Abstract
Patients with post-traumatic stress disorder (PTSD) exhibit exaggerated daytime muscle tension as well as nocturnal sleep disturbances. Yet, these physiological and behavioral features of the disorder are little studied in animal models of PTSD. Accordingly, the present studies were designed to assess alterations in muscle tension and diurnal hyper-vigilance resulting from exposure to a social defeat stressor paired with an olfactory stimulus, which was then used as a reminder of stressor exposure. In the first series of experiments, rats presented with an olfactory cue paired previously with a single social defeat exhibited a significant increase in muscle tension 4 weeks following defeat. In the second series of experiments, an olfactory cue paired previously with a single social defeat induced a significant increase in locomotor activity among quiescent rats 4 weeks following stressor exposure. The present results thus support the a priori hypotheses that novel physiological and behavioral hallmarks of PTSD can be documented in an animal model of the disorder and that the present overt signs of reactive hyper-vigilance can be triggered by reintroduction of an olfactory stimulus present at the time of initial trauma exposure.
Introduction
Heightened muscle tension and abnormal sleep patterns are recognized symptoms of post-traumatic stress disorder (PTSD). In particular, electromyographic measurements are elevated by exposure to affective stimuli in combat veterans with PTSD relative to controls (Keane et al. Citation1998; Morgan et al. Citation1998). Specifically, forehead and facial muscle electromyography differentiates between PTSD and control groups and is hypothesized to reflect a coping response in the expression of affect (Casada et al. Citation1998). This heightened muscle tension provides evidence of conscious hyperarousal in patients with PTSD and is consistent with complementary research identifying heightened stimulus reactivity during sleep in the PTSD population (Lavie et al. Citation1998). Notably, PTSD patients report subjective sleep disturbances including fragmented sleep and repeated nightmares which replay traumatic events (American Psychiatric Association Citation2000) even though objective polysomnographic measures of sleep reveal few differences in the sleep architecture of PTSD patients versus comparison groups (Lavie et al. Citation1998). Moreover, PTSD patients exhibit significantly higher awakening thresholds which quantify depth of sleep when the sleeper is exposed to arousing stimuli of increasing intensity (Lavie Citation2001). It can be suggested that diurnal muscle tension and nocturnal awakening thresholds covary with clinical pathology in PTSD patients. As these physiological and behavioral dependent measures of pathological arousal have not been extensively and exhaustively characterized in an animal model of PTSD, the main goal of the present studies was to record muscle tension and diurnal stimulus reactivity in trauma-exposed rats.
Specific smells circumstantially related to a traumatic event precipitate reoccurrence of intrusive, distressing memories and flashbacks in PTSD patients (Charney et al. Citation1993). This Pavlovian fear response is the foundation for basic research associating odor cues and stressor exposure in an animal model of conditioned freezing (Otto et al. Citation2000) whereby freezing to the odor cue does not decline significantly when rats are tested 17–31 days following fear conditioning (Otto et al. Citation1997). These results suggest that a conditioned odor and an unconditioned noxious stimulus can be linked in order to model the phenomenon whereby specific smells trigger panic attacks in PTSD sufferers (King et al. Citation2001). Accordingly, the present studies utilized both social defeat by an aggressive male rat as a discrete traumatic experience and a social defeat-conditioned odor cue as a recurrent reminder of the trauma over an extended delay of 1 month. Heightened sensitivity to the acquired aversive and arousing properties of the conditioned odor cue was assessed using defensive burying, muscle tension and diurnal stimulus reactivity dependent measures in normal rats.
Materials and methods
Animals
Male Wistar rats (n = 28, Charles River Kingston), 3–5 months of age, were housed individually in a temperature and light cycle-controlled vivarium with ad libitum access to water and rodent chow (Harlan Teklad) and allowed at least seven acclimatization days prior to any experimental manipulations. The sleep/wake cycle was reversed so that the dark cycle (active, waking hours termed the “nocturnal” photoperiod) elapsed from 1000 to 2200 h when the level of human activity in the vivarium was high, and the light cycle (inactive or “diurnal” photoperiod, lights on 2200–1000 h) elapsed when human activity in the vivarium was minimized, an important safeguard when attempting to study undisturbed, sleeping, and/or resting animals. Accordingly, all nocturnal photoperiod studies were conducted under red light and video recordings were performed using an infrared camera capable of capturing images without visible illumination. Separate pairs of male and female Wistar rats were bred in the colony to provide male residents for social defeat implementation. Testing protocols were approved by the Institutional Animal Care and Use Committee of Boston College and carried out in accordance with the National Institute of Health Guide for the Care and Use of Laboratory Animals.
