Abstract
Stress and anxiety are important causal and exacerbating factors in functional gastro-intestinal (GI) disorders such as irritable bowel syndrome. Stress affects GI motility, faecal transit and visceral pain sensitivity. Additionally, permeability and function of the gut epithelium, which acts as a barrier between the external environment and the body's internal milieu is altered by stress. However, the effects of an enhanced stress response on colonic morphology require further investigation. We have used two animal models of stress and anxiety, the maternally separated (MS) and Wistar Kyoto (WKY) rats to examine colonic morphology. These rats exhibit increased anxiety behaviours, visceral hypersensitivity and increased stress-induced defecation in the open field arena. At a morphological level, increased mucus secretion and an associated elevation in the number of mucosal goblet cells was observed in the high anxiety rats. Additionally, the mucosal layer was flattened in MS and WKY rats, a finding indicative of mild mucosal damage. Furthermore, the muscular layer of the distal colon in these animals was thickened, an observation that may have implications for faecal transit and visceral pain perception. This study provides evidence of altered colonic function and morphology in two animal models with a heightened response to stress.
Introduction
Stress-related disorders such as depression and anxiety are among the most common health problems throughout the world and they are frequently found to co-exist with functional disorders of the gastro-intestinal (GI) tract (Walker et al. Citation1992; Henningsen et al. Citation2003; Cryan and Holmes Citation2005). In patients seeking treatment for the functional GI disorder, irritable bowel syndrome (IBS), there is a strong correlation between disease severity and co-morbid lifetime psychiatric disorders, particularly depression and anxiety (Lydiard et al. Citation1993; Spiller Citation2004; Fitzgerald et al. Citation2008). IBS, which is characterised by abdominal pain and altered bowel habit is contributed to, and exacerbated by stress, in addition to early life experiences and genetics (Lowman et al. Citation1987; Mayer et al. Citation2001). Whether the symptoms of psychological distress precede IBS or are a consequence of it is still unclear, however, it is generally believed that the underlying cause of IBS is due to a complex dysregulation of the brain–gut axis (Drossman et al. Citation1999).
Two animal models which exhibit symptoms of anxiety and depression in tandem with altered colonic function are the maternally separated (MS) rat model (Plotsky et al. Citation2005; Barreau et al. Citation2007; O'Mahony et al. Citation2008) and the Wistar Kyoto (WKY) rat (Pare Citation1992; Armario et al. Citation1995; De La Garza and Mahoney Citation2004). MS rats exhibit elevated anxiety and depression-related behaviours (Lee et al. Citation2007) in addition to an exaggerated GI response to inflammatory stimuli (Varghese et al. Citation2006) and colo-rectal distension (CRD; Coutinho et al. Citation2002; Rosztoczy et al. Citation2003). Recently, our laboratory has shown that this model of early-life stress results in increased anxiety-like behaviours, altered central 5-hydroxytryptamine levels, hypervigilance, alterations in the hypothalamic–pituitary–adrenal (HPA) axis, increased stress-induced faecal output and visceral hypersensitivity (O'Mahony et al. Citation2009). Thus, it has been proposed as an appropriate model of co-morbid anxiety and functional GI disorders. Previous studies have shown that neonatal maternal separation predisposes the animals to gastric erosions (Ackerman et al. Citation1978) and enhanced stress-induced increases in colonic paracellular permeability. Furthermore, colonic ion transport is increased in these animals but basal levels of colonic permeability are unaffected (Soderholm et al. Citation2002b). The MS rat is therefore a useful animal model to study how early-life stress can result in physiological and morphological changes in the colon.
The second animal model used was the WKY rat, which was originally bred as a normotensive comparison strain for the spontaneously hypertensive rat. However, it soon became apparent that WKY rats have a genetic pre-disposition to pathological stress responses (Pare Citation1993; Lahmame et al. Citation1997). In particular, WKY rats have marked elevations in stress- and depression-associated hallmarks when compared to Sprague Dawley (SD) rats, the strain most commonly used as a control comparator. These perturbations include heightened behavioral responses to stress (Pare Citation1992; Armario et al. Citation1995; De La Garza and Mahoney Citation2004), increased immobility in the forced swim test (Lopez-Rubalcava and Lucki Citation2000; Rittenhouse et al. Citation2002), an exaggerated increase in adrenocorticotropic hormone and corticosterone levels (Rittenhouse et al. Citation2002) and a region-specific decrease in brain glial fibrillary acidic protein (CitationGosselin et al. 2008) following stressful stimuli. In addition to the depressed and anxious phenotype, increased sensitivity to CRD (Gunter et al. Citation2000; Greenwood-Van Meerveld et al. Citation2005; O'Mahony et al. Citation2009) coupled with alterations in colonic accommodation (Martinez et al. Citation2007) and function (Hyland et al. 2009) make WKY rats attractive as an alternative model for investigating how a heightened stress response coupled with increased anxiety may affect gut morphology.
