Abstract
Methamphetamine (MA) induces multiple effects in rats including alterations to corticosterone (CORT) and adrenocorticotropic hormone (ACTH). This effect is age dependent showing a U-shaped function similar to that of other stressors during the stress hyporesponsive period. Neonatal MA treatment leads to adult learning and memory impairments, but whether these are related to MA-induced CORT release is unknown. Here in, four methods were tested in neonatal rats previously established in adult rats for inhibiting stress-induced CORT release: inhibiting synthesis (metyrapone (MET) or ketoconazole (KTZ)) or surgically by adrenalectomy or adrenal autotransplantation (ADXA). Pretreatment on postnatal day 11 with MET or KTZ prior to four doses of 10 mg/kg of MA initially suppressed MA-induced increases in plasma CORT, but 24 h later, even with additional inhibitor treatment, a large CORT increase was seen which exceeded that of MA alone. Adrenalectomy blocked MA-induced increases in CORT but caused a secondary effect on brain serotonin (5-HT) and dopamine (DA), causing greater reductions than those caused by MA alone. ADXA inhibited MA-induced CORT release without causing a 24-h CORT increase and did not produce additional effects on brain 5-HT or DA. Neonatal ADXA is a new model for developmental drug or stress experiments designed to test the role of CORT in mediating early effects on later outcomes.
Introduction
Methamphetamine (MA) is an addictive drug known to be abused even during pregnancy (Smith et al. Citation2003; Garcia-Bournissen et al. Citation2007). Case–control studies of newborns, infants, and children suggest cognitive and other central nervous system (CNS) effects following human fetal MA exposure based on observations of reduced performance on the Fagan test of infant intelligence, reduced sustained attention, delayed verbal memory, decreased spatial ability, reduced brain volume, and increased physiological stress (Struthers and Hansen Citation1992; Smith et al. Citation2003, Citation2008; Chang et al. Citation2004, Citation2009; Cloak et al. Citation2009). In the rat following MA exposure from postnatal days (P)11–20, a period analogous to second and third trimester human fetal brain development (Bayer et al. Citation1993; Clancy et al. Citation2007), we have shown significant spatial and egocentric learning deficits in the Morris and Cincinnati water mazes, respectively (Vorhees et al. Citation2000, Citation2008; Williams et al. Citation2002, Citation2003a,Citationb; Skelton et al. Citation2007). The mechanism by which MA produces these deficits may be related to its ability to induce prolonged activation of the hypothalamic–pituitary–adrenal (HPA) axis (Williams et al. Citation2000a, Citation2006; Schaefer et al. Citation2006, Citation2008; Grace et al. Citation2008).
The adrenal gland releases corticosterone (CORT) which can then act on glucocorticoid and mineralocorticoid receptors in the brain and interfere with neuronal proliferation, especially in the hippocampus (Woolley et al. Citation1990; Gould et al. Citation1991; Liu et al. Citation2003). The hippocampus is involved in learning and memory (McNaughton et al. Citation1989; Squire and Zola Citation1998), and neurogenesis continues in this region throughout gestation in humans and postnatally in the rat (Bayer et al. Citation1993; Clancy et al. Citation2007). The interaction of increased glucocorticoid release and subsequent effects on glucocorticoid receptors has been hypothesized to mediate long-term stress-induced behavioral disruptions (de Kloet et al. Citation1998; Oitzl et al. Citation2001; Roozendaal et al. Citation2006). Elevations in CORT that occur during the stress hyporesponsive period (∼postnatal day (P)4–14) may be salient because basal CORT levels are normally low during this period and exhibit reduced responses to stress, an adaptation thought to be protective for hippocampal granule cell proliferation (Vazquez Citation1998). Increases in CORT or stress during this period can result in later learning impairments (Aisa et al. Citation2007).
MA (10 mg/kg) has been shown to produce an increase in CORT when administered between P1 and 19 in rats (Williams et al. Citation2006). On P11, MA-treated rats (10 mg/kg × 4) show threefold sustained increases in plasma CORT for at least 24 h compared to saline (SAL)-treated rats (Schaefer et al. Citation2006). Repeated MA treatment from P11 to 15 also causes CORT increase that lasts at least until P16 (Schaefer et al. Citation2008). MA treatment between P11 and 15 compared to forced swim or isolation shows that MA induces significantly greater release of CORT than these stressors (Grace et al. Citation2008). The increase in CORT following neonatal MA may play a role in the observed decrease in spines per neuron in the dentate gyrus and serotonin (5-HT) decreases in the hippocampus and neostriatum observed following P11–20 MA treatment (Williams et al. Citation2004; Schaefer et al. Citation2006, Citation2008).