Experiment 1—Impact of odor conditioning and social defeat on muscle tension, telemetered movement, circadian locomotor activity, and defensive burying
Radiotelemetry
In order to assess physiological activation as a consequence of prior exposure to social defeat, rats were equipped with telemetry implants capable of registering skeletal muscle tension. Rats were anesthetized with isoflurane (2–5% in oxygen) and placed on a heating pad throughout the duration of transponder implantation (TL11M2-C50-PXT; Data Sciences, St Paul, MN, USA). Two biopotential leads were inserted subcutaneously and attached to the left gastrocnemius muscle (Heinrichs Citation2003). The sender body was implanted into the abdomen and sutured to the abdominal wall. Animals were allowed a 1-week post-operative recovery prior to any experimental data acquisition.
Muscle tension is an activity-independent measure of muscle tone in hind limb gastrocnemius fibers (Heinrichs Citation2003) and this was confirmed in the present studies by concurrent measurement of movement of the implanted transmitter in 3D space overlying the receiving platform (quantified as “telemetered movement”). A data acquisition computer was utilized to collect and store biotelemetry data as a waveform over a 10 s period without disruption to the rat. Muscle tension was measured three times at 10-min intervals for 30 min in undisturbed rats in their home cage (1300–1700), whereas telemetered movement was measured over the entire 30-min interval. Muscle tension and movement were measured during the baseline week and weeks 1, 2, and 4 immediately following odor cue exposure.
Social defeat and initial odor conditioning
Aggressive interaction with a male conspecific was employed as a species-specific traumatic stressor which is known to produce sleep fragmentation (Kinn et al. Citation2008). Pairs of male and female rats, termed “residents”, were housed together for several weeks before the start of the experiment until the male rat reliably established reproductive and territorial behaviors (Heinrichs et al. Citation1992); resident rats were also prescreened for aggression by completing at least two successful defeats of a separate group of rats. Social defeat animals were exposed to an aggressive resident rat once on the first day of experimental week 1 (1300–1700 h). Upon being placed in the resident's cage, the experimental rat began sniffing and engaging the resident rat. After this brief period, the resident rat became antagonistic for the remainder of the social defeat, culminating with a physical attack on the intruder. Specifically, within a period of 20–120 s of intruder placement, the resident rat threatened, chased, and pinned the intruder in a defensive supine posture (spine against the cage floor with head and limbs oriented toward the resident rat), the measure of social defeat, at which point the investigators withdrew the intruder, placing it back in its home cage. This type of social defeat consists of a physical attack, without a longer “threat of attack” period and has been used successfully in our laboratory as an effective stressor. Defeat latency was measured from the time the experimental animal was placed in the resident cage until the intruder assumed a defensive supine position and importantly, average defeat latencies (about 90 s) did not vary across social defeat cohorts in the present studies (data not shown).
Concurrent with social defeat, a novel odor cue, specifically the aroma given off when liquid peppermint or coconut extracts (McCormick-Schilling) evaporate into the air, was used as a neutral olfactory stimulus paired with the social defeat for the purpose of subsequently re-exposing rats to a conditioned odor cue as a reminder of prior trauma (see olfactory exposure/reminding and defensive burying task). Specifically, a cotton swab was dipped into the odored extract and then wiped across the resident rat's back immediately prior to social defeat of experimental rats. Odor detectability by experimental rats was suggested by the fact that testing rooms were pungent with the odor as judged by human experimenters and by the fact that intruder rats were observed to actively investigate the resident rat's back at the beginning of the social interaction.
Locomotor activity
Two-dimensional locomotor activity was recorded using a computer-automated array of four infrared photo beams oriented along the horizontal axis of a grid exterior to the home cage (Cage Rack Activity System, San Diego Instruments, San Diego, CA, USA). Locomotor activity was measured in a subset of rats as a general index of overall activity during the baseline week and weeks 1, 2, and 4 immediately following odor cue exposure. Circadian activity patterns in the home cage were examined by continuously recording locomotor activity over the entire 1-month study period.