The physiological stress response evokes an adaptive or allostatic response to an acute environmental threat. However, if the stress system becomes chronically activated it can induce changes in GI function, including altered colonic motility, faecal transit and visceral pain sensitivity (Bhatia and Tandon Citation2005). Stress-induced changes in gut morphology also have important implications for normal gut function as stress can affect permeability of the gut mucosal layer leading to antigen and bacterial translocation across the mucosal layer to the lamina propria. At a macroscopic level, early life stress has been shown to increase colonic weight and induce focal hyperaemia and mesenteric adhesions (Barreau et al. Citation2004). However, little is known about how enhanced stress and anxiety responses influence gut morphology at a microscopic level. Changes in colonic physiology in animal models of increased stress susceptibility would have important implications for understanding of how the brain–gut axis contributes to the symptomatology of functional stress-related GI disorders such as IBS. To this end, we have taken two stress-susceptible rat models, which display enhanced anxiety- and depression-like behaviours, and hypothesised that the colonic physiology and morphology in these rats would be altered when compared to control animals. To date, there has been limited investigation of colonic morphology in either model and thus we assess whether these rats have changes in the (1) colonic length, (2) mucus secretion and number of goblet cells, (3) thickness of the mucosal layer, (4) musculature and (5) the cytoarchitecture of crypts. Moreover, we confirmed that both models have alterations in behaviour and faecal output during an acute stress challenge.
Materials and methods
Animals
For the maternal separation model, virgin female SD rats (7–9 weeks old) from the Biological Services Unit, University College Cork were used as the parental colony. Although there are a number of protocols for maternal separation, we adapted that previously described by Wigger and Neumann (Citation1999), with slight modifications. Our protocol has given rise to robust changes in both behavioural and psychological parameters across the brain–gut axis (Hyland et al. Citation2009; O'Mahony et al. Citation2008, Citation2009). Briefly, the entire rat litter was separated from the dam for 3 h/day, (09.00–12.00 h) between postnatal day 2 and 12. During separation, each litter was placed in a clean cage over a heated blanket (30–33°C). A control, non-separated (NS) group was left undisturbed with the dam in the home cage. After weaning, female pups were culled and male pups were group housed 4–6 per cage and allowed to reach maturity. In our model, no significant differences in bodyweight were noted between NS and MS rats.
For the WKY model, male SD and WKY rats were purchased from Harlan, UK. They were permitted 1 week to acclimatise to their new surroundings prior to experimentation. For all experimental groups, rats were of comparable weight (250—350 g) and age (11–13 weeks) and all animals were in the fed state prior to experimentation. Rats were group-housed 4–6 per cage and maintained on a 12/12 h light/dark cycle (lights on at 08.00 h and off at 20.00 h), with food and water ad libitum.
Behavioural investigations
Open field. Rats were handled for 5 days prior to the open field trials which were conducted by the same investigator between 09.00 and 13.00 h each day. Rats were placed in the centre of an illuminated open field arena, measuring 0.9 m in diameter and the arena was cleaned with 70% ethanol between each trial. The total distance moved in the inner (0.65 m in diameter) and outer zones of the arena over a period of 10 min was recorded and tracked using Ethovision software (version 3.1, Noldus Information Technology, Wageningen, The Netherlands). The mean faecal output was calculated from the number of pellets excreted during the 10 min open field trial and compared between 10 NS and MS rats and behavioural analysis was conducted on five rats from each group. Faecal output and behavioural analysis were compared between 10 SD and 10 WKY rats.
Morphological analysis
A group of rats which had not undergone open field stress were analysed for changes in colonic morphology. The rats were culled by decapitation between 09.00 and 12.00 h, and colons were excised and analysed for macroscopic changes in appearance. Total length from just below the caecum to the anus was also measured for each colon. The colons were subsequently cleared of faecal pellets with cold phosphate-buffered saline (PBS), fixed overnight in PBS containing 4% (w/v) paraformaldehyde and dehydrated in PBS containing 30% (w/v) sucrose. The frozen tissue was cut into transverse sections (10 μm) of proximal and distal colon using a cryostat (Leica CM1900 UV). The mounted slices were stained with haemotoxylin and eosin (H&E) using a standard protocol. In brief, tissue was rinsed in PBS, stained with haematoxylin, cleared in water, stained with eosin, dehydrated and mounted. Colonic morphology was analysed using light microscopy and Cell F software (Olympus Soft Imaging Solutions, Hamburg, Germany). The sample sizes were based on power calculations aimed at detecting differences at the p < 0.05 significance level. Thus, five transverse sections from each region of the colon (proximal and distal) were analysed per rat. N = 5 rats were used from each group (NS, MS, SD and WKY) and for statistical purposes the average values from each rat were compared. Because of technical difficulties the tissue sections from the proximal colons of one NS and one SD rat and the distal colon from one MS and one WKY rat were excluded from analysis. Linear measurements were performed using Cell F software (Olympus). The thickness of the mucosal layer was defined as the distance between the surface epithelial layer and the muscularis mucosae. The thickness of the muscular layer was defined as the total measurement of both the circular and longitudinal muscle layers. The mean value of three measurements per section was taken for both parameters. The number of goblet cells per 0.5 mm of muscularis mucosae independent of the number of crypts was counted to compare between groups with the mean number taken from three sections. Analysis was conducted by the same observer for each group.
Reagents
All reagents were purchased commercially. Mounting medium was purchased from Fisher Scientific (Loughborough, Leicestershire, UK) and the remainder were purchased from Sigma-Aldrich (Poole, Dorset, UK).
Statistics
The data are represented as mean values ± the standard error of the mean (SEM). Statistical analysis was performed using GraphPad Prism (Version 4). Two unpaired groups were compared using a students t-test (95% confidence interval). P ≤ 0.05 was considered significant.