In addition to CORT, 5-HT is also known to be important in neuronal development. 5-HT is abundant in the brain during neurogenesis and is reduced by neonatal MA treatment. 5-HT is important for the differentiation of neurons and receptive target cells, and is involved in organizing the developing brain (Lauder and Krebs Citation1978; Lauder Citation1993). Multiple days of MA treatment during early development (P11–15 and P11–20) reduces 5-HT in various brain regions (Schaefer et al. Citation2008). Consistent with this, P10–20 treatment with the tryptophan hydroxylase inhibitor p-chlorophenylalanine also reduces brain 5-HT and leads to learning and memory deficits (Mazer et al. Citation1997).
In order to understand the role of neonatal CORT release in MA-treated rats, experiments were undertaken to inhibit CORT synthesis or block release. A series of experiments were required because the effect of MA on the adrenal proved difficult to inhibit.
Materials and methods
Animals and housing
Nulliparous female Sprague–Dawley CD (International Genetic Strain) rats (Charles River Laboratories, Raleigh, NC, USA) were mated with males of the same strain and supplier. Rats were housed in a 22 ± 1°C environment at 50% humidity with a 14/10 h light/dark cycle (lights on at 06:00 h). Prior to mating, animals were allowed at least 1 week to acclimate to the vivarium. Females spent 2 weeks housed with a male in a hanging wire cage in order to identify sperm plugs beneath the breeding cage. Once pregnant, females were housed singly in polycarbonate cages (46 × 24 × 20 cm) containing wood chip bedding and ad libitum food and water. The progeny were used as subjects. The Institutional Animal Care and Use Committee approved all procedures, and the vivarium was accredited by the Association for the Assessment and Accreditation of Laboratory Animal Care (AAALAC).
The day of birth was considered P0, and on P1, litters were culled to 8–12 male pups except in Experiment 7, in which litters contained both sexes although only males were used. We have previously shown no reliable sex effects following neonatal drug treatment, but to minimize variation, males were retained preferentially. No significant differences between males and females in adrenal–pituitary hormone levels were noted following P11 MA treatment (Williams et al. Citation2000b). On P7, pups were weighed, individually identified by ear punch, and randomly assigned to treatment groups.
Dosing procedures
(+)-MA HCl (NIH, NIDA, Bethesda, MD, USA) was administered at a dose of 10 mg/kg (expressed as the freebase; purity >95%) in a volume of 3 ml/kg dissolved in isotonic SAL. Two CORT synthesis inhibitors were tested, metyrapone (MET) and ketoconazole (KTZ). MET (Sigma, St Louis, MO, USA) was administered at either 50 or 100 mg/kg in a volume of 3 ml/kg and was dissolved in either 25% dimethyl sulfoxide (DMSO)/75% SAL (MET-D) or 10% ethanol/90% SAL (MET-E) (Roozendaal et al. Citation2006). KTZ (Sigma; 25 or 50 mg/kg) was administered in a volume of 6 ml/kg, and was dissolved in 100% corn oil (KTZ-O) (Smagin and Goeders Citation2004). The vehicle (VEH) used in each experiment corresponded with the pretreatment diluents used [DMSO (VEH-D), ethanol (VEH-E), or corn oil (VEH-O)]. Each dose was administered by subcutaneous injection in the dorsum.
Treatment/dosing regimens
Experiment 1
P11 rats were treated with MET prior to the first of four MA doses (10 mg/kg/dose). Ten litters were used (eight male pups/litter). Two pups were assigned to each of four groups: (1) MET 50 mg/kg in DMSO (MET50-D)+MA, (2) MET 100 mg/kg in DMSO (MET100-D)+MA, (3) DMSO (VEH-D)+MA, and (4) DMSO (VEH-D)+SAL. The pretreatments (MET50-D, MET100-D, and VEH-D) were administered 90 min prior to MA or SAL. Drug injections were administered once every 2 h for 6 h. One pup from each treatment group was killed 30 min after each of the four drug doses (i.e. time: 30, 150, 270, and 390 min after the first dose). Plasma was collected and analyzed for CORT.
Experiment 2
In Experiment 1, CORT levels appeared to be affected by DMSO; therefore, in this experiment, the effects of DMSO were tested. There were six litters used with 12 male pups/litter. The number of animals used in this and subsequent experiments was different to ensure that each treatment was represented within each litter. Groups were (1) weighed at the pretreatment time and weighed at the drug treatment time points (WEIGH+WEIGH), (2) SAL+SAL, (3) VEH-D+SAL, and (4) MET100-D+SAL. On P11, the pretreatment injections were given 90 min prior to SAL as previously described followed by four SAL injections at 2-h intervals. Blood was collected after the first SAL dose at 30, 270, or 390 min. Plasma was collected and analyzed for CORT.
Experiment 3
Based on the outcome of Experiment 2, the effectiveness of MET in a 10% VEH-E on the suppression of CORT following P11 MA treatment was determined. Litters were culled to 10 male pups, and eight litters were used. Within each litter, there were five treatments: (1) MET 100 mg/kg in 10% VEH-E (MET100-E)+MA, (2) 10% VEH-E+MA, (3) VEH-E+SAL, (4) SAL+MA, and (5) SAL+SAL. The pretreatment and MA doses were as in the previous experiments. As before, 30 min after the first or fourth dose, blood was collected and plasma was analyzed for CORT.
