel conspecific in the social interaction test, or increases in 5-HT do not excite these circuits. These issues are the focus of ongoing research.
Voluntary exercise
Exercise is another environmental factor that can increase stress resistance. Similar to the antidepressant and anxiolytic effects of exercise in humans, rodents allowed voluntary access to running wheels for a period of weeks are protected against depression- and anxiety-like behaviors produced by exposure to a variety of laboratory stressors (Greenwood & Fleshner, Citation2011; Sciolino & Holmes, Citation2012 for recent reviews). Identification of the mechanisms underlying the beneficial impact of exercise could lead to novel prevention and treatment strategies for stress-related disorders and could help encourage susceptible individuals to engage in exercise.
Interestingly, the stress resistance produced by exercise includes behaviors caused by uncontrollable tailshock including exaggerated shock-elicited fear (Greenwood et al., Citation2003a), shuttle box escape deficits (Dishman et al., Citation1997; Greenwood et al., Citation2003a), social avoidance (Greenwood et al., 2012a), and potentiation of morphine conditioned place preference (Rozeske et al., Citation2011b). Because, the protective effects of exercise occur in behavioral tests that depend upon hyperactivation and sensitization of DRN 5-HT neurons, one might predict that the mechanisms responsible for the beneficial effects of exercise (1) involve modulation of 5-HT systems, and (2) resemble the mechanisms underlying behavioral immunization produced by prior experience with controllable stress. Much work over the past decade provides insight into these possibilities.
In addition to the common stress-induced behaviors prevented by both controllability and exercise, several observations suggest that controllability and exercise share overlapping stress-protective mechanisms. First, similar to the persistent protective effect of controllable stress against future exposure to uncontrollable stress (the behavioral immunization effect), exercise produces a lasting protective effect against stress-induced social avoidance, exaggerated shock-elicited fear, and the shuttle box escape deficit that persists between 15 and 25 d following cessation of exercise (Greenwood et al., 2012a). Second, like rats exposed to controllable tailshock, rats allowed 6 weeks of access to running wheels, relative to their sedentary counterparts, demonstrate constrained activation of DRN 5-HT neurons during exposure to uncontrollable tailshock stress (Greenwood et al., Citation2003a). A time course study revealed that the protective effect of exercise against the behavioral and DRN-hyperactivating effects of uncontrollable stress are linked: whereas 3 weeks of wheel running neither prevents the behavioral effects of stress nor constrains activation of DRN 5-HT neurons, 6 weeks of wheel running does both (Greenwood et al., 2005a). The constrained activation of the DRN in response to stress in physically active rats was accompanied by attenuation of stress-induced increases in a 5-HT metabolite in the amygdala (Dishman et al., Citation1997), and modulation of Fos induction in circuitries putatively involved in both driving DRN activity during stress, (i.e. the noradrenergic locus coeruleus; Greenwood et al., Citation2003b) and in responding to DRN sensitization during behavioral testing (i.e. the bed nucleus of the stria terminalis; Greenwood et al., Citation2003b). Finally, although the increase in hippocampal brain-derived neurotrophic factor (BDNF) following exercise could be involved in beneficial effects of exercise such as enhanced memory (Greenwood et al., Citation2009; Vaynman et al., Citation2004), hippocampal BDNF does not seem to be involved in the protective effects of either controllable stress (Bland et al., Citation2007) or exercise (Greenwood et al., Citation2007) against the behavioral consequences of uncontrollable tailshocks.
These similarities suggest that controllable stress and exercise might increase resistance to uncontrollable stress through similar mechanisms. Indeed, like learning to turn a wheel in order to terminate tailshock during controllable stress, wheel running could be considered a controllable, instrumental learning task (in this case an appetitive one) that might recruit the PL-pDMS circuitry. In this case, the action of running in a wheel provides reward (Greenwood et al., Citation2011; Lett et al., Citation2000). It follows that wheel running would thus produce plasticity in the circuitry that is hypothesized to underlie controllable stress. During exposure to uncontrollable tailshock, the mPFC of physically active rats would be activated and would inhibit the activation of 5-HT neurons in the DRN.
Two approaches were used to test the role of the mPFC in exercise-induced stress resistance. First, bilateral mPFC cannulae were implanted during week three of wheel running and muscimol was injected through the cannulae immediately prior to uncontrollable tailshock to inactivate the mPFC. If the mPFC was responsible for inhibiting the DRN in physically active rats during stress, then inactivation of the mPFC should restore DRN hyperactivation and the behavioral consequences of uncontrollable stress in previously physically active rats. Surprisingly, however, inactivation of the mPFC had no effect and physically active rats remained protected against both the exaggerated fear and escape deficit produced by tailshock (B. N. Greenwood and J. P. Christianson, unpublished data).
Although these data were inconsistent with a role for the mPFC in mediating the stress-resistant effects of exercise, it was possible that the mPFC would constrain activation of the DRN in physically active rats during behavioral testing 24 hours after stress, despite its being inactivated during stress. To determine if the mPFC is at all required for the protective effect of exercise against the behavioral consequences of stress, the mPFC was lesioned with ibotenic acid after three weeks of exercise. Rats continued to run after surgery and were exposed to uncontrollable stress 3 weeks later. The results were consistent with the muscimol data, suggesting that the mPFC is not necessary for exercise-induced stress resistance. Physical activity still protected rats lacking a mPFC against both the exaggerated fear and deficit in shuttle box escape produced by uncontrollable stress (Greenwood et al., 2013).
These data lead to the conclusions that the mPFC is not required for the protective effects of exercise against the behavioral consequences of uncontrollable stress, and that exercise recruits a mechanism for stress resistance that is unique from that of stressor controllability. These observations, however, do not preclude the importance of exercise-adaptations in the 5-HT system in the protective effects of exercise. Indeed, evidence to date suggests that exercise modulates the 5-HT system directly, and that plasticity in the 5-HT system is an adaptation to exercise critical to its stress-buffering effects (reviewed in Greenwood & Fleshner, Citation2011).