Results
Behavioural findings
MS rats
MS rats have previously been shown to exhibit visceral hypersensitivity, increased corticosterone levels and an altered immune responses (Chung et al. Citation2007; O'Mahony et al. Citation2009). Our MS rat colony displayed increased anxiety-induced defecation during the 10 min open field trial as compared to the less anxiety prone NS control rats (n = 10 rats per group, p < 0.05, ). Furthermore, MS rats tended to spend less time exploring the exposed inner zone of the open field than NS control rats (n = 5, p = 0.09, ), although this did not reach significance. No significant differences were noted in the velocity or total distance moved by NS and MS rats (data not shown).
Figure 1 Behavioural responses of NS and MS rats during an open field trial. A. Mean number of faecal pellets excreted by NS (open bars, n = 10) and MS (n = 10, grey bars) rats during the 10 min exposure to open field stress. (B). Total duration spent by NS and MS rats in the inner zone (seconds, n = 5). Values are mean ± SEM. *indicates p ≤ 0.05.
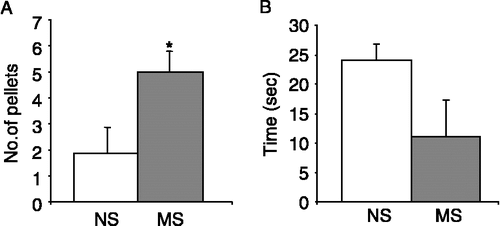
WKY rats
When placed in the open field arena for 10 min, WKY rats defecated significantly more than the control SD strain (n = 10, p < 0.001, ). Interestingly, when placed in the centre of the arena at the start of the open field trial, WKY rats were significantly slower to leave the inner zone than SD rats (n = 10, p < 0.01). However, over the course of the 10 min trial, the total time spent in the inner zone by WKY rats was significantly less than SD rats (n = 10, p < 0.05, ). As previously described (Pare Citation1994; Servatius et al. Citation2008), locomotor activity was reduced in WKY rats in comparison to SD rats with reduced velocity in both zones and significantly lower movement in the outer zone (data not shown).
Figure 2 Behavioural responses of SD and WKY rats during an open field trial. (A). The histogram illustrates the mean number of faecal pellets excreted by SD (filled bars, n = 10) and WKY (hatched bars, n = 10) rats during the 10 min open field trial. (B). The total duration of time (seconds) spent by SD (n = 10) and WKY (n = 10) rats in the exposed inner zone of the open field arena. Values are mean ± SEM. *indicates p ≤ 0.05 and ***indicates p ≤ 0.001.
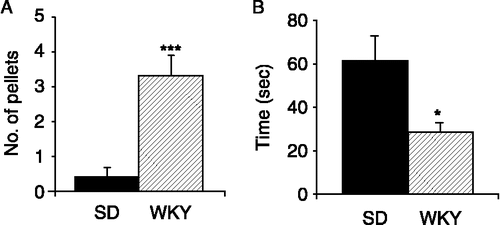
Colonic morphology
MS rats
Excised colons were measured from below the caecum to the anus and it was found that colons from MS rats were significantly shorter than NS controls (n = 5, p < 0.05, ). When the colons were opened along the mesentery, unusual morphology was noted in a number of MS colons. The mucosal layer in the proximal colon of 4/5 MS rats had deep striations which were not apparent in NS colons. Furthermore, three of the five MS colons had an apparent increase in the production of yellow-coloured mucus with none of the NS colons displaying this change. Consistent with this observation, morphological analysis of colonic H&E stained sections showed a significant increase in the number of goblet cells in the crypts of proximal MS colons. The mean numbers of goblet cells in 0.5 mm of muscularis mucosae were twofold greater in MS rats than NS rats (n = 15 sections from five rats, p < 0.05, ). A similar trend was seen in the distal colon with significantly more goblet cells in the crypts of MS colons than in comparable NS controls (n = 15 sections from 5 rats, p < 0.01, ).
Figure 3 Goblet cell hyperplasia in MS rats. (A). The histogram shows the difference in mean colonic length (from caecum to anus) between NS (open bars, n = 5) and MS (grey bars, n = 5) rats. (B). The mean number of goblet cells per 0.5 mm muscularis mucosae in sections of the proximal and distal colon of NS and MS rats (n = 3 sections each from five rats). (C). Representative H&E stained sections from the distal colon of NS (i) and MS (ii) rats illustrate goblet cell hyperplasia in MS distal colon. Goblet cells are indicated by the arrows. Scale bar = 100 μm. Values are mean ± SEM. *indicates p ≤ 0.05, **indicates p ≤ 0.01.
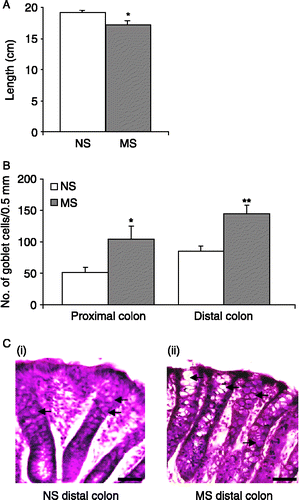
Upon further analysis, in 0.5 mm sections of proximal colon there were no significant differences in either the number (NS = 8.8 ± 0.74, MS = 8.4 ± 0.25, p>0.05) or the length (NS = 211 ± 13.9 μm, MS = 232.3 ± 16.7 μm, p>0.05) of crypts in the proximal colon. The total number (NS = 7 ± 0.6, MS = 7.6 ± 0.93, p>0.05) and lengths (NS = 309 ± 18.5 μm, MS = 293.2 ± 13.3 μm, p>0.05) of crypts in both NS and MS rats were also comparable in the distal colon, suggesting there was no change in epithelial cell proliferation or turnover. The architecture of the crypts and mucosal surface were straight and smooth, respectively, with little evidence of damage in either NS or MS rats.