Experiment 4
Once the short-term inhibitory effects of MET in VEH-E were known, we then examined the ability of MET100-E to attenuate CORT increases over a longer period of time (24 h). Eight litters were used, and litters were culled to eight male pups and divided into four treatment groups similar to Experiment 3, i.e. (1) MET100-E+MA, (2) MET100-E+SAL, (3) VEH-E+MA, and (4) VEH-E+SAL. As in the previous experiments, the pretreatment was administered 90 min prior to MA or SAL. MA treatment was administered every 2 h for 6 h, and plasma was collected 24 h after the first dose and analyzed for CORT.
Experiment 5
Because MET was ineffective 24 h later at blocking CORT increases, KTZ was used to determine whether blocking the production of adrenal androgen biosynthesis by inhibition of C17, 20-desmolase would be effective (Weber et al. Citation1993). On P1, litters were culled to 12 male pups. Eight litters were used with littermates assigned to one of the four treatment groups: (1) KTZ (25 mg/kg) in VEH-O (KTZ25-O)+MA; (2) KTZ (50 mg/kg) in VEH-O (KTZ50-O)+MA; (3) VEH-O+MA; and (4) VEH-O+SAL. The pretreatments (KTZ25-O, KTZ50-O, or VEH-O) were administered 60 min prior to the first MA dose. As in previous experiments, MA was administered once every 2 h for 6 h. Offspring were killed at 1, 7, or 24 h following the first dose of MA, and blood was collected and analyzed for CORT.
Experiment 6
Based on the CORT level, rebound effects were observed 24 h after synthesis inhibitors were given; the next approach investigated was adrenalectomy. Seven litters were culled to 10 male pups in which four animals/litter on P10 were adrenalectomized (ADX), 4 were sham operated (SHAM), 1 pup did not undergo surgery (see below), and 1 pup was not used. The treatments for this experiment were as follows: (1) ADX+MA; (2) ADX+SAL; (3) SHAM+MA; (4) SHAM+SAL; and (5) SAL ONLY. The animals received MA or SAL once every 2 h over a 6-h period on P11. Blood was collected for CORT determination on P12, 24 h after the first MA dose as in Experiments 4 and 5. ACTH was examined in this study to ensure that the ADX was complete because an increase in ACTH would be expected in ADX animals. The neostriatum and hippocampus were collected and analyzed for monoamines to determine if ADX+MA interacted.
Experiment 7
Because of the unexpected effect of ADX+MA on 5-HT, another method was tested. In this approach, adrenals were excised and then replaced in the body cavity in a technique called adrenal autotransplantation (ADXA). ADXA was performed on P9 to give pups an additional day to recover from the surgical procedure before administering MA on P11. Ten litters of 10 mixed sex pups were used; however, only four males were treated as follows: (1) ADXA+MA; (2) ADXA+SAL; (3) SHAM+MA; and (4) SHAM+SAL. As in previous experiments, MA was administered every 2 h, and blood was collected 24 h after the first MA dose and later assayed for CORT. The hippocampus was assayed for 5-HT and 5-hydroxyindoleacetic acid (5-HIAA), and the neostriatum for 5-HT, 5-HIAA, dopamine (DA), and 3,4-dihydroxyphenylacetic acid (DOPAC).
Adrenal surgery
Prior to drug treatment, adrenal glands were removed via a dorsal route for Experiments 6 and 7. In Experiment 6, the adrenals were completely removed, whereas in Experiment 7, after excising the adrenals, they were placed back in the peritoneum for autoengraftment. ADXA was adapted from Okamoto et al. (Citation1992a). Our procedures differed, in that specific placement of the adrenals under the cutaneous maximus was not performed, and antibiotics were not given following the procedure. In both experiments, rats were transported in their home cage to a surgical suite, and half of the litter were subjected to ADX (Experiment 6) or ADXA (Experiment 7), and the other half were subjected to a sham operation, in which incisions were made, and the adrenals were located, but left attached. The dorsum was swabbed with 70% ethanol and Betadine prior to surgery, and all animals were anesthetized with isoflurane prior to and during surgery. After surgery, the wound was sutured, the dermis stapled, and the dorsum swabbed with warm SAL, and then the subjects were returned to the home cage.