Using primarily in situ hybridization to investigate the effects of wheel running on gene expression of factors capable of modulating the central 5-HT system, we have identified exercise-induced adaptations that could contribute to the protective effect of exercise against the behavioral consequences of stress. These adaptations seem to occur both within the DRN itself, as well as in DRN projection sites. Changes within the DRN include altered mRNA levels for the 5-HT transporter, 5-HT1B autoreceptor, and the 5-HT1A autoreceptor (Greenwood et al., 2005b). Of these factors sensitive to exercise, only the change in 5-HT1A autoreceptor mRNA level occurs in a time course consistent with the behavioral effects of exercise (6 weeks but not 3 weeks of wheel running increases 5-HT1A mRNA) (Greenwood et al., Citation2003a, 2005b). Given that constraint over activity of the DRN provided by the mPFC is not necessary for the protective effect of exercise against the behavioral consequences of stress, an increase in 5-HT1A autoreceptors in the DRN following exercise could instead be important. Indeed, elevated levels of 5-HT1A autoreceptors could themselves provide constraint over activation of DRN 5-HT neurons during stressor exposure by increasing auto-inhibition of 5-HT neurons and thus resisting autoreceptor desensitization (Rozeske et al., Citation2011a). The observation that 6 weeks of wheel running prevents the interference with escape behavior elicited by pharmacological elevation of 5-HT in the DRN (via intra-DRN administration of citalopram; Greenwood & Fleshner, Citation2008) is consistent with exercise-induced increase in 5-HT1A autoreceptors within the DRN being an adaptation sufficient to prevent sensitization of DRN 5-HT neurons.
Exercise not only alters gene expression within the DRN, it impacts gene expression in DRN projection sites. A growing body of evidence suggests that exercise reduces the expression and sensitivity of 5-HT2 – family receptors (Broocks et al., Citation1999; Fox et al., Citation2008). We have recently narrowed this effect to 5-HT2C, the receptor critical for the expression of numerous behavioral consequences of uncontrollable stress. Specifically, higher doses of the 5-HT2C agonist CP-809101 were required to enhance fear and interfere with escape behavior when injected into the either BLA or dorsal striatum of physically active, compared to sedentary rats (Greenwood et al., 2012b). These behavioral effects of exercise were accompanied by a reduction in 5-HT2C mRNA in the amygdala and the pDMS (Greenwood et al., 2012b). This pattern exactly opposite of what has been reported following uncontrollable stress: Harada et al. (Citation2008) demonstrated that a single prolonged stressor significantly increased 5-HT2C mRNA in the amygdala. These data indicate that a reduction of 5-HT2C is associated with stress resistance conferred by habitual exercise.
The identification of 5-HT2C receptor downregulation as a mechanism for exercise-induced stress protection seems to have rendered the effect of exercise on the DRN moot. Indeed, exercise could prevent the behavioral effects of excessive 5-HT (and therefore the behavioral consequences of uncontrollable stress) by reducing the post-synaptic effects of 5-HT at the 5-HT2C, and this would occur regardless of any potential impact of exercise on activity of DRN 5-HT neurons during stress or behavioral testing. Recall, however, that exercise prevents all behavioral consequences of uncontrollable stress yet investigated that depend on hyperactivation of DRN 5-HT neurons during stress, not simply sensitized 5-HT responses and 5-HT2C activation in the amygdala or dorsal striatum during behavioral testing. Uncontrollable stress-induced potentiation of the rewarding effect of morphine, for example, is prevented by 6 weeks of wheel running and is dependent upon hyperactivation of the ascending DRN input to the mPFC (Bland et al., Citation2004). Since 5-HT2C receptor gene expression has not been quantified in the mPFC after exercise, it remains a possible mechanism in mediating stress-induced potentiation of drug reward. Nevertheless, attenuation of the DRN response to uncontrollable stress can account for the protective effect of exercise against stress-enhanced morphine conditioned place preference. Taken together, these studies suggest that exercise-induced plasticity within the DRN, in concert with adaptations in 5-HT receptors within DRN projection sites, provides robust protection from a host of stressor consequences.
Identification of the signals and mechanisms by which exercise impacts the 5-HT system remains an important topic of inquiry. Given that persistent increases in 5-HT neurotransmission due to 5-HT transporter knockout (Moya et al., Citation2011) or antidepressant treatment (Barbon et al., Citation2011) can decrease 5-HT2C sensitivity and full length 5-HT2C mRNA, it is possible that subtle increases in 5-HT neurotransmission during repeated exercise could contribute to the reduced expression and/or sensitivity of 5-HT2C. This would imply that repeated elevations of 5-HT neurotransmission during exercise would be required for the reduction in 5-HT2C expression and the stress-protective effects of exercise. Although we have not yet tested this possibility, recent data indicate that 5-HT is required for the antidepressant-like effects of wheel running in the forced swim test (Cunha et al., Citation2013).
Conclusions
Control over stress, safety signals and habitual exercise protect animals from the anxiety-like consequences of uncontrollable tailshock in dissociable ways. Central to our view is the assumption that stress-protective factors act by altering the acute consequences of uncontrollable stressor exposure. The serotonergic neurons originating in the DRN and with ascending projections to the amygdala, striatum and prefrontal cortex mediate the acute anxiety-like behavioral consequences of tailshock. Control over stress appears to regulate the ascending 5-HT system by a top-down inhibition from the PL region of the mPFC to the DRN. Voluntary exercise, on the other hand, appears to be independent of top-down inhibition. Using a variety of pharmacological and anatomical tools, we have demonstrated that voluntary exercise (1) alters DRN physiology to favor inhibition and (2) renders post synaptic 5-HT2C receptors less sensitive to agonists. Either or both of these phenomena would decrease the impact on 5-HT on behavior following stress. Lastly, providing safety signals in the midst of unpredictable and uncontrollable stress mitigates the consequences of the stressor much like exercise and control over stress, yet existing data suggest that safety signals do not directly influence the ascending serotonergic system. Instead, safety signals may render limbic targets of the DRN less sensitive to subsequent stimulation. Thus, stress-protective factors operate via distinguishable mechanisms yet converge upon common final pathways, in this case the serotonergic system and associated projections (). This review is not meant to be exhaustive of all environmental variables known to alter subsequent stress reactivity. Interested readers should also consider how rearing and social conditions (Stevens et al., Citation2009; Stiller et al., Citation2011; Suomi, Citation2006), enriched environments (Green et al., Citation2010) and early life stressors (Lyons & Macri, Citation2011) offer protection from stress.