Nonetheless, the mucosal layer was found to be significantly thinner in proximal sections of MS colons (n = 25 sections from five rats), as compared to NS tissue (n = 20 sections from four rats, p ≤ 0.01, ) and the columnar polarised morphology of the epithelial cells was less distinct and appeared more flattened in the MS samples. The muscular layer of the proximal colon in MS rats (n = 20 sections from four rats) was also thinner than that of the NS rats (n = 24 sections from five rats, p < 0.01, ). In the distal colon the mucosal layer was also significantly thinner in MS rats (n = 25 sections from five rats) as compared to NS rats (n = 20 sections from four rats, p < 0.05, ) and again, epithelial cell morphology was flattened in MS samples. In contrast to the proximal colon, the muscular layer in the MS distal colon (n = 20 sections from four rats) was found to be significantly thicker than that of the NS rat (n = 24 sections from five rats, p < 0.05, ).
Figure 4 Thickness of the mucosal and muscular layers in MS rats. (A). The histogram illustrates the mean thickness of the mucosal layer in the proximal and distal colon of NS (n = 20 sections from four rats, open bars) and MS (n = 25 sections from five rats, grey bars) rats. (B). The mean width of the muscular layers of NS (n = 24 sections from five rats) and MS (n = 20 sections from four rats) rats in the proximal and distal colon. Values are mean ± SEM. *indicates p ≤ 0.05, **indicates p ≤ 0.01.
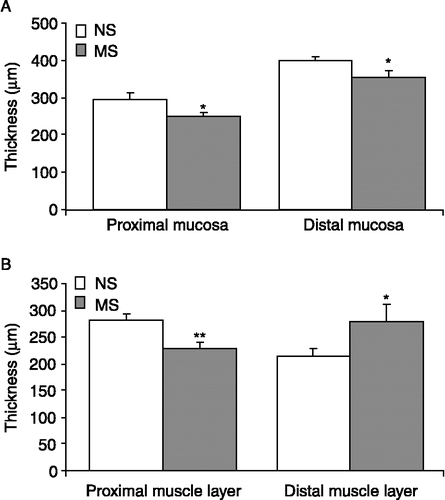
WKY rats
In contrast to the shorter colons observed in the MS rats, WKY rat colons displayed a small but significant increase in length in comparison with SD colons (n = 10, p < 0.05, ). However, when the colons were opened along the mesentery, several (6/10) WKY colons displayed poorly defined striations in the proximal colon and like the MS rats, more than half (6/10) exhibited increased mucus secretion as compared to the low-anxiety SD rat. This observation is consistent with the apparent goblet cell hyperplasia in the proximal colon. There were increased numbers of goblet cells per 0.5 mm of muscularis mucosae in WKY proximal colons as compared to the SD colons (n = 15 sections from five rats, p < 0.01, ). However, no increase in goblet cell number was noted in WKY distal colons (). Both SD and WKY colons had straight crypts and a smooth mucosal surface with no overt signs of damage. The density and length of crypts measured in 0.5 mm sections of proximal and distal colon were not dissimilar between strains in either the proximal or distal colon. However, the mucosal layer in WKY proximal colons (n = 20 sections from four rats) was thinner than that of SD rats (n = 25 sections from five rats, p ≤ 0.05, ). By contrast the mucosal layer in the distal colon of WKY rats (n = 20 sections from four rats) was significantly thicker than in SDs (n = 25 sections from five rats, p < 0.05, ). The thickness of the muscular wall in the proximal colon was similar in both strains but in the distal colon, WKY rats (n = 20 sections from four rats) had a significantly thicker muscular layer than the SD controls (n = 25 sections from five rats, p < 0.05, ).
Figure 5 Goblet cell hyperplasia in WKY rats. (A). The bar chart demonstrates the small but significant increase in colonic length in WKY rats (hatched bars, n = 10) versus SD rats (filled bars, n = 10). (B). The histogram illustrates that the mean number of goblet cells in the crypts (counted for 0.5 mm of muscularis mucosae) are increased in the proximal colon of WKY rats (n = 15 sections from five rats) but unchanged in the distal colon. (C). Representative H&E stained colonic sections illustrate increased numbers of goblet cells in the proximal colon of WKY rats (ii) as compared to SD colons (i). Goblet cells are indicated by the arrows. Scale bar = 100 μm. Values are mean ± SEM. *indicates p ≤ 0.05.
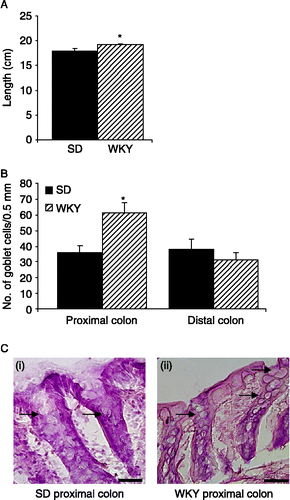
Figure 6 Thickness of the mucosal and muscular layers of WKY rats. (A). The histogram illustrates the mean thickness of the mucosal layers in the proximal and distal colon of SD (filled bars n = 25 sections from five rats) and WKYs (hatched bars n = 20 sections from four rats). (B). The mean thickness of the muscular layers in SD (n = 25 sections from five rats) and WKY (n = 20 sections from four rats) proximal and distal colons. Values are mean ± SEM. *indicates p ≤ 0.05.
