Abstract
Psychological stress is a risk factor for cardiovascular disease including atherosclerosis, but the mechanisms are unknown. The vascular lectin-like oxidized low-density lipoprotein receptor-1 (LOX-1) is involved in vascular pathology and early atherogenesis. We hypothesized that LOX-1 is up-regulated by psychological stress via the formation of oxygen-derived free radicals, and that treatment with EUK-8 (a superoxide dismutase and catalase mimetic) prevents production of oxygen-derived free radicals and leads to reduced expression of LOX-1 in the vascular wall. As a model for psychological stress, we exposed male apolipoprotein E-deficient mice to repeated restraint stress by placement in a conical tube for 2 h per day for 14 consecutive days. Stressed and control mice were treated with EUK-8 (n = 4–5) or vehicle (n = 4–5). Reactive oxygen species and peroxynitrite levels, as detected by oxidative fluorescence microscopy, were increased in the aortic root of mice exposed to stress compared to those of controls by 212 ± 22% (mean ± SEM; p < 0.001) and 110 ± 6% (p < 0.001), respectively. LOX-1, as detected by immunohistochemistry, was increased by 443 ± 63% in stressed mice compared to control mice (p < 0.001). EUK-8 reduced reactive oxygen species, peroxynitrite, and LOX-1 levels in stressed mice compared to vehicle-treated stressed mice. To conclude, LOX-1 induced by reactive oxygen species and/or peroxynitrite could be one mechanism by which stress promotes cardiovascular disease.
Introduction
In humans and animal models alike, psychological stress is a risk factor for cardiovascular disease including atherosclerosis. The exact underlying mechanisms by which psychological stress influences the progression of atherosclerosis are not known. It is known, however, that psychological stress can translate into increased oxidative stress through a variety of mechanisms, such as activation of inflammatory pathways, the sympathetic nervous system, and the hypothalamic–pituitary–adrenal axis (Epel et al. Citation2004; Sivonova et al. Citation2004; Nation et al. Citation2008; Bauer et al. Citation2009). Excessive production of oxygen-derived free radicals such as superoxide can lead to endothelial dysfunction by scavenging vascular nitric oxide, which results in the formation of peroxynitrite. Reduced nitric oxide level, in turn, can increase adhesion of platelets to vascular endothelium, reduce vasodilation, and promote coagulation. Oxygen-derived free radicals may also contribute to atherogenesis by promoting oxidation of low-density lipoprotein (LDL) to oxLDL recruitment of macrophages into the vessel wall. The lectin-like oxLDL receptor-1 (LOX-1) is a scavenger receptor expressed in endothelial and vascular smooth muscle cells (Sawamura et al. Citation1997), but also in macrophages (Yoshida et al. Citation1998), monocytes (Draude et al. Citation1999), and platelets (Chen et al. Citation2001). LOX-1 takes up oxLDL, and during this process, the receptor generates superoxide via nicotinamide adenine dinucleotide phosphate (reduced form) (NADPH) oxidase (Cominacini et al. Citation2001). Interestingly, we have previously demonstrated that peroxynitrite can up-regulate LOX-1 in isolated endothelial cells (Sankaralingam et al. Citation2009a), thus providing a feed forward loop for generation of reactive oxygen species. However, whether this mechanism could contribute to pathological conditions such as the development of atherosclerosis is not known.
Human and animal studies have demonstrated the presence of LOX-1 in the endothelial cells of early atherosclerotic lesions. This suggests a potential role for LOX-1 in the initiation of atherosclerosis. Moreover, the continued expression of LOX-1 in advanced atherosclerotic lesions has been linked to complications such as plaque rupture (Chen et al. Citation2000a,Citationb, Citation2002). Given the importance of LOX-1 in vascular pathology, we tested the hypothesis that psychological stress, induced by restraint stress, is associated with increased amounts of LOX-1 protein in the vascular wall due to an increase in reactive oxygen species and peroxynitrite. To test this, we used the well-established genetically modified apolipoprotein E-deficient mouse strain (Apoe− / − ), which is prone to develop atherosclerotic lesions similar to those seen in humans (Nakashima et al. Citation1994) and treated them with an oxygen-derived free radical scavenger, EUK-8.