Figure 2. Stress protective neurocircuits. Exposure to an aversive, uncontrollable stressor, excessively activates serotonin (5-HT) neurons in the dorsal raphe nucleus (DRN), leading to desensitization of 5-HT1A inhibitory autoreceptors located on DRN 5-HT neurons. 5-HT1A autoreceptor desensitization removes an important source of inhibitory control over DRN activity, such that DRN 5-HT neurons respond to subsequent challenge with excessive 5-HT release in DRN projection sites. During behavioral testing, extracellular 5-HT in the (1) dorsal striatum (pDMS) interferes with instrumental escape behavior through a mechanism involving 5-HT2C receptors (5-HT2C), (2) medial prefrontal cortex (mPFC) potentiates rewarding effects of abusive drugs, and (3) basolateral amygdala (AMG) elicits fear and anxiety, with anxiety mediated by 5-HT2C activity. Stress-resistant manipulations that prevent the behavioral consequences of uncontrollable stress act through distinct, yet converging mechanisms. Behavioral control over stress (heavy dashed red lines) recruits top-down inhibitory control over DRN 5-HT activity during stress through projections from the prelimbic cortex (PL). Safety signals (blue line) inhibit excessive fear and anxiety elicited by uncontrollable stress through a mechanism involving projections from the sensory insular cortex to the bed nucleus of the stria terminalis (BNST). Habitual exercise (light dotted black line) produces plasticity in the 5-HT system; including an increase in 5-HT1A autoreceptor expression in the DRN and reduced sensitivity of 5-HT2C in the pDMS and AMG. These mechanisms for stress resistance and resilience provide insight into how controllability, safety learning, and exercise could help protect individuals against genetic (5-HT1A, 5-HT transporter) or environmental (early life stress, exposure to trauma) vulnerabilities to stress-related psychiatric disorders.
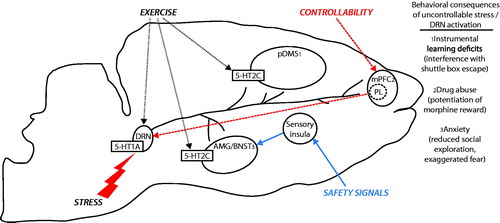
The work reviewed above is consistent with a role for the ascending DRN serotonergic system in other preclinical models of anxiety. A considerable body of evidence indicates that inhibition of the DRN or postsynaptic blockade of 5-HT receptors, including the 5-HT2C, prevent the expression of anxiety-like behaviors. For instance, repeated withdrawals from ethanol produces social avoidance which is reversed by systemic (File et al., Citation1993a,Citationb; Overstreet et al., Citation2003) or intra-DRN 5-HT1A agonist, or intra-BLA 5-HT2C antagonists (Overstreet et al., Citation2006). These studies resonate with 5-HT1A receptor binding positron emission tomography imaging studies in peer-reared versus mother-reared Rhesus monkeys. Peer-reared monkeys exhibit anxiety and stressor vulnerability (Stevens et al., Citation2009; Suomi, Citation2006), which correlate with decreased 5-HT1A receptor binding in the brainstem (Spinelli et al., Citation2010). Exposure to social defeat in hamsters produces conditioned, anxiety behaviors that are blocked by intra-DRN 5-HT1A agonists (Cooper et al., Citation2008, Citation2009) and post synaptic 5-HT1A and 5-HT2C antagonists (Harvey et al., Citation2012; Morrison & Cooper, Citation2012) (see Hammack et al. (Citation2012) for thoughtful comparison of the learned helplessness and social defeat paradigms). Recently, Morrison et al. (Citation2012) identified that hamsters with dominant social status appear to be resistant to the conditioned effects of social defeat. Similar to control over stress, the resistant phenotype correlated with induction of Fox in the mPFC and was prevented by mPFC inactivation (Morrison et al., Citation2013). Taken together, these preclinical studies provide compelling evidence for enhanced 5-HT transmission as a mediator of stressor induced anxiety-like behavior. Plasticity within the 5-HT system, or its inputs such as the mPFC, could therefore be critical for protection from stressor-induced anxiety. Importantly, these preclinical observations resonate with several clinical reports. Reduced brainstem 5-HT1A receptor binding has been found in anxiety, panic disorder and major depression (Drevets et al., Citation2007; Lanzenberger et al., Citation2007; Nash et al., Citation2008; Neumeister et al., Citation2004). As in the tailshock model, a consequence of low levels of DRN 5-HT1A receptors could be a sensitized ascending 5-HT system that could disrupt cortical and limbic functions as a pathophysiological mechanism in anxiety and depression (see Holmes, Citation2008 for recent review).
Translating the lessons learned from rodent studies of control, safety and exercise to the clinic is promising and should provide alternatives to the inadequate existing pharmacotherapies that act solely via monaminergic systems. Connecting specific neural circuits with symptoms of behavioral syndromes is a relatively new direction that has grown from technological advances in human brain imaging and is a current priority in translational psychiatry (Insel et al., 2010). Hypoactivity of the mPFC and insular cortex can occur in anxiety disorders (Etkin & Wager, Citation2007), and may reflect a preexisting pathology that impairs plasticity in these regions. Thus, therapies that foster plasticity or strengthen cortical control over the brainstem and limbic stress-responsive circuits should improve these conditions. Indeed, emerging evidence suggests that cognitive therapy, pharmacotherapies, intracranial electrical stimulation and transcranial magnetic stimulation restore emotional balance by fostering plasticity within the prefrontal cortex (Clark & Beck, Citation2010; Duman & Aghajanian, Citation2012; Fox et al., Citation2012; Holtzheimer & Mayberg, Citation2011).
Although the mPFC is no doubt an important target for enabling stress resistance, the work presented in the current review suggests that potential alternative therapies should not focus solely on the mPFC. Indeed, we present alternative stress protective circuits that converge on the ascending DRN serotonergic system and could provide alternate targets for fostering stress resistance and resilience. Although insular cortex abnormalities are reported in numerous clinical conditions (Paulus & Stein, Citation2010), and impaired safety learning is a consequence of posttraumatic stress disorder (Jovanovic et al., Citation2010), translational studies aimed to bolster insular cortex function have not been reported. Finally, the preclinical data suggest that exercise could offer the most lasting stabilization of stressor-responsive neural circuits. Given that withdrawal from physical activity is a common symptom in stress-related disorders, therapies aimed at maintaining physical activity could prove promising for the prevention and treatment of these disorders. In conclusion, the current review provided evidence that exercise and therapies aimed to foster the perception of control over stressors could help prevent the development of many deleterious effects of uncontrollable traumas. The continued study of stress resistance and resilience should lead to exciting advances in the treatment of stress-related psychopathologies.
Declaration of interest
The authors report no conflicts of interest. The authors alone are responsible for the content and writing of this article.