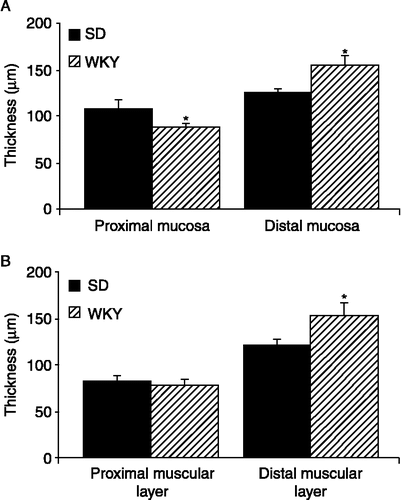
Discussion
Stress and anxiety are thought to contribute to the symptoms of functional GI tract disorders with stressful events frequently inducing symptom flare-ups (Mayer et al. Citation2001; Ringel and Drossman Citation2001). The outcomes of this study demonstrate that colonic physiology and morphology are altered in two animal models which are characterised by their susceptibility to stress. The behavioural responses of the MS and WKY rats to the novel stressful stimulus of the open field arena provide additional evidence that these rats are appropriate models of increased stress-susceptibility, and therefore changes in gut function and morphology have important implications for stress-related GI disorders such as IBS. Consistent with previous reports, we found that MS (O'Mahony et al. Citation2009) and WKY rats defecated significantly more in the open field than their lower anxiety counterparts, an outcome that fits with stress increasing colonic motility (Saito et al. Citation2005). Additionally, reduction in exploration and movement in the open field is generally associated with increased anxiety (Stanford Citation2007), which was borne out in our studies with MS rats being less inclined to enter the exposed inner zone and tending to stay near the walls of the arena. Interestingly, WKY rats, which are often used as a model of anxiety and depression (Wieland et al. Citation1986; Pare Citation1992; Armario et al. Citation1995; De La Garza and Mahoney Citation2004), responded to being placed in the open field by freezing as a strategy to adapt to the novel environment. These data are consistent with recent evidence that suggests WKY rats respond to a novel environment with decreased activity and behavioural withdrawal (Pare Citation1994; Servatius et al. Citation2008).
The effects of stress on morphology of the colon was assessed as this region was most related to the physiological changes that have been described such as increased faecal output, colonic accommodation and visceral hypersensitivity (Martinez et al. Citation2007; O'Mahony et al. Citation2009). Excised colons were examined for macroscopic and microscopic changes in morphology. It was noted that 60% of MS and WKY colons but none of the lower anxiety animals had visibly increased levels of mucus secretion. Although previously described in IBS patients (Mayer and Collins Citation2002), to our knowledge increased secretion of mucus has not previously been described in an IBS animal model. Mucus protects the underlying epithelial cells from shear stresses and also eases the passage of faecal pellets (Brownlee et al. Citation2003). Although often overlooked the mucus layer is also a crucial component of the innate immune system. The colonic mucus layer is believed to be made up of two layers rich in the secreted gel-forming mucin muc2. The inner densely packed layer creates a mucus barrier that can protect colonic epithelia from damaging luminal agents (Johansson et al. Citation2008). Inflammatory reactions have been linked with goblet cell hyperplasia as in the case of nematode infections where the goblet cells enlarge and release mucus (Artis et al. Citation2004; Pemberton et al. Citation2004a, Citation2004b). It is therefore of interest that maternally deprived rats are more susceptible to nematode infection despite an increase in goblet cell number (Barreau et al. Citation2006). Muc2 and Trefoil factor 3 when secreted by goblet cells into the mucus, have been shown to act cooperatively in the protection of epithelial cells from toxic assault (Kindon et al. Citation1995). Although beyond the scope of the current study, future investigations focused on the impact of stress on the secretion of these factors will be interesting.
Goblet cells are highly polarised cells that synthesise and secrete mucins to maintain the mucus blanket (Specian and Oliver Citation1991; Robbe et al. Citation2004). Consistent with an increase in mucus secretion, microscopic examination of H&E stained tissue sections revealed increased numbers of goblet cells in the proximal colon of both MS and WKY rats and in the distal colon of MS rats (). The effect was particularly pronounced in the MS colons, where the numbers of goblet cells were increased twofold along the entire length of the colon. Previous studies which examined the effects of stress on mucus secretion, demonstrated how variability in the type of stressor could result in divergent morphological changes. Acute wrap restraint stress in Wistar rats resulted in increased mucosity (Castagliuolo et al. Citation1996), whereas a 10-day chronic stress paradigm resulted in a decrease in goblet cell number and mucin depletion (Soderholm et al. Citation2002a). Interestingly, it has been shown that goblet cells in WKY rats appear to be susceptible to stress-induced uptake of antigens (Kiliaan et al. Citation1998) which may indicate a possible mechanism by which an immune response to the foreign proteins either initiates or exacerbates inflammation. Such alterations may in turn have a role in the manifestation of the increased sensitivity to visceral pain and other aspects of IBS symptomatology.