Materials and methods
Animals
We used atherosclerosis-prone male Apoe− / − mice (B6.129P2-Apoetm1Unc/J, n = 19, Jackson Laboratory, Bar Harbour, ME, USA) because rodents do not normally develop atherosclerosis due to the low concentrations of serum LDL in relation to concentrations of high-density lipoprotein. In addition, the importance of LOX-1 in vascular pathology has been demonstrated in this strain of mice (Inoue et al. Citation2005). They were allowed to acclimatize for 1 week and kept in a controlled environment with a 12 h light/dark cycle with lights on from 06:00 to 18:00 h, and had free access to normal chow and water. The University of Alberta Animal Care and Use Committee approved all procedures of this study. The procedures were in accordance with federal, state, and local laws and regulations and were in compliance with the Institute for Laboratory Animal Research Guide for Care and Use of Laboratory Animals, Washington, DC, USA: National Academy Press, 1996.
Groups and treatments
We used 10-week-old mice, which is when atherosclerosis just starts to develop in Apoe− / − mice. Stress was induced by restraining them for 2 h per day for 14 days, based on previous studies (Kim and Han Citation2006). The restraint container consisted of a perforated plastic tube placed horizontally (length: 11 cm, diameter: 3 cm, nose end: conical with breathing hole, tail end: occluded with a screw cap). Thus, the mouse could breathe freely, but movements were restricted. Unstressed mice serving as controls were kept in their home cages. All mice were housed 4–5 per cage.
One group of mice was subjected to restraint stress as described above and treated with the superoxide dismutase and catalase mimetic EUK-8 (stress/EUK-8, n = 5). Twenty-five micrograms of EUK-8 (EMD Biosciences, Inc., San Diego, CA, USA) were dissolved in 100 μl dimethyl sulfoxide (DMSO) and further diluted in 12.5 ml ddH2O containing 0.9% NaCl to a final concentration of 2 mg/ml. Each mouse was given an intra-peritoneal injection of 0.55 ml every other day (corresponding to approximately EUK-8, 50 mg/kg). To control for effects of EUK-8, an unstressed group was given EUK-8 injections every other day (control/EUK-8, n = 5). Additional groups consisted of stressed or unstressed mice, given 0.55 ml vehicle injections (prepared as described above) every other day (stress/Veh, n = 5 and control/Veh, n = 4, respectively).
Tissue collection
On experimental day 14, immediately after the last stress session and at corresponding time of day (between 10:00 and 10:45 h) for control mice, blood samples for plasma corticosterone measurement were collected to confirm the stress effect of restraint. Each mouse was loosely wrapped in a soft cloth, the tail vein was nicked with a surgical blade, and approximately 75 μl blood was collected in heparinized micro-hematocrit capillary tubes (Fisher Scientific, Pittsburgh, PA, USA), all within 2 min from picking the mouse up, to avoid corticosterone release due to handling. Following centrifugation of the blood, the plasma was stored at − 80°C until analysis. Corticosterone was analyzed in duplicates using a single 96-well plate of a correlate-EIA kit (Assay Designs, Ann Arbor, MI, USA).
Body weights were recorded, and the mice were euthanized using isoflurane inhalation (Halocarbon Products Corp., River Edge, NJ, USA). We examined the aortic root in this study because we were interested in pro-atherosclerotic processes, and this site is prone to early atherosclerosis development in Apoe− / − mice. Thus, the heart along with the aortic root was dissected out, embedded in optimal cutting temperature medium (OCT, Sakura Finetek USA, Inc., Torrance, CA, USA), frozen in liquid nitrogen, and stored at − 80°C. The first 500 μm of the aortic root was sectioned into 10 and 20 μm transverse sections for immunohistochemistry and oxidative fluorescence microscopy, respectively, and stored at − 80°C.