Olfactory exposure/reminding and defensive burying task
For olfactory exposure/reminding, rats were first transported to a testing room separate from the colony room. In order to re-expose rats to the odor, a 0.1 ml volume of odored extract was squirted onto cotton gauze pads that were placed into a hollow, ventilated cylindrical container (the odor vesicle) which maintained odor exposure but prevented the odor-impregnated cotton from coming into contact with the rat. All rats were exposed to the odor cue for 15 min by placing the odor vesicle in the geometric center of the home cage floor (1300–1700 h); this served as a neutral cue for control rats and as a reminder of trauma for socially-defeated rats. In order to document that the odor cue paired with social defeat acquired motivational value as a conditioned stimulus, a defensive burying task was employed to assess the behavior of a rat presented with an aversive stimulus (Reynolds and Berridge Citation2002). Behavior was videotaped for 10 min and scored subsequently by a rater blind to experimental treatments. Frequency of vesicle burying, latency to initial contact with the vesicle, and number of explorations of the vesicle served as measures of defensive burying and object interaction. In separate validation experiments examining the specificity of the aversively conditioned odor cue to induce defensive burying, vesicles containing unconditioned, novel odors were never buried by either control (zero of eight rats) or stressed (zero of eight rats) groups. Accordingly only vesicles containing conditioned odor cues were employed in the present experiments. Home cage bedding was changed after the test to ensure that no vestige of the odor was transmitted back into the colony room.
Telemetered rats were first tested during a baseline week for muscle tension and telemetered movement (). At the beginning of week 1, rats were randomly assigned to one of two conditions, control (n = 4) or social defeat (n = 4). Rats in the control group remained in the home cage, were exposed to olfactory reminder cues, but were not exposed to social defeat. Rats in the social defeat condition were exposed on the first day of week 1 to an aggressive resident rat as described previously. Muscle tension and telemetered movement were assessed during weeks 1, 2, and 4 immediately following conditioned odor presentation. In Experiment 1, the behavior of animals during olfactory reminding was only recorded during week 4 (). A separate group of non-telemetered rats (n = 8) was tested using the same protocol with circadian locomotor activity and defensive burying as dependent measures ().
Table I. Experimental timeline.
Experiment 2—Impact of odor conditioning and social defeat on diurnal stimulus reactivity, circadian locomotor activity, and defensive burying
On three non-consecutive nights (i.e., after 2200 h) each testing week, the diurnal odor and tone reactivity of rats were tested by monitoring home cage locomotor activity in the context of presentations of conditioned odor and unconditioned tone stimuli of progressively increasing intensity. The data acquisition and control computer was located in the room adjacent to the colony room, allowing the experimenter to monitor quiescent rats without detection. All tests were completed between 2215 and 2245 h, during the light portion of the circadian cycle when rats ordinarily sleep, and approximately 30 min following the onset of the diurnal portion of the circadian cycle. On Day 1 of each testing week, a control reactivity test was performed. For this test, the experimenter entered the room adjacent to the colony and set the computer to record beam breaks every 15 s for a 10-min period. The other two diurnal reactivity tests, odor and tone, were performed over 10 min either 2 or 4 days following the weekly control reactivity tests in a counterbalanced fashion. For the odor test, the experimenter divided the width of the room adjacent to the colony into 10 equal lengths so that the odor vesicle could be moved incrementally, one step closer for each elapsed minute of the test, toward a portal in the wall through which air flowed into the colony room. At the conclusion of this procedure, the colony room was plainly scented with the odor cue. For the tone test, the experimenter used a digital metronome (Dr Beat) that was located in the adjacent room and was connected to speakers in the colony room. During the test, a pure tone (440 Hz) was sounded for 3 s per minute, and the intensity level (gain knob settings 1–10 resulting in sound pressure levels of 50, 51, 53, 55, 57, 60, 62, 64, 66, and 68 dB, respectively, 1 m from the source) was increased by one increment per minute. At the highest sound intensity, the tone was plainly audible in the colony room. In each of these tests, control, cue, and odor, stimulus reactivity was detected by an increase in locomotor activity at the time of stimulus presentation.
Each rat was first tested during a baseline week for control, tone, and odor reactivity and continuously for circadian activity (). At the beginning of week 1, rats were randomly assigned to one of two conditions, control (n = 6) or social defeat (n = 6). Rats in the control group remained in the home cage, were exposed to olfactory reminder cues as described in Experiment 1, but were not exposed to social defeat. Rats in the social defeat condition were exposed on the first day of week 1 to an aggressive resident rat as described previously. Stimulus reactivity was assessed during weeks 1, 2, and 4 during the diurnal phase of the photocycle whereas circadian activity was assessed continuously over the same period ().