References
- Alleva E, Francia N. (2009). Psychiatric vulnerability: suggestions from animal models and role of neurotrophins. Neurosci Biobehav Rev 33:525–36
- Amat J, Aleksejev RM, Paul E, Watkins LR, Maier SF. (2010). Behavioral control over shock blocks behavioral and neurochemical effects of later social defeat. Neuroscience 165:1031–8
- Amat J, Baratta MV, Paul E, Bland ST, Watkins LR, Maier SF. (2005). Medial prefrontal cortex determines how stressor controllability affects behavior and dorsal raphe nucleus. Nat Neurosci 8:365–71
- Amat J, Matus-Amat P, Watkins LR, Maier SF. (1998a). Escapable and inescapable stress differentially and selectively alter extracellular levels of 5-HT in the ventral hippocampus and dorsal periaqueductal gray of the rat. Brain Res 797:12–22
- Amat J, Matus-Amat P, Watkins LR, Maier SF. (1998b). Escapable and inescapable stress differentially alter extracellular levels of 5-HT in the basolateral amygdala of the rat. Brain Res 812:113–20
- Amat J, Paul E, Watkins LR, Maier SF. (2008). Activation of the ventral medial prefrontal cortex during an uncontrollable stressor reproduces both the immediate and long-term protective effects of behavioral control. Neuroscience 154:1178–86
- Amat J, Paul E, Zarza C, Watkins LR, Maier SF. (2006). Previous experience with behavioral control over stress blocks the behavioral and dorsal raphe nucleus activating effects of later uncontrollable stress: role of the ventral medial prefrontal cortex. J Neurosci 26:13264–72
- Amat J, Sparks PD, Matus-Amat P, Griggs J, Watkins LR, Maier SF. (2001). The role of the habenular complex in the elevation of dorsal raphe nucleus serotonin and the changes in the behavioral responses produced by uncontrollable stress. Brain Res 917:118–26
- Balleine BW, O’Doherty JP. (2010). Human and rodent homologies in action control: corticostriatal determinants of goal-directed and habitual action. Neuropsychopharmacology 35:48–69
- Baratta MV, Christianson JP, Gomez DM, Zarza CM, Amat J, Masini CV, Watkins LR, Maier SF. (2007). Controllable versus uncontrollable stressors bi-directionally modulate conditioned but not innate fear. Neuroscience 146:1495–503
- Baratta MV, Zarza CM, Gomez DM, Campeau S, Watkins LR, Maier SF. (2009). Selective activation of dorsal raphe nucleus-projecting neurons in the ventral medial prefrontal cortex by controllable stress. Eur J Neurosci 30:1111–16
- Barbon A, Orlandi C, La Via L, Caracciolo L, Tardito D, Musazzi L, Mallei A, et al. (2011). Antidepressant treatments change 5-HT2C receptor mRNA expression in rat prefrontal/frontal cortex and hippocampus. Neuropsychobiology 63:160–8
- Benison AM, Chumachenko S, Harrison JA, Maier SF, Falci SP, Watkins LR, Barth DS. (2011). Caudal granular insular cortex is sufficient and necessary for the long-term maintenance of allodynic behavior in the rat attributable to mononeuropathy. J Neurosci 31:6317–28
- Benison AM, Rector DM, Barth DS. (2007). Hemispheric mapping of secondary somatosensory cortex in the rat. J Neurophysiol 97:200–7
- Bland ST, Hargrave D, Pepin JL, Amat J, Watkins LR, Maier SF. (2003a). Stressor controllability modulates stress-induced dopamine and serotonin efflux and morphine-induced serotonin efflux in the medial prefrontal cortex. Neuropsychopharmacology 28:1589–96
- Bland ST, Schmid MJ, Watkins LR, Maier SF. (2004). Prefrontal cortex serotonin, stress, and morphine-induced nucleus accumbens dopamine. Neuroreport 15:2637–41
- Bland ST, Tamlyn JP, Barrientos RM, Greenwood BN, Watkins LR, Campeau S, Day HE, Maier SF. (2007). Expression of fibroblast growth factor-2 and brain-derived neurotrophic factor mRNA in the medial prefrontal cortex and hippocampus after uncontrollable or controllable stress. Neuroscience 144:1219–28
- Bland ST, Twining C, Watkins LR, Maier SF. (2003b). Stressor controllability modulates stress-induced serotonin but not dopamine efflux in the nucleus accumbens shell. Synapse 49:206–8
- Broocks A, Meyer T, George A, Hillmer-Vogel U, Meyer D, Bandelow B, Hajak G, et al. (1999). Decreased neuroendocrine responses to meta-chlorophenylpiperazine (m-CPP) but normal responses to ipsapirone in marathon runners. Neuropsychopharmacology 20:150–61
- Burghardt NS, Bush DE, McEwen BS, LeDoux JE. (2007). Acute selective serotonin reuptake inhibitors increase conditioned fear expression: blockade with a 5-HT(2C) receptor antagonist. Biol Psychiatry 62:1111–18
- Cabib S, Campus P, Colelli V. (2012). Learning to cope with stress: psychobiological mechanisms of stress resilience. Rev Neurosci 23:659–72
- Campbell BM, Merchant KM. (2003). Serotonin 2C receptors within the basolateral amygdala induce acute fear-like responses in an open-field environment. Brain Res 993:1–9
- Carli M, Baviera M, Invernizzi RW, Balducci C. (2006). Dissociable contribution of 5-HT1A and 5-HT2A receptors in the medial prefrontal cortex to different aspects of executive control such as impulsivity and compulsive perseveration in rats. Neuropsychopharmacology 31:757–67
- Celada P, Puig MV, Casanovas JM, Guillazo G, Artigas F. (2001). Control of dorsal raphe serotonergic neurons by the medial prefrontal cortex: Involvement of serotonin-1A, GABA(A), and glutamate receptors. J Neurosci 21:9917–29
- Christianson JP, Benison AM, Jennings J, Sandsmark EK, Amat J, Kaufman RD, Baratta MV, et al. (2008a). The sensory insular cortex mediates the stress-buffering effects of safety signals but not behavioral control. J Neurosci 28:13703–11
- Christianson JP, Drugan RC, Flyer JG, Watkins LR, Maier SF. (2013). Anxiogenic effects of brief swim stress are sensitive to stress history. Prog Neuropsychopharmacol Biol Psychiatry 44:17--22
- Christianson JP, Fernando AB, Kazama AM, Jovanovic T, Ostroff LE, Sangha S. (2012). Inhibition of fear by learned safety signals: a mini-symposium review. J Neurosci 32:14118–24