Vascular assessment of oxidative stress
Oxidative fluorescence microscopy was used to compare the production of reactive oxygen species, nitric oxide, and peroxynitrite in aortic root sections using cell-permeable dyes (Invitrogen, Burlington, Ont., Canada). Prior to incubation with the respective dyes, the aortic root sections (20 μm) on microscope slides stored at − 80°C were thawed out, washed thrice with Hank's balanced salt solution (HBSS, containing calcium and magnesium), and incubated with HBSS for 10 min at 37°C. Subsequently, the respective dyes were added, incubated at 37°C, washed to remove the excess dye on the surface of the aortic root section, cover slipped, and imaged using an IX81 Olympus fluorescence microscope.
Reactive oxygen species were detected using dihydroethidine (a relatively specific dye for superoxide), 20 μmol/l for 20 min, cover slipped, and photographed through cyanine 3 (CY3) filter.
Nitric oxide was detected using 3-amino,4-aminomethyl-2′,7′-difluorofluorescein diacetate (DAF-FM DA, 5 μmol/l, 30 min, photographed through a fluorescein isothiocyanate (FITC) filter). DAF-FM DA is a cell-permeable, photo-stable nitric oxide fluorescent indicator.
Peroxynitrite was detected in aortic root sections using dihydrorhodamine 123 (5 μmol/l, 30 min, photographed through a CY3 filter), a cell permeable dye that is oxidized mainly by peroxynitrite to rhodamine 123.
Immunohistochemistry for LOX-1
Sections on microscope slides were fixed with acetone, blocked with 1% bovine serum albumin, and incubated with rabbit anti-mouse polyclonal antibody against LOX-1 (1:100, Santa Cruz Biotechnologies, Santa Cruz, CA, USA) and FITC anti-rabbit secondary antibody (1:200, Alexa Fluor 488, Invitrogen). Cover slips were applied using mounting medium containing 4′,6-diamidino-2-phenylindole (DAPI) (Vector Laboratories, Burlingame, CA, USA) to counterstain the nucleus. At least three different parts of the aortic root were imaged per section.
Data analyses
Data quantification
Fluorescent microphotographs were analyzed using Adobe Photoshop. The outline of the aortic root section to be analyzed was marked, and the total intensity for the particular wavelength obtained was divided by the area in pixels to obtain a mean fluorescence intensity. Values were expressed as percentage of control/Veh group. It was necessary to normalize the result to control/Veh group because we could not process all samples in one experiment. Data imputation was used to assign a SEM to the control/Veh group in order to perform a valid two-way analysis of variance (ANOVA). LOX-1 was semi-quantified by measuring fluorescence intensity of the captured images in Adobe Photoshop and expressed as mean fluorescence intensity.
Statistics
We performed a two-way ANOVA with group (control or stress) and treatment (Veh or EUK-8) as factors; Bonferroni post hoc tests were performed to make specific comparisons. We used GraphPad Prism version 5.01 for Windows, GraphPad Software, San Diego, CA, USA, www.graphpad.com. All data are presented as group mean ± SEM, and p < 0.05 was considered statistically significant.
Results
Plasma corticosterone and body weights
On experimental day 14, directly after stress for the stress groups and at the corresponding time of day for control groups, the stress/Veh and stress/EUK-8 groups had significantly higher plasma corticosterone concentration (147 ± 16 and 204 ± 17 ng/ml, respectively) compared to control/Veh and control/EUK-8 groups (34 ± 8 and 54 ± 5 ng/ml, respectively), indicating a significant effect of group (df = 1, F = 93, p < 0.001). This confirms that the restraint efficiently evoked a stress response. The correlation coefficient for the standard curve in the corticosterone assay was 1.0. Body weights did not differ between groups on the last day of stress (control/Veh, 23 ± 1 g; stress/Veh, 24 ± 1 g; control/EUK-8, 24 ± 2 g; and stress/EUK-8, 24 ± 1 g).