Data analysis
Two- and three-way fixed factor analyses of variance (ANOVA) were used to examine data. The between-subjects variable was social defeat exposure. The within-subjects variables were time (hour and day) and stimulus cue (control, tone, and odor). Significant overall ANOVAs were followed by post-hoc Bonferroni and Scheffé tests to explore individual group differences. For the defensive burying frequency measure, nominal frequency data were analyzed using a non-parametric Chi-square test. For all analyses, significance was set at p < 0.05.
Results
Experiment 1—Muscle tension
ANOVA revealed significant main effects of time [F(3,18) = 3.2, p < 0.05] and experimental treatment [F(1,6) = 6.1, p < 0.05] on the muscle tension measure. In addition, the time by experimental treatment interaction was also significant [F(3,18) = 3.7, p < 0.05] due in part to the finding that hind limb muscle tension in the socially-defeated animals was consistently greater () than controls over the final 3 weeks of testing, significantly so in week 4 (Scheffé test, p < 0.05). In particular, no muscle tension value in the untreated control group exceeded 15 μV (zero of four rats) whereas all rats in the social defeat group exceeded 30 μV (four of four) at some point during the 4-week testing period.
Figure 1 Exposure to a social defeat stressor augments hind limb muscle tension. Muscle tension (μV; mean+SEM) was recorded radiotelemetrically before the beginning of experimental manipulations (baseline) and 1, 2, or 4 weeks afterward (n = 4/group). Rats were socially defeated prior to the week 1 muscle tension test and the social defeat-paired odor cue was presented prior to the week 2 and 4 tests. *p < 0.05 relative to unstressed controls.
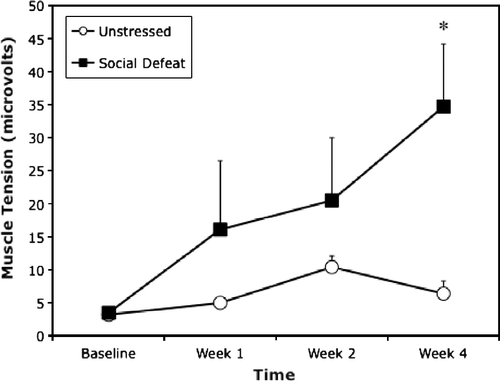
As expected, home cage locomotor activity measured in the vivarium animal colony did vary significantly [F(23,139) = 8.4, p < 0.0001] with time of day as rats exhibited low levels of diurnal activity and high levels of nocturnal activity on a daily basis (data not shown). However, no main [Treatment − F(1,6) = 2.3, n.s.] or interaction [Time × Treatment − F(6,36) = 2.2, n.s.] effects of social defeat on telemetered movement were detected (data not shown). Thus, increased muscle tension was not accompanied by detectable increases in spatial locomotor activity. This suggests that the present social defeat manipulation increased movements-in-place or neuromuscular “fidgeting” as has been reported previously (Heinrichs Citation2003).
Experiment 2—Diurnal stimulus reactivity
ANOVA revealed a significant main effect of stimulus cue on the locomotor reactivity measure, as determined by an increase in locomotor activity coincident with the stimulus cue [F(2,12) = 11.0, p < 0.005]. This main effect reflects the finding that the odor cue stimulated rats more effectively than either the control or tone condition (). There was also a significant interaction of the stimulus cue and testing phase on the locomotor reactivity measure [F(6,36) = 11.5, p < 0.05]. This resulted partly from the finding that stimulus reactivity occurred significantly (Bonferroni test, p < 0.01) more often in the social defeat group presented with the odor cue during week 4 testing (). Latency to first locomotor activity following presentation of the odor cue was shorter in socially-defeated compared to control rats (2 ± 4 vs. 8 ± 4 s, respectively), although this difference was not significant. Note that motor activity of social defeated rats following odor cue presentation differed significantly only from unstressed controls, but neither from defeated rats under control conditions, nor from control rats exposed to odor cues. These results suggest that pairing of a social defeat stressor and a neutral odor cue produced reactive hyper-vigilance to the aversive odor cue 4 weeks following conditioning.
Figure 2 Exposure to a social defeat stressor enhances odor cue-induced locomotor reactivity. Cumulative motor activity (locomotor counts per 10 min; mean+SEM) measured in the absence of stimulation (control) or following cue presentation (tone or odor) prior to social defeat (baseline week) and at weeks 1, 2, and 4 in unstressed controls rats or rats exposed to social defeat prior to the week 1 awakening test (n = 6/group). *p < 0.05 relative to unstressed controls.