Blood and tissue collection
Each animal was euthanized by decapitation. Blood was collected in tubes containing 2% EDTA (0.05 ml) and centrifuged (1399 Relative centrifugal force) for 25 min at 4°C. Plasma was collected and stored at − 80°C until assayed. In Experiments 6 and 7, the brain was removed from each animal, and an ice-chilled brain block (Zivic-Miller, Pittsburgh, PA, USA) was used to aid in dissection of the neostriatum (caudate and putamen) and hippocampus. For the neostriatum, a coronal cut was made at the optic chiasm and another cut was made 2 mm rostral to the first. The neostriatum was dissected from this 2-mm slab. Hippocampi were removed from the remaining tissue bilaterally. All dissections were done on a chilled dissection plate, and tissues were frozen on dry ice. Tissue weights were determined by placing the tissues in pre-weighed tubes and then by re-weighing the tubes. All tissues were stored at − 80°C until monoamines were quantified by high-pressure liquid chromatography with electrochemical detection.
Hormone assessment
CORT (OCTEIA CORT kit: Experiments 1–6: ALPCO Diagnostics, Windham, NH, USA; Experiment 7: IDS, Fountain Hills, AZ, USA) and ACTH (Peninsula Laboratories, Inc., San Carlos, CA, USA) in plasma were assayed in duplicate using a commercially available EIA. Plasma was diluted 3:1 for CORT and 4:1 for ACTH prior to analysis.
Monoamine determination
The tissue concentrations of DA, DOPAC, 5-HT, and 5-HIAA in the neostriatum and 5-HT and 5-HIAA in the hippocampus were quantified using high-pressure liquid chromatography with electrochemical detection. Tissues were homogenized in 50 volumes of 0.2 M perchloric acid and centrifuged for 6 min at 10,000g. Aliquots of 20 μl were injected into a C18-column (MD-150, 3 × 150 mm; ESA, Chelmsford, MA, USA) connected to either a LC-4B amperometric detector (Bioanalytical Systems, West Lafayette, IN, USA) or a Coulochem detector (25 A: ESA, Chelmsford, MA, USA), and an integrator recorded the peak heights that followed each injection. The potential for the LC-4B was 0.6 V, and the potentials of E1 and E2 on the analytical cell (model 5014B) of the Coulochem were − 150 and 160 mV, respectively. The mobile phases consisted of 35 mM citric acid, 54 mM sodium acetate, 50 mg/l of disodium ethylenedeamine tetraacetate, 70 mg/l of octanesulfonic acid sodium salt, 6% (v/v) methanol, and 6% (v/v) acetonitrile, pH 4.0, and pumped at a flow rate of 0.4 ml/min. Quantities of the analytes were calculated on the basis of known standards. Retention times for DOPAC, DA, 5-HIAA, and 5-HT were approximately 6, 8, 11, and 17 min, respectively.
Statistics
Data were analyzed using mixed linear ANOVA models (SAS Proc Mixed, SAS Institute, Cary, NC, USA), and depending upon the design, models were either two-way ANOVAs with treatment and time as main effects (Experiments 1–3 and 5), two-way ANOVAs with pretreatment and treatment as main effects (Experiment 4), or two-way ANOVAs with surgery and treatment as main effects (Experiments 6 and 7). The covariance matrix for each data set was checked using best fit statistics to determine the better fit of the variance–covariance structure. Kenward–Roger-adjusted degrees of freedom were used and can be fractional. Only drug treatment and surgery main effects and interaction F-ratios are shown for clarity of data presentation. The Tukey–Kramer method was used for a posteriori pairwise group comparison. Significance was set at p ≤ 0.05; trends were noted at p ≤ 0.10.
Results
Experiment 1
Following pretreatments of MET50-D or MET100-D, CORT samples obtained 30 min after each of the MA drug doses showed main effects of Treatment (F(3,205) = 58.01, p < 0.0001) and Time (p < 0.0001; ). There was no interaction of Treatment × Time. CORT levels were similar for the treatments between the 30 and 150 min time points, but were significantly increased at each subsequent time point compared to those of the previous time points (). Tukey–Kramer post-hoc analysis showed that CORT levels, regardless of time, were significantly elevated in both the VEH-D+MA and MET50-D+MA groups compared to those of VEH-D+SAL group ( inset). The MET100-D+MA group was not statistically different from the VEH-D+SAL control group.
Figure 1. CORT concentrations in plasma 30 min after each drug (MA or SAL) dose on P11 following MET+DMSO pretreatment. CORT levels were significantly elevated at all subsequent times except between the 30 and 150 min time points. Inset: Means of CORT levels for each treatment group regardless of kill time. CORT levels were significantly elevated in the VEH-D+MA and MET50-D+MA groups compared to those in the VEH-D+SAL control group. Ten animals/treatment were used. *p < 0.05 from VEH-D+SAL control group.