Effect of restraint stress and EUK-8 on vascular oxidative stress
Reactive oxygen species
We first examined the effect of stress on aortic root reactive oxygen species production. There was a significant effect of group (df = 1, F = 66, p < 0.0001), treatment (df = 1, F = 78, p < 0.0001), and an interaction (df = 1, F = 27, p < 0.001). Post hoc test showed that when compared to the control/Veh group, reactive oxygen species production was increased by approximately 200% (p < 0.001) in the stress/Veh group. Interestingly, treatment of stressed mice with EUK-8 significantly reduced reactive oxygen species formation (p < 0.001) to amounts similar to those in the control/Veh group. EUK-8 also reduced reactive oxygen species formation in control mice by approximately 60% compared to the control/Veh group (p < 0.05, ).
Figure 1 Effect of restraint stress on aortic root reactive oxygen species generation in Apoe− / − mice. Representative images showing reactive oxygen species generation in aortic root transverse sections from Apoe− / − mice. Mice were either vehicle treated: control/Veh (n = 4) and stress/Veh (n = 4) or treated with EUK-8, an oxygen-derived free radical scavenger: control/EUK-8 (n = 4) and stress/EUK-8 (n = 4). Stressed mice were exposed to restraint stress for 2 h per day for 14 consecutive days. EUK-8 or vehicle was administered by intra-peritoneal injection every other day for 14 days. The lower panel shows a summary graph. Data are group means ± SEM. *p < 0.05, ***p < 0.001, post hoc Bonferroni tests.
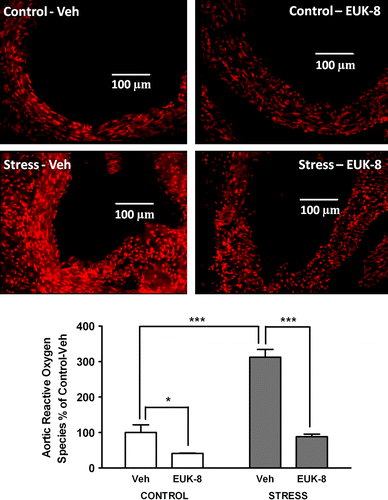
Nitric oxide
We also examined the effect of stress and EUK-8 treatment on nitric oxide in the aortic root. There was no significant effect of group, where as EUK-8 treatment had a significant effect (df = 1, F = 68, p < 0.0001); and there was an interaction (df = 1, F = 6, p < 0.05). EUK-8 treatment led to significantly increased nitric oxide formation in both the control (p < 0.01) and stressed (p < 0.001) groups compared to their respective non-treated controls ().
Figure 2 Effect of restraint stress on aortic root nitric oxide and peroxynitrite generation in Apoe− / − mice. Aortic root nitric oxide (A) and peroxynitrite (B) formation was assessed using the cell-permeable dyes DAF-FM DA and dihydrorhodamine 123, respectively, in aortic root transverse sections of Apoe− / − mice. Mice were either vehicle treated: control/Veh (n = 4–5) or stress/Veh (n = 4) or treated with EUK-8, an oxygen-derived free radical scavenger: control/EUK-8 (n = 4) or stress/EUK-8 (n = 4). Stressed mice were exposed to restraint stress for 2 h per day for 14 consecutive days. EUK-8 or vehicle was administered by intra-peritoneal injection every other day for 14 days. The mean intensity of the staining (photographs not shown) was used to calculate the percent values shown in the summary graphs for nitric oxide (A) and peroxynitrite (B) formation. Data are group means ± SEM. **p < 0.01, ***p < 0.001, NS, not significant, post hoc Bonferroni tests.