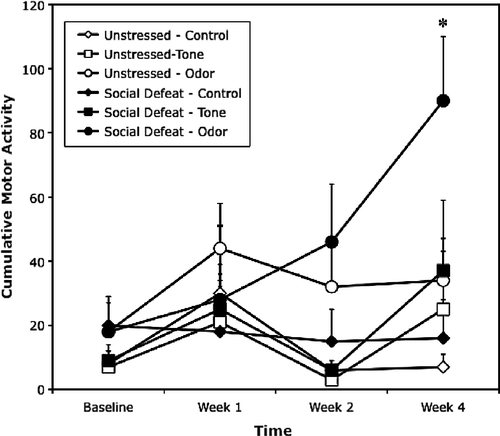
Paralleling the behavior observed in rats from Experiment 1, locomotor activity measured in the animal colony did vary significantly [F(23,230) = 93.5, p < 0.0001] with time of day as rats exhibited low levels of diurnal activity and high levels of nocturnal activity (data not shown). However, no main [Treatment − F(1,10) < 1, n.s.] or interaction [Day × Treatment − F(6,60) = 1.5, n.s.; Hour × Treatment − F(23,230) = 1.1, n.s.; Day × Hour × Treatment − F(138,1380) = 1.0, n.s.] effects of social defeat on overall locomotor activity were detected (data not shown). Thus, there was no evidence that experimental treatments produced changes in overall levels of activity/inactivity or shifted the phase of activity/inactivity over time. This finding suggests that the social defeat stressor did not alter diurnal stimulus reactivity as a consequence of sleep/wake cycle dysregulation.
Experiments 1 and 2—Defensive burying
There was a significant [χ2(1) = 7.8, p < 0.005] effect of experimental treatment on the frequency of odor vesicle burying (). In particular, when odor vesicle burying was summed across weeks 1, 2, and 4, the social defeat group was more likely to bury relative to the control group. This finding was consistent with the observation that socially defeated rats interacted actively with the olfactory vesicle, by frequently moving it into the corner and burying it under wood chip bedding. The latency to vesicle contact and frequency of vesicle exploration measures were not impacted significantly by experimental treatment ().
Table II. Impact of social defeat on frequency of odor vesicle burying, latency to first contact, and number of explorations.
Discussion
The muscle tension dependent measure provided support for the first experimental hypothesis that trauma exposure would lead to a persistent state of hyper-vigilance. Hind limb muscle tension, studied in the present experiments because muscle groups involved in hind limb motion are necessary for mounting “fight or flight” coping responses of rats exposed to traumatic stimuli, was found to increase progressively and significantly over three post-trauma testing periods in the social defeat group. This augmented reactivity to an aversively conditioned odor cue in the present animal model is analogous to the affective coping response which is hypothesized to underlie heightened muscle tension exhibited by patients with PTSD (Casada et al. Citation1998). The present study also yielded a significant finding related to diurnal stimulus reactivity in which the social defeat group exhibited more locomotor activity than non-stressed controls when exposed to the noxiously conditioned odor cue, but not a neutral tone cue. This suggests that the watchfulness of odor-conditioned animals was altered in such a way that they were more sensitive to salient environmental stimuli. Comparable animal studies have documented the impact of stress on physiological arousal and sleep patterns (Lancel et al. Citation2002; Kinn et al. Citation2008; Pawlyk et al. Citation2008). For instance, one study showed that electric foot shock applied just prior to the onset of the light phase of the circadian cycle decreased wakefulness and increased rapid-eye-movement and slow-wave sleep (Vazquez-Palacios and Velazquez-Moctezuma Citation2000). Consistent with this result, exposure to situational reminders of the aversive training context is reported to disrupt sleep architecture in rats (Pawlyk et al. Citation2005). The present study extends these findings of stress-induced sleep fragmentation exerted during the active circadian period into the quiescent circadian period when patients with PTSD are reportedly vulnerable to nightmare-related awakening (Lavie et al. Citation1998). Taken together, the present results are consistent with published findings demonstrating that exposure to a single stressor is capable of eliciting signs of behavioral and physiological arousal which constitute an animal model of PTSD (Cohen et al. Citation2003).