- Christianson JP, Jennings JH, Ragole T, Flyer JG, Benison AM, Barth DS, Watkins LR, Maier SF. (2011). Safety signals mitigate the consequences of uncontrollable stress via a circuit involving the sensory insular cortex and bed nucleus of the stria terminalis. Biol Psychiatry 70:458–64
- Christianson JP, Paul ED, Irani M, Thompson BM, Kubala KH, Yirmiya R, Watkins LR, Maier SF. (2008b). The role of prior stressor controllability and the dorsal raphe nucleus in sucrose preference and social exploration. Behav Brain Res 193:87–93
- Christianson JP, Ragole T, Amat J, Greenwood BN, Strong PV, Paul ED, Fleshner M, et al. (2010). 5-hydroxytryptamine 2C receptors in the basolateral amygdala are involved in the expression of anxiety after uncontrollable traumatic stress. Biol Psychiatry 67:339–45
- Christianson JP, Thompson BM, Watkins LR, Maier SF. (2009). Medial prefrontal cortical activation modulates the impact of controllable and uncontrollable stressor exposure on a social exploration test of anxiety in the rat. Stress 12:445–50
- Clark DA, Beck AT. (2010). Cognitive theory and therapy of anxiety and depression: convergence with neurobiological findings. Trends Cogn Sci 14:418–24
- Cooper MA, Grober MS, Nicholas CR, Huhman KL. (2009). Aggressive encounters alter the activation of serotonergic neurons and the expression of 5-HT1A mRNA in the hamster dorsal raphe nucleus. Neuroscience 161:680–90
- Cooper MA, McIntyre KE, Huhman KL. (2008). Activation of 5-HT1A autoreceptors in the dorsal raphe nucleus reduces the behavioral consequences of social defeat. Psychoneuroendocrinology 33:1236–47
- Cunha MP, Oliveira A, Pazini FL, Machado DG, Bettio LE, Budni J, Aguiar ASJ, et al. (2013). The antidepressant-like effect of physical activity on a voluntary running wheel. Med Sci Sports Exerc 45:851--9
- Dalley JW, Cardinal RN, Robbins TW. (2004). Prefrontal executive and cognitive functions in rodents: neural and neurochemical substrates. Neurosci Biobehav Rev 28:771–84
- Dayan P, Balleine BW. (2002). Reward, motivation, and reinforcement learning. Neuron 36:285–98
- Descarries L, Riad M. (2012). Effects of the antidepressant fluoxetine on the subcellular localization of 5-HT1A receptors and SERT. Philos Trans R Soc Lond B Biol Sci 367:2416–25
- Dishman RK, Renner KJ, Youngstedt SD, Reigle TG, Bunnell BN, Burke KA, Yoo HS, et al. (1997). Activity wheel running reduces escape latency and alters brain monoamine levels after footshock. Brain Res Bull 42:399–406
- Dong HW, Petrovich GD, Watts AG, Swanson LW. (2001). Basic organization of projections from the oval and fusiform nuclei of the bed nuclei of the stria terminalis in adult rat brain. J Comp Neurol 436:430–55
- Drevets WC, Thase ME, Moses-Kolko EL, Price J, Frank E, Kupfer DJ, Mathis C. (2007). Serotonin-1A receptor imaging in recurrent depression: replication and literature review. Nucl Med Biol 34:865–77
- Dudley KJ, Li X, Kobor MS, Kippin TE, Bredy TW. (2011). Epigenetic mechanisms mediating vulnerability and resilience to psychiatric disorders. Neurosci Biobehav Rev 35:1544–51
- Duman RS, Aghajanian GK. (2012). Synaptic dysfunction in depression: potential therapeutic targets. Science 338:68–72
- Etkin A, Wager TD. (2007). Functional neuroimaging of anxiety: a meta-analysis of emotional processing in PTSD, social anxiety disorder, and specific phobia. Am J Psychiatry 164:1476–88
- File SE, Andrews N, al-Farhan M, Wu PY. (1993a). The role of 5-HT in the anxiogenic effects of acute ethanol withdrawal and in the long-lasting cognitive deficits. Alcohol Alcohol Suppl 2:495–9
- File SE, Andrews N, al-Farhan M. (1993b). Anxiogenic responses of rats on withdrawal from chronic ethanol treatment: effects of tianeptine. Alcohol Alcohol 28:281–6
- Fleshner M, Maier SF, Lyons DM, Raskind MA. (2011). The neurobiology of the stress-resistant brain. Stress 14:498–502
- Fox JH, Hammack SE, Falls WA. (2008). Exercise is associated with reduction in the anxiogenic effect of mCPP on acoustic startle. Behav Neurosci 122:943–8
- Fox MD, Buckner RL, White MP, Greicius MD, Pascual-Leone A. (2012). Efficacy of transcranial magnetic stimulation targets for depression is related to intrinsic functional connectivity with the subgenual cingulate. Biol Psychiatry 72:595–603
- Franklin TB, Saab BJ, Mansuy IM. (2012). Neural mechanisms of stress resilience and vulnerability. Neuron 75:747–61
- Gabbott PL, Warner TA, Jays PR, Salway P, Busby SJ. (2005). Prefrontal cortex in the rat: projections to subcortical autonomic, motor, and limbic centers. J Comp Neurol 492:145–77
- Grahn RE, Hammack SE, Will MJ, O’Connor KA, Deak T, Sparks PD, Watkins LR, Maier SF. (2002). Blockade of alpha1 adrenoreceptors in the dorsal raphe nucleus prevents enhanced conditioned fear and impaired escape performance following uncontrollable stressor exposure in rats. Behav Brain Res 134:387–92
- Grahn RE, Will MJ, Hammack SE, Maswood S, McQueen MB, Watkins LR, Maier SF. (1999). Activation of serotonin-immunoreactive cells in the dorsal raphe nucleus in rats exposed to an uncontrollable stressor. Brain Res 826:35–43
- Green TA, Alibhai IN, Roybal CN, Winstanley CA, Theobald DE, Birnbaum SG, Graham AR, et al. (2010). Environmental enrichment produces a behavioral phenotype mediated by low cyclic adenosine monophosphate response element binding (CREB) activity in the nucleus accumbens. Biol Psychiatry 67:28–35
- Greenwood BN, Fleshner M. (2008). Exercise, learned helplessness, and the stress-resistant brain. Neuromolecular Med 10:81–98
- Greenwood BN, Fleshner M. (2011). Exercise, stress resistance, and central serotonergic systems. Exerc Sport Sci Rev 39:140–9
- Greenwood BN, Foley TE, Burhans D, Maier SF, Fleshner M. (2005a). The consequences of uncontrollable stress are sensitive to duration of prior wheel running. Brain Res 1033:164–78