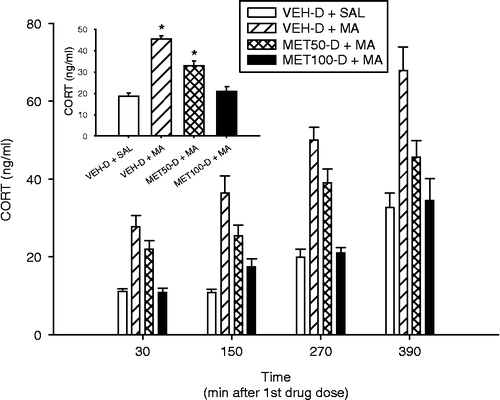
Experiment 2
Because of the progressive increase in CORT in the VEH-D+SAL group over time in Experiment 1, in Experiment 2, the effect of DMSO was evaluated. There was a significant main effect of Treatment (F(3,55) = 5.97, p < 0.001; inset) and Time (p < 0.0003), and there was a trend for a Treatment × Time interaction (F(6,55) = 1.91, p < 0.1; ). Regardless of time, CORT levels in the VEH-D+SAL group were significantly elevated compared to those in the WEIGH+WEIGH group, and there was a trend for CORT to be increased compared to the SAL+SAL group; CORT levels in the MET100-D+SAL group were significantly different from the SAL+SAL and WEIGH+WEIGH groups, but not from the VEH-D+SAL group. There was no difference between the SAL+SAL and the WEIGH+WEIGH groups. CORT levels, regardless of treatment, were significantly elevated at 390 min (p < 0.05) with a trend at 270 min (p < 0.1) compared to those at 30 min, but there was no difference between the levels at 270 and 390 min.
Figure 2. CORT levels in plasma 30 min after the first, third, and fourth drug (SAL) doses on P11 following a MET in DMSO pretreatment. CORT values regardless of treatment were increased at 390 min, and there was a trend for CORT to be increased at 270 min compared to 30 min. Inset: Means of CORT levels for each treatment group without regard to time. CORT was increased in the MET100-D+SAL group compared that in to both SAL+SAL and WEIGH+WEIGH groups. In the VEH-D+SAL group, CORT was significantly elevated compared to the WEIGH+WEIGH group, and there was a trend for CORT to be increased compared to the SAL+SAL group. Six animals/treatment were used. *p < 0.05 from SAL+SAL group, %p < 0.05 from WEIGH+WEIGH group, and †p < 0.1 from SAL+SAL group.
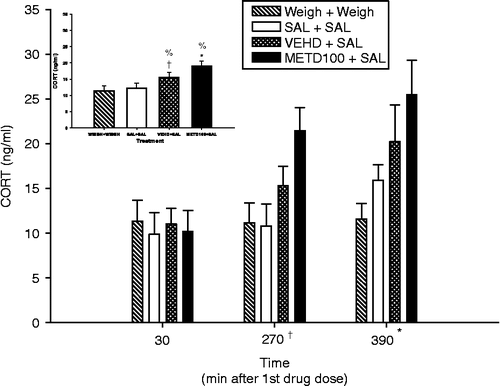
Experiment 3
Because CORT levels were elevated following VEH-D+SAL and MET100-D+SAL treatments in Experiment 2, and pups showed agitation following DMSO injection, MET was dissolved in a 10% VEH-E. The effect of MET in blocking MA-induced CORT increases was again examined. There were significant effects of Treatment (F(4,45) = 12.22, p < 0.0001), Time (p < 0.0001), and Treatment × Time (F(4,45) = 3.36, p < 0.02; ). CORT levels at both time points (30 and 390 min) were significantly elevated in the SAL+MA and VEH-E+MA groups, but not in the MET100-E+MA groups compared to the SAL+SAL controls. The VEH-E+SAL treatment produced no increased CORT compared to the SAL+SAL group. CORT levels overall, regardless of treatment, were significantly elevated at the 390 min time point compared to the 30 min time point.
Figure 3. CORT values following pretreatment with MET in ethanol and drug doses (MA or SAL) 30 or 390 min after the first drug dose on P11. CORT levels, regardless of treatment, were increased at the 390-min time point compared to those at the 30-min time point. At both times, the SAL+MA and the VEH-E+MA groups had elevated CORT values compared to SAL+SAL controls. Eight animals/treatment were used. *p < 0.05 from SAL+SAL and VEHE+SAL control groups.
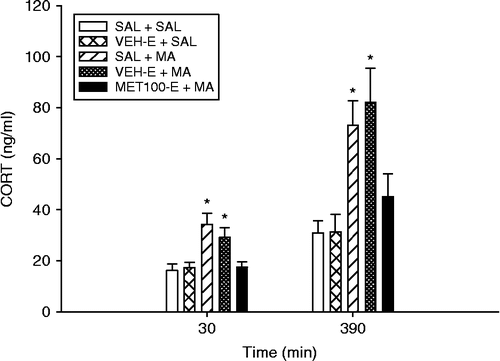
Experiment 4
Since we previously showed that MA can increase CORT levels for at least 24 h (Schaefer et al. Citation2006), in Experiment 4, we examined the protracted effect of MET100-E pretreatment on MA-induced CORT increase at 24 h. There were main effects of Pretreatment (F(1,16) = 7.04, p < 0.02) and Treatment (F(1,16) = 24.03, p < 0.0002), and an interaction of Pretreatment × Treatment (F(1,16) = 4.99, p < 0.04; ). Analysis of the interaction using simple effect tests demonstrated that CORT levels were elevated in the MET100-E+MA-treated group compared to those in the VEH-E+SAL-, VEH-E+MA-, and MET100-E+SAL-treated groups. In addition, the VEH-E+MA group showed a significant increase in CORT levels compared to the VEH-E+SAL group (p < 0.05, one-tailed).