The present results strongly support the second a priori hypothesis that the conditioned odor cue would be instrumental in eliciting long-term hyper-vigilance. In particular, the defensive burying test (Treit et al. Citation1981; De Boer and Koolhaas Citation2003) yielded a statistically significant effect of social defeat by increasing the frequency of odor vesicle burial. The act of burying the vesicle entailed the rat either pawing or using its head to thrust bedding on top of and/or around the vesicle (Pinel and Treit Citation1983). The finding that unconditioned, novel odors/vesicles were not buried suggests that this behavior does not occur spontaneously. Instead, the greater likelihood of burial in the social defeat group provides evidence that the odor cue previously paired with social defeat constitutes an anxiogenic-like stimulus (Heinrichs et al. Citation2002) which retained efficacy in the present studies by producing conditioned avoidance over a 4 week training–retention interval. The present findings are consistent with a report demonstrating an increase in defensive burying of an object paired with noxious shock, an outcome which reflects the hyper-vigilance characteristic of PTSD (Mikics et al. Citation2008).
The main effects of exposure to social defeat in the present studies cannot be easily attributed to non-specific effects of the trauma condition on overall locomotor activity. The circadian data yielded a significant effect of time that reflected the normal and expected periodicity, but no main or interaction effects involving the social defeat manipulation. This suggests that rats exposed previously to social defeat did not exhibit an elevated level of locomotor activity in their respective home cages relative to untreated controls. Consistent with the present dissociation of social defeat-induced vigilant and arousal-like responses from non-specific increases in motor behavior, several authors have argued that signs of hyperarousal can arise in both animal models of PTSD (Adamec Citation1997) and PTSD patients (Dagan et al. Citation1997) without changes in exploratory behavior or overall motor activity. Similarly and specifically relevant to the diurnal stimulus reactivity studies of Experiment 2, actigraph measures failed to corroborate sleep complaints (Klein et al. Citation2003) or sleep quality index scores (Calhoun et al. Citation2007) registered by PTSD patients. Thus, it can be suggested that home cage photocell activity is either insensitive to trauma exposure of the social defeat type and intensity employed in the present experiments or, alternatively, is governed by separate and independent skeletal movement systems from those which mediate the affective response to stressor exposure (Heinrichs Citation2003).
The incubation period of several weeks during which significant behavioral and physiological consequences of social defeat exposure developed is a noteworthy and interesting aspect of the present findings. In particular, the muscle tension measure, while increasing monotonically over intermediate weeks, was not significantly elevated until 4 weeks following social defeat. Such incubation effects are hypothesized to reflect an enhancement of memory strength for conditioned cues associated with stressor exposure (Madan et al. Citation2008). More strikingly, the effect of social defeat on enhancing conditioned stimulus reactivity was not revealed until 4 weeks following social defeat and there was no hint of the development of significant hyperreactivity to the odor cue at any prior time. These findings are consistent with the timecourse of PTSD onset in which sleep complaints reported at 4 weeks post-trauma are a useful index of vulnerability to suffer from the disorder, whereas the magnitude of sleep disturbances in those diagnosed with PTSD is amplified over 12 weeks post-trauma (Koren et al. Citation2002). Taken together, these data suggest that signs of hyper-vigilance are manifested beginning 4 weeks after exposure to an aversive stimulus or trauma in both rodent and human species (Shalev et al. Citation2000).
One limitation of the present studies is that increased diurnal arousal was not quantified directly via electroencephalographic recording, but rather inferred from an indirect measure of motor activity. While activity monitoring is face valid relative to the actigraphic method of quantifying sleep quality in a natural clinical setting (Klein et al. Citation2003), the present dependent measure is ill-equipped to distinguish genuine awakenings from resting-to-active transitions which conscious rats may have performed at the exact moment of home cage activity recording. Future studies could more effectively address this ambiguity by employing an electrophysiological determination of sleep stage. A second limitation of the present studies is that the entire social defeat exposed group of animals was employed as the study population in spite of the finding that PTSD affects only a fraction, perhaps 20–30%, of exposed individuals (Cohen et al. Citation2003). This incongruity can be addressed in future studies by taking advantage of the heterogeneity of the stress response in animals which can be divided into distinct groups according to the magnitude of response (Cohen et al. Citation2003).