In both the MS and WKY proximal colons and in the distal colon of MS rats, the mucosal layer was significantly thinner than in control rats. Flattening of mucosal epithelial cells is indicative of mild mucosal damage or cell restitution (Dignass et al. Citation1994). This is in contrast to the mucosa of the distal colon of WKY rats, which was significantly thicker than that of SD rats, which may indicate increased cellular proliferation and cell turnover in this region although no change in crypt length was evident. The total thickness of the muscle layer, including both circular and longitudinal muscle was measured and was found to be thinner in the proximal colon of MS animals than NS controls. No statistical difference in the thickness of the muscle layer in this region was evident between SD and WKY rats. In contrast, the muscle layer in the distal colon which was significantly thicker in both MS and WKY rats when compared to their lower anxiety counterparts. Many animal models have shown circular muscle hypertrophy and abnormalities of neuromuscular innervation following inflammation and this is thought to be directly linked to susceptibility to visceral hypersensitivity (Spiller Citation2006). Thus, the changes in muscle thickness and by inference, changes in the contractility of the muscular layer may have important implications for visceral pain sensation in the distal colon as well as the rate of faecal transit.
The results from this study demonstrate that two animal models of IBS which have enhanced stress susceptibility and anxiety display altered colonic function and morphology. Functionally, faecal output during the 10 min open field trial was increased in both MS and WKY rats as compared to their respective controls. At a cellular level, goblet cell hyperplasia was evident in the colonic crypts of both MS and WKY rats which is associated with increased mucus secretions. Further changes were noted in the mucosal layer which was flattened in the MS and WKY proximal colons and the distal colon of the MS rats and there was also evidence of thickening of the muscle layer in the distal colon of MS and WKY with the associated consequences for colonic motility and visceral pain sensation. It should be noted that stress can stimulate functional changes in other areas within the GI tract especially the ileum. Further studies focusing on stress-induced changes in the ileum or other regions of the GI tract would also be warranted.
Both of the animal models of IBS investigated show alterations in the HPA-axis with increased stress-induced corticosterone levels in the MS (O'Mahony et al. Citation2009) and WKY rats (Rittenhouse et al. Citation2002). Future studies should focus on disentangling the role of HPA-axis components on the manifestation of colonic morphological changes observed herein. Moreover, there is also evidence to suggest changes in immune function such as mast cell hyperplasia in the MS (Barreau et al. Citation2004; Hyland et al. Citation2009) and WKY rats (Hyland et al, unpublished observations) which may also have a role in the observed goblet cell hyperplasia (Soderholm et al. Citation2002a). Further investigations will be required to define the molecular mechanisms underlying such changes, especially in regard to their link with the HPA-axis, immune, central and enteric nervous system alterations observed in both models of IBS. Moreover, from a therapeutic point of view, pharmacological studies should investigate whether resolution of the changes in morphology outlined are coincident with reversal of behavioural and physiological parameters in these models.
The novel findings of this morphological study demonstrate that increased anxiety and susceptibility to stress in two animal models of IBS are associated with a number of distinct changes in colonic physiology and morphology. These data provide additional evidence that stress-induced changes in brain–gut communication are linked with colonic changes at both a functional and cellular level.
Acknowledgements
We wish to express our gratitude to Pat Fitzgerald for his technical assistance. All experiments were in full accordance with the European Community Council Directive (86/609/EEC) and were approved by the local ethical committee in University College Cork.
Declaration of interest. JFC and TGD are supported in part by Science Foundation Ireland in the form of a Centre grant (Alimentary Pharmabiotic Centre). The centre is also funded by GlaxoSmithKline.
References
- Ackerman SH, Hofer MA, Weiner H. 1978. Predisposition to gastric erosions in the rat: Behavioral and nutritional effects of early maternal separation. Gastroenterology. 75:649–654.
- Armario A, Gavalda A, Marti J. 1995. Comparison of the behavioural and endocrine response to forced swimming stress in five inbred strains of rats. Psychoneuroendocrinology. 20:879–890.
- Artis D, Wang ML, Keilbaugh SA, He W, Brenes M, Swain GP, Knight PA, Donaldson DD, Lazar MA, Miller HR, Schad GA, Scott P, Wu GD. 2004. RELMbeta/FIZZ2 is a goblet cell-specific immune-effector molecule in the gastrointestinal tract. Proc Natl Acad Sci USA. 101:13596–13600.
- Barreau F, Ferrier L, Fioramonti J, Bueno L. 2004. Neonatal maternal deprivation triggers long term alterations in colonic epithelial barrier and mucosal immunity in rats. Gut. 53:501–506.
- Barreau F, de Lahitte JD, Ferrier L, Frexinos J, Bueno L, Fioramonti J. 2006. Neonatal maternal deprivation promotes Nippostrongylus brasiliensis infection in adult rats. Brain Behav Immun. 20:254–260.
- Barreau F, Ferrier L, Fioramonti J, Bueno L. 2007. New insights in the etiology and pathophysiology of irritable bowel syndrome: Contribution of neonatal stress models. Pediatr Res. 62:240–245.
- Bhatia V, Tandon RK. 2005. Stress and the gastrointestinal tract. J Gastroenterol Hepatol. 20:332–339.
- Brownlee IA, Havler ME, Dettmar PW, Allen A, Pearson JP. 2003. Colonic mucus: Secretion and turnover in relation to dietary fibre intake. Proc Nutr Soc. 62:245–249.
- Castagliuolo I, Lamont JT, Qiu B, Fleming SM, Bhaskar KR, Nikulasson ST, Kornetsky C, Pothoulakis C. 1996. Acute stress causes mucin release from rat colon: Role of corticotropin releasing factor and mast cells. Am J Physiol. 271:G884–G892.