Figure 4. Plasma CORT following a pretreatment of MET100-E or VEH-E and MA 24 h after the first MA dose on P11 (killed on P12). CORT levels were statistically elevated in the MET100-E+MA-treated animals compared to those of all other treatments. In addition, the VEH-E+MA animals had higher CORT values than the VEH-E+SAL animals. Eight animals/treatment were used. *p < 0.05 from the VEH-E+SAL animals; #p < 0.05 from all other treatments.
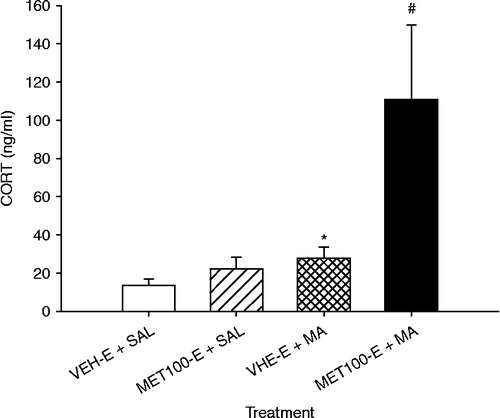
Experiment 5
Because there was an apparent CORT rebound increase in the MET100-E+MA group at 24 h in Experiment 4, in this experiment, we pretreated with KTZ-O to block the formation of CORT at an earlier stage in the synthetic pathway. There were effects of Treatment (F(3,74) = 4.10, p < 0.01), Time (p < 0.001), and Time × Treatment (F(6,74) = 4.11, p < 0.001; ). CORT levels increased significantly at each successive time point. Post-hoc analysis showed that CORT levels were significantly increased at 1 h after the first dose in the VEH-O+MA, KTZ25-O+MA, and KTZ50-O+MA groups compared to those of the VEH-O+SAL group. At 7 h, the VEH-O+MA and KTZ25-O+MA groups had elevated CORT levels compared to the VEH-O+SAL and KTZ50-0+MA groups. At 24 h, the KTZ25-O+MA, KTZ50-O+MA, and VEH-O+MA (p < 0.05, one-tailed) groups had elevated CORT compared to the VEH-O+SAL group.
Figure 5. CORT values following a KTZ-O pretreatment and MA drug exposure at the 1-, 7-, and 24-h time points after the first dose on P11. CORT levels increased significantly at each successive time point. At the 1-h time point, all groups had significantly elevated CORT levels compared to the VEH-O+SAL control group. At the 7-h time point, the VEH-O+MA and the KTZ25-O+MA groups had increased CORT compared to the VEH-O+SAL control group, but there was no difference between the VEH-O+SAL group and the KTZ50-O+MA group. At the 24-h time point, the VEH-O+MA group and both groups that received the KTZ-O pretreatment had elevated CORT relative to the VEH-O+SAL control group. Eight animals/treatment were used. *p < 0.05 from the VEH-O+SAL control group.
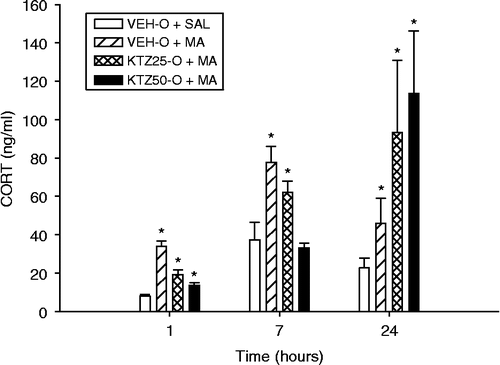
Experiment 6
Since CORT levels showed rebound effects after 24 h, this experiment examined the effect of ADX in conjunction with MA. For CORT, there were effects of Treatment (F(1,18) = 21.75, p < 0.0002), Surgery (F(1,18) = 31.54, p < 0.0001), and Surgery × Treatment (F(1,18) = 20.01, p < 0.0003; ). CORT levels were significantly increased in the SHAM+MA group compared to those of all other groups. CORT levels were significantly lower in the ADX+SAL animals compared to those of the SHAM+SAL animals.
Figure 6. CORT (A) and ACTH (B) values following ADX or SHAM pretreatment and MA or SAL 24 h after the first drug dose on P11 (killed on P12). CORT (A) levels were significantly higher in the SHAM+MA compared to those in all other groups. The ADX+SAL group had lower CORT levels than the SHAM+SAL group. ACTH (B) values were significantly higher in both ADX+SAL and ADX-MA groups compared to those in SHAM+SAL and SHAM+MA groups, respectively. Seven animals/treatment were used. *p < 0.05 from SHAM+same drug.