Based upon the results of this study, it can be proposed that links exist between trauma experience, skeletal muscle tension, and hyper-vigilance. In particular, one can integrate the muscle tension and diurnal stimulus reactivity results to suggest that automatic motor behaviors may result in abnormal sensitivity during the entire photoperiod. Two series of studies favor this view based upon data that elevated awakening thresholds occur in the absence of an objectively measured alteration in sleep architecture (Lavie et al. Citation1998) and that fear conditioning in rats promotes leg and neck muscle twitches during sleep (Madan et al. Citation2008). The essential idea is that heightened muscle tone may serve as an allostatic load (McEwen Citation2000) for the unconscious organism which shifts physiological processes toward increased vigilance; this in turn creates a heightened sensitivity to otherwise innocuous exteroceptive stimuli such as the conditioned odor cue employed in the present studies. Hence, one countermeasure for abnormal stimulus reactivity that could be tested in future studies would be to attempt to normalize heightened sympathetic nervous system activity (Bonne et al. Citation2004; Charney Citation2004) that impacts skeletal muscles as a means of attenuating hyper-vigilance.
Acknowledgements
A. M. Nelson and K. S. DeMartini contributed equally to the conception and implementation of this project. We thank Chris Eswar, Melanie Leussis, Peter DeMartini, and Steve Dunlap for experimental assistance and encouragement.
Declaration of interest: The authors report no conflicts of interest. The authors alone are responsible for the content and writing of the paper.
References
- Adamec R. 1997. Transmitter systems involved in neural plasticity underlying increased anxiety and defense—Implications for understanding anxiety following traumatic stress. Neurosci Biobehav Rev. 21 6: 755–765.
- American Psychiatric Association. 2000. Diagnostic and statistical manual of mental disorders DSM-IV-TR. Washington, DC: American Psychiatric Association.
- Bonne O, Grillon C, Vythilingam M, Neumeister A, Charney. 2004. Adaptive and maladaptive psychobiological responses to severe psychological stress: Implications for the discovery of novel pharmacotherapy. Neurosci Biobehav Rev. 28 1: 65–94.
- Calhoun PS, Wiley M, Dennis MF, Means MK, Edinger JD, Beckham JC. 2007. Objective evidence of sleep disturbance in women with posttraumatic stress disorder. J Trauma Stress. 20 6: 1009–1018.
- Casada JH, Amdur R, Larsen R, Liberzon I. 1998. Psychophysiologic responsivity in posttraumatic stress disorder: Generalized hyperresponsiveness versus trauma specificity. Biol Psychiatry. 44 10: 1037–1044.
- Charney DS. 2004. Psychobiological mechanisms of resilience and vulnerability: Implications for successful adaptation to extreme stress. Am J Psychiatry. 161 2: 195–216.
- Charney DS, Deutch AY, Krystal JH, Southwick SM, Davis M. 1993. Psychobiologic mechanisms of posttraumatic stress disorder. Arch Gen Psychiatry. 50 4: 295–305.
- Cohen H, Zohar J, Matar M. 2003. The relevance of differential response to trauma in an animal model of posttraumatic stress disorder. Biol Psychiatry. 53 6: 463–473.
- Dagan Y, Zinger Y, Lavie P. 1997. Actigraphic sleep monitoring in posttraumatic stress disorder (PTSD) patients. J Psychosom Res. 42 6: 577–581.
- De Boer SF, Koolhaas JM. 2003. Defensive burying in rodents: Ethology, neurobiology and psychopharmacology. Eur J Pharmacol. 463 1–3: 145–161.
- Heinrichs SC. 2003. Nonexercise muscle tension and behavioral fidgeting are positively correlated with food availability/palatability and body weight in rats. Physiol Behav. 79 2: 199–207.
- Heinrichs SC, Pich EM, Miczek KA, Britton KT, Koob GF. 1992. Corticotropin-releasing factor antagonist reduces emotionality in socially defeated rats via direct neurotropic action. Brain Res. 581 2: 190–197.
- Heinrichs SC, De Souza EB, Schulteis G, Lapsansky JL, Grigoriadis DE. 2002. Brain penetrance, receptor occupancy and antistress in vivo efficacy of a small molecule corticotropin releasing factor type I receptor selective antagonist. Neuropsychopharmacology. 27 2: 194–202.
- Keane TM, Kolb LC, Kaloupek DG, Orr SP, Blanchard EB, Thomas RG, Hsieh FY, Lavori PW. 1998. Utility of psychophysiological measurement in the diagnosis of posttraumatic stress disorder: Results from a Department of Veterans Affairs Cooperative Study. J Consult Clin Psychol. 66 6: 914–923.