- Chung EK, Zhang X, Li Z, Zhang H, Xu H, Bian Z. 2007. Neonatal maternal separation enhances central sensitivity to noxious colorectal distention in rat. Brain Res. 1153:68–77.
- Coutinho SV, Plotsky PM, Sablad M, Miller JC, Zhou H, Bayati AI, McRoberts JA, Mayer EA. 2002. Neonatal maternal separation alters stress-induced responses to viscerosomatic nociceptive stimuli in rat. Am J Physiol Gastrointest Liver Physiol. 282:G307–G316.
- Cryan JF, Holmes A. 2005. The ascent of mouse: Advances in modelling human depression and anxiety. Nat Rev Drug Discov. 4:775–790.
- De La Garza R2nd, Mahoney JJ3rd. 2004. A distinct neurochemical profile in WKY rats at baseline and in response to acute stress: Implications for animal models of anxiety and depression. Brain Res. 1021:209–218.
- Dignass AU, Tsunekawa S, Podolsky DK. 1994. Fibroblast growth factors modulate intestinal epithelial cell growth and migration. Gastroenterology. 106:1254–1262.
- Drossman DA, Creed FH, Olden KW, Svedlund J, Toner BB, Whitehead WE. 1999. Psychosocial aspects of the functional gastrointestinal disorders. Gut. 45 Suppl. 2: II25–II30.
- Fitzgerald P, Cassidy Eugene M, Clarke G, Scully P, Barry S, Quigley Eamonn MM, Shanahan F, Cryan J, Dinan Timothy G. 2008. Tryptophan catabolism in females with irritable bowel syndrome: Relationship to interferon-gamma, severity of symptoms and psychiatric co-morbidity. Neurogastroenterol Motil. 20: 1291–1297.
- Gosselin RD, Gibney S, O'Malley D, Dinan TG, Cryan JF. 2009. Region specific decrease in glial fibrillary acidic protein immunoreactivity in the brain of a rat model of depression. Neuroscience 159:915–925.
- Greenwood-Van Meerveld B, Johnson AC, Cochrane S, Schulkin J, Myers DA. 2005. Corticotropin-releasing factor 1 receptor-mediated mechanisms inhibit colonic hypersensitivity in rats. Neurogastroenterol Motil. 17:415–422.
- Gunter WD, Shepard JD, Foreman RD, Myers DA, Greenwood-Van Meerveld B. 2000. Evidence for visceral hypersensitivity in high-anxiety rats. Physiol Behav. 69:379–382.
- Henningsen P, Zimmermann T, Sattel H. 2003. Medically unexplained physical symptoms, anxiety, and depression: A meta-analytic review. Psychosom Med. 65:528–533.
- Hyland NP, Julio-Pieper M, O'Mahony SM, Bulmer DC, Lee K, Quigley EM, Dinan TG, Cryan JF. 2009. A distinct subset of submucosal mast cells undergoes hyperplasia following neonatal maternal separation: A role in visceral hypersensitivity?. Gut. 58:1029–1030 author reply 1030–1021.
- Johansson ME, Phillipson M, Petersson J, Velcich A, Holm L, Hansson GC. 2008. The inner of the two Muc2 mucin-dependent mucus layers in colon is devoid of bacteria. Proc Natl Acad Sci USA. 105:15064–15069.
- Kiliaan AJ, Saunders PR, Bijlsma PB, Berin MC, Taminiau JA, Groot JA, Perdue MH. 1998. Stress stimulates transepithelial macromolecular uptake in rat jejunum. Am J Physiol. 275:G1037–G1044.
- Kindon H, Pothoulakis C, Thim L, Lynch-Devaney K, Podolsky DK. 1995. Trefoil peptide protection of intestinal epithelial barrier function: Cooperative interaction with mucin glycoprotein. Gastroenterology. 109:516–523.
- Lahmame A, Grigoriadis DE, De Souza EB, Armario A. 1997. Brain corticotropin-releasing factor immunoreactivity and receptors in five inbred rat strains: Relationship to forced swimming behaviour. Brain Res. 750:285–292.
- Lee JH, Kim HJ, Kim JG, Ryu V, Kim BT, Kang DW, Jahng JW. 2007. Depressive behaviors and decreased expression of serotonin reuptake transporter in rats that experienced neonatal maternal separation. Neurosci Res. 58:32–39.
- Lopez-Rubalcava C, Lucki I. 2000. Strain differences in the behavioral effects of antidepressant drugs in the rat forced swimming test. Neuropsychopharmacology. 22:191–199.
- Lowman BC, Drossman DA, Cramer EM, McKee DC. 1987. Recollection of childhood events in adults with irritable bowel syndrome. J Clin Gastroenterol. 9:324–330.
- Lydiard RB, Fossey MD, Marsh W, Ballenger JC. 1993. Prevalence of psychiatric disorders in patients with irritable bowel syndrome. Psychosomatics. 34:229–234.
- Martinez V, Ryttinger M, Kjerling M, Astin-Nielsen M. 2007. Characterisation of colonic accommodation in Wistar Kyoto rats with impaired gastric accommodation. Naunyn Schmiedebergs Arch Pharmacol. 376:205–216.
- Mayer EA, Collins SM. 2002. Evolving pathophysiologic models of functional gastrointestinal disorders. Gastroenterology. 122:2032–2048.