- Greenwood BN, Foley TE, Day HE, Burhans D, Brooks L, Campeau S, Fleshner M. (2005b). Wheel running alters serotonin (5-HT) transporter, 5-HT1A, 5-HT1B, and alpha 1b-adrenergic receptor mRNA in the rat raphe nuclei. Biol Psychiatry 57:559–68
- Greenwood BN, Foley TE, Day HE, Campisi J, Hammack SH, Campeau S, Maier SF, Fleshner M. (2003a). Freewheel running prevents learned helplessness/behavioral depression: role of dorsal raphe serotonergic neurons. J Neurosci 23:2889–98
- Greenwood BN, Foley TE, Le TV, Strong PV, Loughridge AB, Day HE, Fleshner M. (2011). Long-term voluntary wheel running is rewarding and produces plasticity in the mesolimbic reward pathway. Behav Brain Res 217:354–62
- Greenwood BN, Kennedy S, Smith TP, Campeau S, Day HE, Fleshner M. (2003b). Voluntary freewheel running selectively modulates catecholamine content in peripheral tissue and c-Fos expression in the central sympathetic circuit following exposure to uncontrollable stress in rats. Neuroscience 120:269–81
- Greenwood BN, Loughridge AB, Sadaoui N, Christianson JP, Fleshner M. (2012a). The protective effects of voluntary exercise against the behavioral consequences of uncontrollable stress persist despite an increase in anxiety following forced cessation of exercise. Behav Brain Res 233:314–21
- Greenwood BN, Spence KG, Crevling DM, Clark PJ, Craig WC, Fleshner M. (2013). Exercise-induced stress resistance is independent of exercise controllability and the medial prefrontal cortex. Eur J Neurosci 37:469--78
- Greenwood BN, Strong PV, Fleshner M. (2010). Lesions of the basolateral amygdala reverse the long-lasting interference with shuttle box escape produced by uncontrollable stress. Behav Brain Res 211:71–6
- Greenwood BN, Strong PV, Foley TE, Fleshner M. (2009). A behavioral analysis of the impact of voluntary physical activity on hippocampus-dependent contextual conditioning. Hippocampus 19:988–1001
- Greenwood BN, Strong PV, Foley TE, Thompson RS, Fleshner M. (2007). Learned helplessness is independent of levels of brain-derived neurotrophic factor in the hippocampus. Neuroscience 144:1193–208
- Greenwood BN, Strong PV, Loughridge AB, Day HE, Clark PJ, Mika A, Hellwinkel JE, et al. (2012b). 5-HT(2C) receptors in the basolateral amygdala and dorsal striatum are a novel target for the anxiolytic and antidepressant effects of exercise. PLoS One 7:e46118
- Hale MW, Shekhar A, Lowry CA. (2012). Stress-related serotonergic systems: implications for symptomatology of anxiety and affective disorders. Cell Mol Neurobiol 32:695–708
- Hammack SE, Cooper MA, Lezak KR. (2012). Overlapping neurobiology of learned helplessness and conditioned defeat: implications for PTSD and mood disorders. Neuropharmacology 62:565–75
- Hammack SE, Richey KJ, Watkins LR, Maier SF. (2004). Chemical lesion of the bed nucleus of the stria terminalis blocks the behavioral consequences of uncontrollable stress. Behav Neurosci 118:443–8
- Hammack SE, Schmid MJ, LoPresti ML, Der-Avakian A, Pellymounter MA, Foster AC, Watkins LR, Maier SF. (2003). Corticotropin releasing hormone type 2 receptors in the dorsal raphe nucleus mediate the behavioral consequences of uncontrollable stress. J Neurosci 23:1019–25
- Harada K, Yamaji T, Matsuoka N. (2008). Activation of the serotonin 5-HT2C receptor is involved in the enhanced anxiety in rats after single-prolonged stress. Pharmacol Biochem Behav 89(1):11--16
- Harvey ML, Swallows CL, Cooper MA. (2012). A double dissociation in the effects of 5-HT2A and 5-HT2C receptors on the acquisition and expression of conditioned defeat in Syrian hamsters. Behav Neurosci 126:530–7
- Hauger RL, Risbrough V, Oakley RH, Olivares-Reyes JA, Dautzenberg FM. (2009). Role of CRF receptor signaling in stress vulnerability, anxiety, and depression. Ann N Y Acad Sci 1179:120–43
- Holmes A. (2008). Genetic variation in cortico-amygdala serotonin function and risk for stress-related disease. Neurosci Biobehav Rev 32:1293–314
- Holtzheimer PE, Mayberg HS. Deep brain stimulation for psychiatric disorders. (2011). Annu Rev Neurosci 34:289–307
- Insel T, Cuthbert B, Garvey M, Heinssen R, Pine DS, Quinn K, Sanislow C, Wang P. (2010). Research domain criteria (RDoC): toward a new classification framework for research on mental disorders. Am J Psychiatry 167:748–51
- Jackson RL, Alexander JH, Maier SF. (1980). Learned helplessness, inactivity, and associative deficits: effects of inescapable shock on response choice escape learning. J Exp Psychol Anim Behav Process 6:1–20
- Jackson RL, Minor TR. (1988). Effects of signaling inescapable shock on subsequent escape learning: implications for theories of coping and “learned helplessness”. J Exp Psychol Anim Behav Process 14:390–400
- Jankowski MP, Sesack SR. (2004). Prefrontal cortical projections to the rat dorsal raphe nucleus: ultrastructural features and associations with serotonin and gamma-aminobutyric acid neurons. J Comp Neurol 468:518–29
- Jovanovic T, Norrholm SD, Blanding NQ, Davis M, Duncan E, Bradley B, Ressler KJ. (2010). Impaired fear inhibition is a biomarker of PTSD but not depression. Depress Anxiety 27:244–51
- Jovanovic T, Ressler KJ. (2010). How the neurocircuitry and genetics of fear inhibition may inform our understanding of PTSD. Am J Psychiatry 167:648–62
- Kennett GA, Wood MD, Bright F, Trail B, Riley G, Holland V, Avenell KY, et al. (1997). SB 242084, a selective and brain penetrant 5-HT2C receptor antagonist. Neuropharmacology 36:609–20
- Kim JJ, Jung MW. (2006). Neural circuits and mechanisms involved in Pavlovian fear conditioning: a critical review. Neurosci Biobehav Rev 30:188–202
- Kubala KH, Christianson JP, Kaufman RD, Watkins LR, Maier SF. (2012). Short- and long-term consequences of stressor controllability in adolescent rats. Behav Brain Res 234:278–84