- King JA, Abend S, Edwards E. 2001. Genetic predisposition and the development of posttraumatic stress disorder in an animal model. Biol Psychiatry. 50 4: 231–237.
- Kinn AM, Gronli J, Fiske E, Kuipers S, Ursin R, Murison R, Portas CM. 2008. A double exposure to social defeat induces sub-chronic effects on sleep and open field behaviour in rats. Physiol Behav. 95 4: 553–561.
- Klein E, Koren D, Arnon I, Lavie P. 2003. Sleep complaints are not corroborated by objective sleep measures in post-traumatic stress disorder: A 1-year prospective study in survivors of motor vehicle crashes. J Sleep Res. 12 1: 35–41.
- Koren D, Arnon I, Lavie P, Klein E. 2002. Sleep complaints as early predictors of posttraumatic stress disorder: A 1-year prospective study of injured survivors of motor vehicle accidents. Am J Psychiatry. 159 5: 855–857.
- Lancel M, Muller-Preuss P, Wigger A, Landgraf R, Holsboer F. 2002. The CRH1 receptor antagonist R121919 attenuates stress-elicited sleep disturbances in rats, particularly in those with high innate anxiety. J Psychiatr Res. 36 4: 197–208.
- Lavie P. 2001. Sleep disturbances in the wake of traumatic events. N Engl J Med. 345 25: 1825–1832.
- Lavie P, Katz N, Pillar G, Zinger Y. 1998. Elevated awaking thresholds during sleep: Characteristics of chronic war-related posttraumatic stress disorder patients. Biol Psychiatry. 44 10: 1060–1065.
- Madan V, Brennan FX, Mann GL, Horbal AA, Dunn GA, Ross RJ, Morrison AR. 2008. Long-term effect of cued fear conditioning on REM sleep microarchitecture in rats. Sleep. 31 4: 497–503.
- McEwen BS. 2000. Allostasis and allostatic load: Implications for neuropsychopharmacology. Neuropsychopharmacology. 22 2: 108–124.
- Mikics E, Baranyi J, Haller J. 2008. Rats exposed to traumatic stress bury unfamiliar objects—A novel measure of hyper-vigilance in PTSD models?. Physiol Behav. 94 3: 341–348.
- Morgan CA3rd, Kingham P, Nicolaou A, Southwick SM. 1998. Anniversary reactions in Gulf War veterans: A naturalistic inquiry 2 years after the Gulf War. J Trauma Stress. 11 1: 165–171.
- Otto T, Cousens G, Herzog C. 1997. Odor-guided fear conditioning in rats: 1: Acquisition, retention, and latent inhibition. Behav Neurosci. 111 6: 1257–1264.
- Otto T, Cousens G, Rajewski K. 2000. Behavioral and neuropsychological foundations of olfactory fear conditioning. Behav Brain Res. 110 1–2: 119–128.
- Pawlyk AC, Jha SK, Brennan FX, Morrison AR, Ross RJ. 2005. A rodent model of sleep disturbances in posttraumatic stress disorder: The role of context after fear conditioning. Biol Psychiatry. 57 3: 268–277.
- Pawlyk AC, Morrison AR, Ross RJ, Brennan FX. 2008. Stress-induced changes in sleep in rodents: Models and mechanisms. Neurosci Biobehav Rev. 32 1: 99–117.
- Pinel JPJ, Treit D. 1983. The conditioned defensive burying paradigm and behavioral neuroscience. In: Robinson T, editors. Behavioral approaches to brain research. Oxford: Oxford University Press212–234.
- Reynolds SM, Berridge KC. 2002. Positive and negative motivation in nucleus accumbens shell: Bivalent rostrocaudal gradients for GABA-elicited eating, taste “liking”/“disliking” reactions, place preference/avoidance, and fear. J Neurosci. 22 16: 7308–7320.
- Shalev AY, Peri T, Brandes D, Freedman S, Orr SP, Pitman RK. 2000. Auditory startle response in trauma survivors with posttraumatic stress disorder: A prospective study. Am J Psychiatry. 157 2: 255–261.
- Treit D, Pinel JP, Fibiger HC. 1981. Conditioned defensive burying: A new paradigm for the study of anxiolytic agents. Pharmacol Biochem Behav. 15 4: 619–626.
- Vazquez-Palacios G, Velazquez-Moctezuma J. 2000. Effect of electric foot shocks, immobilization, and corticosterone administration on the sleep-wake pattern in the rat. Physiol Behav. 71 1–2: 23–28.