- Mayer EA, Naliboff BD, Chang L, Coutinho SV. 2001. Stress and irritable bowel syndrome. Am J Physiol Gastrointest Liver Physiol. 280:G519–G524.
- O'Mahony S, Chua AS, Quigley EM, Clarke G, Shanahan F, Keeling PW, Dinan TG. 2008. Evidence of an enhanced central 5HT response in irritable bowel syndrome and in the rat maternal separation model. Neurogastroenterol Motil. 20:680–688.
- O'Mahony SM, Marchesi JR, Scully P, Codling C, Ceolho AM, Quigley EM, Cryan JF, Dinan TG. 2009. Early life stress alters behavior, immunity, and microbiota in rats: Implications for irritable bowel syndrome and psychiatric illnesses. Biol Psychiatry 65:263–267.
- Pare WP. 1992. The performance of WKY rats on three tests of emotional behavior. Physiol Behav. 51:1051–1056.
- Pare WP. 1993. Passive-avoidance behavior in Wistar–Kyoto (WKY), Wistar, and Fischer-344 rats. Physiol Behav. 54:845–852.
- Pare WP. 1994. Open field, learned helplessness, conditioned defensive burying, and forced-swim tests in WKY rats. Physiol Behav. 55:433–439.
- Pemberton AD, Knight PA, Gamble J, Colledge WH, Lee JK, Pierce M, Miller HR. Innate BALB/c enteric epithelial responses to Trichinella spiralis: Inducible expression of a novel goblet cell lectin, intelectin-2, and its natural deletion in C57BL/10 mice. J Immunol. 2004a; 173:1894–1901.
- Pemberton AD, Knight PA, Wright SH, Miller HR. Proteomic analysis of mouse jejunal epithelium and its response to infection with the intestinal nematode, Trichinella spiralis. Proteomics. 2004b; 4:1101–1108.
- Plotsky PM, Thrivikraman KV, Nemeroff CB, Caldji C, Sharma S, Meaney MJ. 2005. Long-term consequences of neonatal rearing on central corticotropin-releasing factor systems in adult male rat offspring. Neuropsychopharmacology. 30:2192–2204.
- Ringel Y, Drossman DA. 2001. Psychosocial aspects of Crohn's disease. Surg Clin North Am. 81:231–252.
- Rittenhouse PA, Lopez-Rubalcava C, Stanwood GD, Lucki I. 2002. Amplified behavioral and endocrine responses to forced swim stress in the Wistar–Kyoto rat. Psychoneuroendocrinology. 27:303–318.
- Robbe C, Capon C, Coddeville B, Michalski JC. 2004. Diagnostic ions for the rapid analysis by nano-electrospray ionization quadrupole time-of-flight mass spectrometry of O-glycans from human mucins. Rapid Commun Mass Spectrom. 18:412–420.
- Rosztoczy A, Fioramonti J, Jarmay K, Barreau F, Wittmann T, Bueno L. 2003. Influence of sex and experimental protocol on the effect of maternal deprivation on rectal sensitivity to distension in the adult rat. Neurogastroenterol Motil. 15:679–686.
- Saito K, Kasai T, Nagura Y, Ito H, Kanazawa M, Fukudo S. 2005. Corticotropin-releasing hormone receptor 1 antagonist blocks brain–gut activation induced by colonic distention in rats. Gastroenterology. 129:1533–1543.
- Servatius RJ, Jiao X, Beck KD, Pang KC, Minor TR. 2008. Rapid avoidance acquisition in Wistar–Kyoto rats. Behav Brain Res. 192:191–197.
- Soderholm JD, Yang PC, Ceponis P, Vohra A, Riddell R, Sherman PM, Perdue MH. Chronic stress induces mast cell-dependent bacterial adherence and initiates mucosal inflammation in rat intestine. Gastroenterology. 2002a; 123:1099–1108.
- Soderholm JD, Yates DA, Gareau MG, Yang PC, MacQueen G, Perdue MH. Neonatal maternal separation predisposes adult rats to colonic barrier dysfunction in response to mild stress. Am J Physiol Gastrointest Liver Physiol. 2002b; 283:G1257–G1263.
- Specian RD, Oliver MG. 1991. Functional biology of intestinal goblet cells. Am J Physiol. 260:C183–C193.
- Spiller RC. 2004. Irritable bowel syndrome. Br Med Bull. 72:15–29.
- Spiller R. 2006. How inflammation changes neuromuscular function and its relevance to symptoms in diverticular disease. J Clin Gastroenterol. 40 Suppl. 3: S117–S120.
- Stanford SC. 2007. The open field test: Reinventing the wheel. J Psychopharmacol. 21:134–135.
- Varghese AK, Verdu EF, Bercik P, Khan WI, Blennerhassett PA, Szechtman H, Collins SM. 2006. Antidepressants attenuate increased susceptibility to colitis in a murine model of depression. Gastroenterology. 130:1743–1753.
- Walker EA, Katon WJ, Jemelka RP, Roy-Bryne PP. 1992. Comorbidity of gastrointestinal complaints, depression, and anxiety in the epidemiologic catchment area (ECA) study. Am J Med. 92:26S–30S.
- Wieland S, Boren JL, Consroe PF, Martin A. 1986. Stock differences in the susceptibility of rats to learned helplessness training. Life Sci. 39:937–944.
- Wigger A, Neumann ID. 1999. Periodic maternal deprivation induces gender-dependent alterations in behavioral and neuroendocrine responses to emotional stress in adult rats. Physiol Behav. 66:293–302.