- Lanzenberger RR, Mitterhauser M, Spindelegger C, Wadsak W, Klein N, Mien LK, Holik A, et al. (2007). Reduced serotonin-1A receptor binding in social anxiety disorder. Biol Psychiatry 61:1081–9
- Lett BT, Grant VL, Byrne MJ, Koh MT. (2000). Pairings of a distinctive chamber with the aftereffect of wheel running produce conditioned place preference. Appetite 34:87–94
- Lyons DM, Macri S. (2011). Resilience and adaptive aspects of stress in neurobehavioral development. Neurosci Biobehav Rev 35:1451
- Maier SF. (1990). Role of fear in mediating shuttle escape learning deficit produced by inescapable shock. J Exp Psychol Anim Behav Process 16:137–49
- Maier SF. (2001). Exposure to the stressor environment prevents the temporal dissipation of behavioral depression/learned helplessness. Biol Psychiatry 49:763–73
- Maier SF, Amat J, Baratta MV, Paul E, Watkins LR. (2006). Behavioral control, the medial prefrontal cortex, and resilience. Dialogues Clin Neurosci 8:397–406
- Maier SF, Grahn RE, Kalman BA, Sutton LC, Wiertelak EP, Watkins LR. (1993). The role of the amygdala and dorsal raphe nucleus in mediating the behavioral consequences of inescapable shock. Behav Neurosci 107:377–88
- Maier SF, Grahn RE, Watkins LR. (1995). 8-OH-DPAT microinjected in the region of the dorsal raphe nucleus blocks and reverses the enhancement of fear conditioning and interference with escape produced by exposure to inescapable shock. Behav Neurosci 109:404–12
- Maier SF, Kalman BA, Grahn RE. (1994). Chlordiazepoxide microinjected into the region of the dorsal raphe nucleus eliminates the interference with escape responding produced by inescapable shock whether administered before inescapable shock or escape testing. Behav Neurosci 108:121–30
- Maier SF, Keith JR. (1987). Shock signals and the development of stress-induced analgesia. J Exp Psychol Anim Behav Process 13:226–38
- Maier SF, Seligman MEP. (1976). Learned helplessness: Theory and evidence. Journal of Experimental Psychology: General 105:3–46
- Maier SF, Watkins LR. (2005). Stressor controllability and learned helplessness: the roles of the dorsal raphe nucleus, serotonin, and corticotropin-releasing factor. Neurosci Biobehav Rev 29:829–41
- Maier SF, Watkins LR. (2010). Role of the medial prefrontal cortex in coping and resilience. Brain Res 1355:52--60
- Maswood S, Barter JE, Watkins LR, Maier SF. (1998). Exposure to inescapable but not escapable shock increases extracellular levels of 5-HT in the dorsal raphe nucleus of the rat. Brain Res 783:115–20
- Matos FF, Urban C, Yocca FD. (1996). Serotonin (5-HT) release in the dorsal raphe and ventral hippocampus: raphe control of somatodendritic and terminal 5-HT release. J Neural Transm 103:173–90
- McDonald AJ, Shammah-Lagnado SJ, Shi C, Davis M. (1999). Cortical afferents to the extended amygdala. Ann N Y Acad Sci 877:309–38
- Mehta D, Binder EB. (2012). Gene x environment vulnerability factors for PTSD: the HPA-axis. Neuropharmacology 62:654–62
- Millan MJ. (2005). Serotonin 5-HT2C receptors as a target for the treatment of depressive and anxious states: focus on novel therapeutic strategies. Therapie 60:441–60
- Mineka S, Cook M, Miller S. (1984). Fear conditioned with escapable and inescapable shock: effects of a feedback stimulus. J Exp Psychol Anim Behav Process 10:307--23
- Minor TR, Trauner MA, Lee CY, Dess NK. (1990). Modeling signal features of escape response: effects of cessation conditioning in “learned helplessness” paradigm. J Exp Psychol Anim Behav Process 16:123–36
- Mitchell ES, Sexton T, Neumaier JF. (2007). Increased expression of 5-HT6 receptors in the rat dorsomedial striatum impairs instrumental learning. Neuropsychopharmacology 32:1520–30
- Morrison KE, Bader LR, McLaughlin CN, Cooper MA. (2013). Defeat-induced activation of the ventral medial prefrontal cortex is necessary for resistance to conditioned defeat. Behav Brain Res 243:158--64
- Morrison KE, Cooper MA. (2012). A role for 5-HT1A receptors in the basolateral amygdala in the development of conditioned defeat in Syrian hamsters. Pharmacol Biochem Behav 100:592–600
- Morrison KE, Curry DW, Cooper MA. (2012). Social status alters defeat-induced neural activation in Syrian hamsters. Neuroscience 210:168--78
- Moya PR, Fox MA, Jensen CL, Laporte JL, French HT, Wendland JR, Murphy DL. (2011). Altered 5-HT2C receptor agonist-induced responses and 5-HT2C receptor RNA editing in the amygdala of serotonin transporter knockout mice. BMC Pharmacol 11:3
- Nash JR, Sargent PA, Rabiner EA, Hood SD, Argyropoulos SV, Potokar JP, Grasby PM, Nutt DJ. (2008). Serotonin 5-HT1A receptor binding in people with panic disorder: positron emission tomography study. Br J Psychiatry 193:229–34
- Neumeister A, Bain E, Nugent AC, Carson RE, Bonne O, Luckenbaugh DA, Eckelman W, et al. (2004). Reduced serotonin type 1A receptor binding in panic disorder. J Neurosci 24:589–91
- Overstreet DH, Knapp DJ, Angel RA, Navarro M, Breese GR. (2006). Reduction in repeated ethanol-withdrawal-induced anxiety-like behavior by site-selective injections of 5-HT1A and 5-HT2C ligands. Psychopharmacology (Berl) 187:1–12
- Overstreet DH, Knapp DJ, Moy SS, Breese GR. (2003). A 5-HT1A agonist and a 5-HT2c antagonist reduce social interaction deficit induced by multiple ethanol withdrawals in rats. Psychopharmacology (Berl) 167:344–52
- Parker KJ, Maestripieri D. (2011). Identifying key features of early stressful experiences that produce stress vulnerability and resilience in primates. Neurosci Biobehav Rev 35:1466–83
- Paulus MP, Stein MB. (2010). Interoception in anxiety and depression. Brain Struct Funct 214:451–63
- Quirk GJ, Beer JS. (2006). Prefrontal involvement in the regulation of emotion: convergence of rat and human studies. Curr Opin Neurobiol 16:723–7