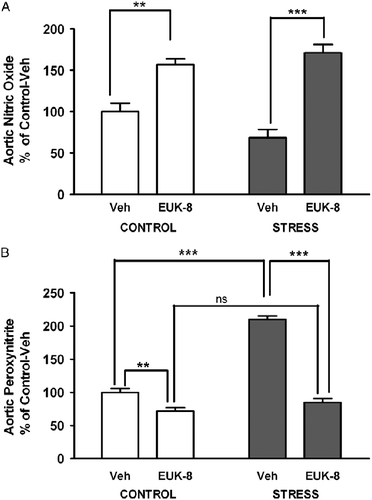
Peroxynitrite
Since superoxide can react with nitric oxide to generate peroxynitrite, we also compared the aortic root peroxynitrite formation in response to stress and treatment with EUK-8. There was a significant effect of group (df = 1, F = 127, p < 0.0001), treatment (df = 1, F = 196, p < 0.0001), and interaction (df = 1, F = 78, p < 0.0001). Post hoc test showed that similar to the effect of stress on reactive oxygen species, the stress/Veh group had approximately 100% more peroxynitrite than the control/Veh group (p < 0.001). EUK-8 treatment significantly decreased peroxynitrite amount in stressed mice compared to stress/Veh treatment (p < 0.001) and in control mice compared to control/Veh treatment (p < 0.01; ).
Effect of restraint stress and EUK-8 on aortic root LOX-1 expression
We also assessed aortic root LOX-1 expression. Immunohistochemistry showed that LOX-1 was expressed in the aortic root of all Apoe− / − mice, but there was a significant effect of group (df = 1, F = 103, p < 0.0001), treatment (df = 1, F = 15, p < 0.01), and interaction (df = 1, F = 12, p < 0.01). Post hoc test showed that stress (stress/Veh) increased LOX-1 expression by approximately 400% as compared to the control/Veh group (p < 0.001). Interestingly, EUK-8 reduced LOX-1 expression only in the stressed mice (p < 0.001), but this group still had more LOX-1 expression than the control/EUK-8 group (p < 0.001; ).
Figure 3 Effect of restraint stress on aortic root LOX-1 expression in Apoe− / − mice. Representative images showing LOX-1 expression in aortic root transverse sections from Apoe− / − mice. LOX-1 was detected using immunohistochemistry (green), and nuclei were visualized using DAPI (blue). Mice were either vehicle treated: control/Veh (n = 5) or stress/Veh (n = 4) or treated with EUK-8, an oxygen-derived free radical scavenger: control/EUK-8 (n = 5) or stress/EUK-8 (n = 5). Stressed mice were exposed to restraint stress for 2 h per day for 14 consecutive days. EUK-8 or vehicle was administered by intra-peritoneal injection every other day for 14 days. The lower panel shows a summary graph. Data are group means ± SEM. ***p < 0.001, post hoc Bonferroni tests.