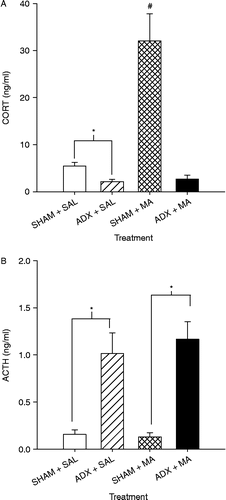
ACTH was significantly affected by surgery (F(1,18) = 41.33, p < 0.0001; ) but not by treatment. Regardless of drug treatment, ACTH levels in ADX animals were elevated compared to those in SHAM animals (). There was no interaction of Treatment × Surgery.
Hippocampal 5-HT was significantly affected by Treatment (F(1,18) = 10.46, p < 0.005; ), and in the interaction of Surgery × Treatment (F(1,18) = 2.08, p < 0.005); however, there was no main effect of ADX. Post-hoc analysis showed a small 5-HT increase in the ADX+SAL group compared to the SHAM+SAL group and a substantial decrease in the ADX+MA animals compared to the ADX+SAL animals, but no difference between SHAM+SAL and SHAM+MA animals. There was a trend for 5-HT to be decreased in the ADX+MA animals compared to SHAM+MA animals.
Figure 7. Hippocampal and neostriatal monoamines following ADX or SHAM surgery and MA or SAL 24 h after the first drug dose on P11 (killed on P12). 5-HT in the hippocampus (A) was significantly increased in the ADX+SAL animals compared to that in the SHAM+SAL animals. The ADX+MA group had decreased hippocampal 5-HT compared to the ADX+SAL group, and there was a trend for 5-HT to be decreased in the ADX+MA animals compared to that in the SHAM+MA animals. 5-HIAA in the hippocampus (B) was significantly decreased by ADX in the MA-treated animals compared to that in the ADX+SAL animals and a tendency to be decreased compared to that in the SHAM+MA animals. 5-HT (C) and 5-HIAA (D) in the neostriatum were significantly decreased in MA-treated animals regardless of surgery compared to those in SAL-treated animals. *p < 0.05 from SAL. Neostriatal DA (E) was significantly decreased in the ADX-MA group compared to that in the SHAM-MA group, and the SHAM+MA group had DA elevations compared to SHAM+SAL group. Neostriatal DOPAC (F) was significantly decreased by ADX in the MA-treated animals compared to that in SHAM animals and ADX+SAL animals. *p < 0.05; †p < 0.1.
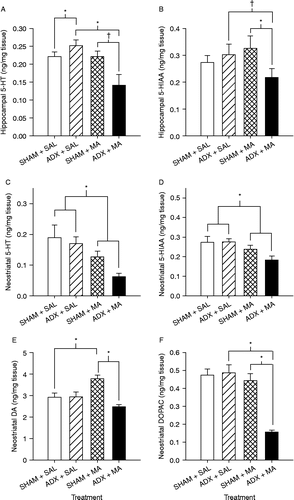
5-HIAA in the hippocampus showed a Surgery × Treatment interaction (F(1,17) = 6.58, p < 0.02) but no significant main effects of treatment or surgery (). Post-hoc analysis showed decreased 5-HIAA levels in the ADX+MA group compared to the SHAM+MA group. There was a trend for 5-HIAA to be decreased in the ADX+MA group compared to the ADX+SAL group. No other differences were noted.
In the neostriatum, 5-HT (F(1,18) = 11.99, p < 0.003) and 5-HIAA (F(1,18) = 13.33 p < 0.002) demonstrated significant main effects of Treatment (, respectively). MA-treated animals regardless of surgery had significantly lower levels of 5-HT and 5-HIAA than SAL-treated animals. No interaction of treatment and surgery was observed.
DA in the neostriatum showed a main effect of surgery (F(1,18) = 13.06, p < 0.002) and a Surgery × Treatment interaction (F(1,18) = 13.78, p < 0.002), but no main effect of treatment. Simple effect analysis of the interaction revealed a significant decrease in DA levels in the ADX+MA group compared to the SHAM+MA group and an increase in the SHAM+MA group compared to the SHAM+SAL group ().
DOPAC in the neostriatum showed effects of Treatment (F(1,18) = 30.31, p < 0.0001), and Surgery (F(1,18) = 17.51, p < 0.0006), and a Surgery × Treatment interaction (F(1,18) = 20.96, p < 0.0002). Post-hoc analysis showed that the ADX+MA group had significantly lower DOPAC levels than animals in the SHAM+MA and ADX+SAL groups ().