- Ravinder S, Burghardt NS, Brodsky R, Bauer EP, Chattarji S. (2013). A role for the extended amygdala in the fear-enhancing effects of acute selective serotonin reuptake inhibitor treatment. Transl Psychiatry 3:e209
- Remple MS, Henry EC, Catania KC. (2003). Organization of somatosensory cortex in the laboratory rat (Rattus norvegicus): Evidence for two lateral areas joined at the representation of the teeth. J Comp Neurol 467:105–18
- Rescorla RA. (1969). Pavlovian conditioned inhibtion. Psychol Bull 72:77–94
- Rodgers KM, Benison AM, Klein A, Barth DS. (2008). Auditory, somatosensory, and multisensory insular cortex in the rat. Cereb Cortex 18:2941–51
- Rozeske RR, Der-Avakian A, Bland ST, Beckley JT, Watkins LR, Maier SF. (2009). The medial prefrontal cortex regulates the differential expression of morphine-conditioned place preference following a single exposure to controllable or uncontrollable stress. Neuropsychopharmacology 34:834–43
- Rozeske RR, Der-Avakian A, Watkins LR, Maier SF. (2012). Activation of the medial prefrontal cortex by escapable stress is necessary for protection against subsequent inescapable stress-induced potentiation of morphine conditioned place preference. Eur J Neurosci 35:160–5
- Rozeske RR, Evans AK, Frank MG, Watkins LR, Lowry CA, Maier SF. (2011a). Uncontrollable, but not controllable, stress desensitizes 5-HT1A receptors in the dorsal raphe nucleus. J Neurosci 31:14107–15
- Rozeske RR, Greenwood BN, Fleshner M, Watkins LR, Maier SF. (2011b). Voluntary wheel running produces resistance to inescapable stress-induced potentiation of morphine conditioned place preference. Behav Brain Res 219:378–81
- Russo SJ, Murrough JW, Han MH, Charney DS, Nestler EJ. (2012). Neurobiology of resilience. Nat Neurosci 15:1475–84
- Sato F, Akhter F, Haque T, Kato T, Takeda R, Nagase Y, Sessle BJ, Yoshida A. (2012). Projections from the insular cortex to pain-receptive trigeminal caudal subnucleus (medullary dorsal horn) and other lower brainstem areas in rats. Neuroscience 233C:9–27
- Scharf SH, Schmidt MV. (2012). Animal models of stress vulnerability and resilience in translational research. Curr Psychiatry Rep 14:159–65
- Schmidt MV, Sterlemann V, Muller MB. (2008). Chronic stress and individual vulnerability. Ann N Y Acad Sci 1148:174–83
- Sciolino NR, Holmes PV. (2012). Exercise offers anxiolytic potential: a role for stress and brain noradrenergic-galaninergic mechanisms. Neurosci Biobehav Rev 36:1965–84
- Seligman ME, Maier SF. (1967). Failure to escape traumatic shock. J Exp Psychol 74:1–9
- Shi CJ, Cassell MD. (1998a). Cascade projections from somatosensory cortex to the rat basolateral amygdala via the parietal insular cortex. J Comp Neurol 399:469–91
- Shi CJ, Cassell MD. (1998b). Cortical, thalamic, and amygdaloid connections of the anterior and posterior insular cortices. J Comp Neurol 399:440–68
- Short KR, Maier SF. (1993). Stressor controllability, social interaction, and benzodiazepine systems. Pharmacol Biochem Behav 45:827–35
- Southwick SM, Charney DS. (2012). The science of resilience: implications for the prevention and treatment of depression. Science 338:79–82
- Southwick SM, Vythilingam M, Charney DS. (2005). The psychobiology of depression and resilience to stress: implications for prevention and treatment. Annu Rev Clin Psychol 1:255–91
- Spinelli S, Chefer S, Carson RE, Jagoda E, Lang L, Heilig M, Barr CS, et al. (2010). Effects of early-life stress on serotonin(1A) receptors in juvenile Rhesus monkeys measured by positron emission tomography. Biol Psychiatry 67:1146–53
- Sprouse JS, Aghajanian GK. (1987). Electrophysiological responses of serotoninergic dorsal raphe neurons to 5-HT1A and 5-HT1B agonists. Synapse 1:3–9
- Stevens HE, Leckman JF, Coplan JD, Suomi SJ. (2009). Risk and resilience: early manipulation of macaque social experience and persistent behavioral and neurophysiological outcomes. J Am Acad Child Adolesc Psychiatry 48:114–27
- Stiller AL, Drugan RC, Hazi A, Kent SP. (2011). Stress resilience and vulnerability: the association with rearing conditions, endocrine function, immunology, and anxious behavior. Psychoneuroendocrinology 36:1383–95
- Strong PV, Christianson JP, Loughridge AB, Amat J, Maier SF, Fleshner M, Greenwood BN. (2011). 5-hydroxytryptamine 2C receptors in the dorsal striatum mediate stress-induced interference with negatively reinforced instrumental escape behavior. Neuroscience 197:132–44
- Strong PV, Greenwood BN, Fleshner M. (2009). The effects of the selective 5-HT(2C) receptor antagonist SB 242084 on learned helplessness in male Fischer 344 rats. Psychopharmacology (Berl) 203:665–75
- Suomi SJ. (2006). Risk, resilience, and gene x environment interactions in rhesus monkeys. Ann N Y Acad Sci 1094:52–62
- Tanaka SC, Shishida K, Schweighofer N, Okamoto Y, Yamawaki S, Doya K. (2009). Serotonin affects association of aversive outcomes to past actions. J Neurosci 29:15669–74
- Varela JA, Wang J, Christianson JP, Maier SF, Cooper DC. (2012). Control over stress, but not stress per se increases prefrontal cortical pyramidal neuron excitability. J Neurosci 32:12848–53
- Vaynman S, Ying Z, Gomez-Pinilla F. (2004). Hippocampal BDNF mediates the efficacy of exercise on synaptic plasticity and cognition. Eur J Neurosci 20:2580–90
- Vertes RP. (2004). Differential projections of the infralimbic and prelimbic cortex in the rat. Synapse 51:32–58
- Weiss JM. (1971). Effects of coping behavior with and without a feedback signal on stress pathology in rats. J Comp Physiol Psychol 77:22–30
- Will MJ, Watkins LR, Maier SF. (1998). Uncontrollable stress potentiates morphine’s rewarding properties. Pharmacol Biochem Behav 60:655–64
- Yin HH, Knowlton BJ. (2006). The role of the basal ganglia in habit formation. Nat Rev Neurosci 7:464–76