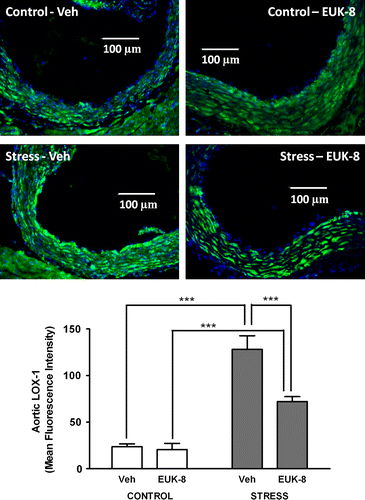
Discussion
The purpose of this study was to examine the effect of restraint stress on vascular oxidative stress and LOX-1 expression, which could lead to atherosclerosis. We first hypothesized that restraint stress would up-regulate LOX-1 expression and found that stress indeed increased LOX-1 expression in the aortic root. LOX-1 expression is low in the vasculature under normal physiological conditions, but is elevated in pro-oxidant disease states such as hypertension and diabetes (Kataoka et al. Citation1999; Chen et al. Citation2000b). The involvement of LOX-1 in the atherosclerotic process is well established, and LOX-1 is present in human atherosclerotic lesions (Li et al. Citation2002). The Apoe− / − mouse is a well-established mouse model for studies relating to atherosclerosis, and it has been shown that LOX-1 expression is greater at a pre-atherosclerotic stage in 8-week-old Apoe− / − mice than in wild-type mice on a normal chow diet. This suggests that LOX-1 plays an early role in the atherosclerotic process in Apoe− / − mice (Fisker Hag et al. Citation2008) as well as in humans. Indications of a causal role for LOX-1 in atherosclerosis have been provided by a study where over-expression of LOX-1 in Apoe− / − mice led to more atherosclerosis (Inoue et al. Citation2005). Evidence for an important role for LOX-1 in atherogenesis is further strengthened by the finding that genetic knockout of LOX-1 in another mouse model of atherosclerosis, the LDL receptor-deficient mouse, resulted in decreased atherosclerotic lesions (Mehta et al. Citation2007). Based on our results, it can be speculated that up-regulation of LOX-1 is one mechanism by which stress contributes to atherogenesis. Various environmental, social, or physiological factors can induce stress (increased allostatic load), and depending on the stressor, the sympathetic nervous system and the hypothalamic–pituitary–adrenal axis are activated to various degrees. Thus, it is important to note that the results seen in this study might be specific to the restraint stress response.
How did restraint stress lead to up-regulation of LOX-1? We hypothesized that a stress-induced increase in reactive oxygen species and/or peroxynitrite would up-regulate LOX-1. Thus, pharmacological scavenging of vascular oxygen-derived free radicals would decrease LOX-1 expression. In favor of our hypothesis, we found that stressed mice had more vascular reactive oxygen species including peroxynitrite. Furthermore, scavenging of oxygen-derived free radicals using EUK-8 reduced reactive oxygen species and LOX-1 expression. This indicates that either reactive oxygen species, i.e. superoxide and/or peroxynitrite, were indeed involved in the up-regulation of LOX-1 in stressed mice. Another antioxidant, tempol, has proven effective in reducing LOX-1 mRNA expression in cultured endothelial cells (Nagase et al. Citation2001). Indeed, recent studies have shown that peroxynitrite can induce the expression of LOX-1 in endothelial cells in culture (Sankaralingam et al. Citation2009a,Citationb). Therefore, peroxynitrite might have played a role in the up-regulation of LOX-1 in response to the psychological stress of repeated restraint.
In this study, we also found that, although EUK-8 reduced restraint-induced reactive oxygen species and peroxynitrite formation to that of control levels, it did not decrease LOX-1 expression to control levels. This indicates that pathways and factors other than oxygen-derived free radicals are also involved in the up-regulation of vascular LOX-1 in response to restraint stress. It has been reported that angiotensin II increases LOX-1 in cell culture, and that LOX-1 mRNA is down-regulated in mammary arteries of patients on long-term angiotensin-converting enzyme (ACE) inhibitors (Morawietz et al. Citation1999). Neurons can release angiotensin II during stimulation of the autonomic nervous system, and angiotensin II might therefore be involved in the up-regulation of LOX-1 in our study. Another possibility is that increased heart rate and blood pressure due to restraint stress contribute to higher shear stress in the region of the aortic root. Shear stress has been shown to increase the expression of LOX-1 in cell culture models (Murase et al. Citation1998). Moreover, LOX-1 expression can be increased by several other factors such as tumor necrosis factor-α (Nagase et al. Citation1997), which also signals through superoxide. Such downstream effects of various factors on reactive oxygen species production could have contributed to increased aortic root LOX-1 expression in response to stress.
In summary, this study demonstrates that restraint stress can lead to up-regulation of LOX-1 in the vasculature, in part via the formation of oxygen-derived free radicals. Therefore, the formation of reactive oxygen species and peroxynitrite and subsequent up-regulation of LOX-1 might be one mechanism by which psychological stress contributes to create pro-atherosclerotic conditions.
Acknowledgements
The authors thank Dr Yan-Yan Jiang for technical assistance. Grants from the Canadian Institutes for Health Research (CIHR) supported this work. The CIHR training programme in Maternal–Fetal–Newborn Health supported I. J. Andersson and S. Sankaralingam. Strategic Training Initiative in Research in Reproductive Health Sciences Training Programs of the CIHR, the Alberta Heritage Foundation for Medical Research (AHFMR), and the Junior Personnel Award from the Heart and Stroke Foundation of Canada also supported S. Sankaralingam. S. T. Davidge is an AHFMR Scientist and a Canada Research Chair in Women's Cardiovascular Health.
Declaration of interest: The authors report no conflicts of interest. The authors alone are responsible for the content and writing of the paper.
References
- Bauer ME, Jeckel CM, Luz C. 2009. The role of stress factors during aging of the immune system. Ann N Y Acad Sci. 1153:139–152.
- Chen H, Li D, Sawamura T, Inoue K, Mehta JL. Upregulation of LOX-1 expression in aorta of hypercholesterolemic rabbits: Modulation by losartan. Biochem Biophys Res Commun. 2000a; 276:1100–1104.
- Chen M, Kakutani M, Minami M, Kataoka H, Kume N, Narumiya S, Kita T, Masaki T, Sawamura T. Increased expression of lectin-like oxidized low density lipoprotein receptor-1 in initial atherosclerotic lesions of watanabe heritable hyperlipidemic rabbits. Arterioscler Thromb Vasc Biol. 2000b; 20:1107–1115.
- Chen M, Kakutani M, Naruko T, Ueda M, Narumiya S, Masaki T, Sawamura T. 2001. Activation-dependent surface expression of LOX-1 in human platelets. Biochem Biophys Res Commun. 282:153–158.
- Chen M, Masaki T, Sawamura T. 2002. LOX-1, the receptor for oxidized low-density lipoprotein identified from endothelial cells: Implications in endothelial dysfunction and atherosclerosis. Pharmacol Ther. 95:89–100.
- Cominacini L, Rigoni A, Pasini AF, Garbin U, Davoli A, Campagnola M, Pastorino AM, Lo Cascio V, Sawamura T. 2001. The binding of oxidized low density lipoprotein (ox-LDL) to ox-LDL receptor-1 reduces the intracellular concentration of nitric oxide in endothelial cells through an increased production of superoxide. J Biol Chem. 276:13750–13755.
- Draude G, Hrboticky N, Lorenz RL. 1999. The expression of the lectin-like oxidized low-density lipoprotein receptor (LOX-1) on human vascular smooth muscle cells and monocytes and its down-regulation by lovastatin. Biochem Pharmacol. 57:383–386.
- Epel ES, Blackburn EH, Lin J, Dhabhar FS, Adler NE, Morrow JD, Cawthon RM. 2004. Accelerated telomere shortening in response to life stress. Proc Natl Acad Sci USA. 101:17312–17315.
- Fisker Hag AM, Pedersen SF, Kjaer A. 2008. Gene expression of LOX-1, VCAM-1, and ICAM-1 in pre-atherosclerotic mice. Biochem Biophys Res Commun. 377:689–693.
- Inoue K, Arai Y, Kurihara H, Kita T, Sawamura T. 2005. Overexpression of lectin-like oxidized low-density lipoprotein receptor-1 induces intramyocardial vasculopathy in apolipoprotein E-null mice. Circ Res. 97:176–184.
- Kataoka H, Kume N, Miyamoto S, Minami M, Moriwaki H, Murase T, Sawamura T, Masaki T, Hashimoto N, Kita T. 1999. Expression of lectinlike oxidized low-density lipoprotein receptor-1 in human atherosclerotic lesions. Circulation. 99:3110–3117.
- Kim KS, Han PL. 2006. Optimization of chronic stress paradigms using anxiety- and depression-like behavioral parameters. J Neurosci Res. 83:497–507.
- Li DY, Chen HJ, Staples ED, Ozaki K, Annex B, Singh BK, Vermani R, Mehta JL. 2002. Oxidized low-density lipoprotein receptor LOX-1 and apoptosis in human atherosclerotic lesions. J Cardiovasc Pharmacol Ther. 7:147–153.
- Mehta JL, Sanada N, Hu CP, Chen J, Dandapat A, Sugawara F, Satoh H, Inoue K, Kawase Y, Jishage K, Suzuki H, Takeya M, Schnackenberg L, Beger R, Hermonat PL, Thomas M, Sawamura T. 2007. Deletion of LOX-1 reduces atherogenesis in LDLR knockout mice fed high cholesterol diet. Circ Res. 100:1634–1642.
- Morawietz H, Rueckschloss U, Niemann B, Duerrschmidt N, Galle J, Hakim K, Zerkowski HR, Sawamura T, Holtz J. 1999. Angiotensin II induces LOX-1, the human endothelial receptor for oxidized low-density lipoprotein. Circulation. 100:899–902.
- Murase T, Kume N, Korenaga R, Ando J, Sawamura T, Masaki T, Kita T. 1998. Fluid shear stress transcriptionally induces lectin-like oxidized LDL receptor-1 in vascular endothelial cells. Circ Res. 83:328–333.
- Nagase M, Hirose S, Sawamura T, Masaki T, Fujita T. 1997. Enhanced expression of endothelial oxidized low-density lipoprotein receptor (LOX-1) in hypertensive rats. Biochem Biophys Res Commun. 237:496–498.
- Nagase M, Ando K, Nagase T, Kaname S, Sawamura T, Fujita T. 2001. Redox-sensitive regulation of LOX-1 gene expression in vascular endothelium. Biochem Biophys Res Commun. 281:720–725.
- Nakashima Y, Plump AS, Raines EW, Breslow JL, Ross R. 1994. Apoe-deficient mice develop lesions of all phases of atherosclerosis throughout the arterial tree. Arterioscler Thromb. 14:133–140.
- Nation DA, Gonzales JA, Mendez AJ, Zaias J, Szeto A, Brooks LG, Paredes J, D'Angola A, Schneiderman N, McCabe PM. 2008. The effect of social environment on markers of vascular oxidative stress and inflammation in the watanabe heritable hyperlipidemic rabbit. Psychosom Med. 70:269–275.
- Sankaralingam S, Xu Y, Sawamura T, Davidge ST. Increased lectin-like oxidized low-density lipoprotein receptor-1 expression in the maternal vasculature of women with preeclampsia: Role for peroxynitrite. Hypertension. 2009a; 53:270–277.
- Sankaralingam S, Xu H, Jiang Y, Sawamura T, Davidge ST. Evidence for increased methylglyoxal in the vasculature of women with preeclampsia: Role in upregulation of LOX-1 and arginase. Hypertension. 2009b; 54:897–904.
- Sawamura T, Kume N, Aoyama T, Moriwaki H, Hoshikawa H, Aiba Y, Tanaka T, Miwa S, Katsura Y, Kita T, Masaki T. 1997. An endothelial receptor for oxidized low-density lipoprotein. Nature. 386:73–77.
- Sivonova M, Zitnanova I, Hlincikova L, Skodacek I, Trebaticka J, Durackova Z. 2004. Oxidative stress in university students during examinations. Stress. 7:183–188.
- Yoshida H, Kondratenko N, Green S, Steinberg D, Quehenberger O. 1998. Identification of the lectin-like receptor for oxidized low-density lipoprotein in human macrophages and its potential role as a scavenger receptor. Biochem J. 334 Pt 1: 9–13.