Experiment 7
Because animals in Experiment 6 showed an interaction of MA and ADX in regard to monoamines, it is suggested that some level of CORT was required in order to maintain monoamine levels at the same level as seen in the SHAM-MA group. Therefore, in this experiment, ADXA- or SHAM-operated animals were examined 24 h after four doses of MA on P11. For plasma CORT, there was a main effect of Surgery (F(1,17) = 34.60, p < 0.0001) and a Surgery × Treatment interaction (F(1,17) = 8.72, p < 0.009), but no Treatment effect (). CORT levels were significantly higher in the SHAM+MA group compared to both the SHAM+SAL and the ADXA+MA groups. The ADXA+SAL animals had lower CORT levels compared to the SHAM+SAL animals, and there was no difference between the ADXA+SAL animals and ADXA+MA animals.
Figure 8. CORT levels following ADXA or SHAM pretreatment and MA or SAL 24 h after the first drug dose on P11 (killed on P12). CORT levels were significantly higher in the SHAM+MA group compared to those in both the SHAM+SAL group and importantly the ADXA+MA group. The ADXA+SAL animals had lower CORT levels compared to the SHAM+SAL animals. Six animals/treatment were used. *p < 0.05.
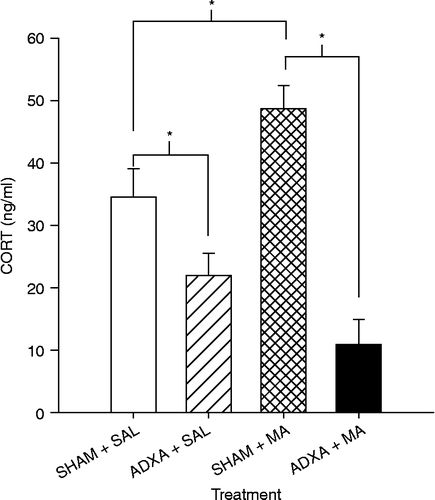
Hippocampal 5-HT was significantly affected by Treatment (F(1,11) = 5.44, p < 0.04; ). 5-HT was decreased in both MA-treated groups compared to the SAL-treated groups without regard to surgery. 5-HIAA in the hippocampus and 5-HT, 5-HIAA, and DA in the neostriatum were not affected by Surgery or Treatment or the interaction of Surgery and Treatment (, respectively). In the neostriatum, DOPAC was affected by Treatment (F(1,14) = 7.37, p < 0.02) such that the MA-treated groups had lower DOPAC levels than SAL-treated groups regardless of Surgery ().
Figure 9. Hippocampal and neostriatal monoamines following ADXA or SHAM pretreatment and MA or SAL 24 h after the first drug dose on P11 (killed on P12). 5-HT in the hippocampus (A) was significantly decreased in the MA-treated animals compared to that in SAL-treated animals regardless of surgery. 5-HIAA in the hippocampus (B), neostriatal 5-HT (C), neostriatal 5-HIAA (D), and neostriatal DA (E) were not affected by surgery or treatment nor were there any interactions. DOPAC (F) in the neostriatum was affected by treatment such that MA-treated animals had lower DOPAC levels than SAL-treated animals regardless of surgery. *p < 0.05.
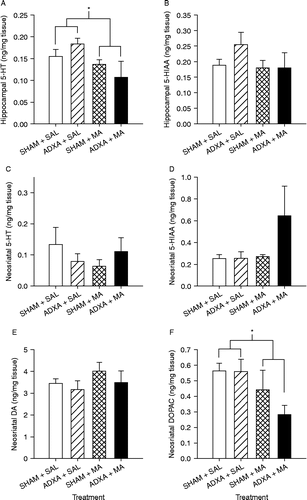
Discussion
The HPA axis response to stress is initiated by the release of corticotropin-releasing factor from the paraventricular nucleus of the hypothalamus, followed by pituitary release of ACTH, and ultimately CORT from the adrenals. CORT affects both glucocorticoid and mineralocorticoid receptors throughout the brain, including the hippocampus, and has been shown to modulate learning ability in adult animals (de Kloet et al. Citation1998; Oitzl et al. Citation2001; Roozendaal et al. Citation2006). In developing animals, large increases in CORT are detrimental to the developing nervous system, and our previous data show that MA can induce large increases in CORT relative to what is normal at these ages (Williams et al. Citation2000a, Citation2006; Schaefer et al. Citation2006, Citation2008). In this experiment, we first attempted to block the CORT increase with MET, a drug that blocks the production of CORT by preventing the enzymatic conversion of 11-β-deoxycorticosterone to CORT. In the adult rat, pretreatment with MET is successful at preventing the learning deficits induced by fenfluramine, a drug that increases CORT (Skelton et al. Citation2004). Although MET appeared to be effective at blocking MA-induced increases in CORT during the period of MA treatment on P11, a dramatic 407% paradoxical increase in CORT compared to control animals occurred 24 h later. A potential cause for this increase may be that a large increase in 11-β-deoxycorticosterone was available for conversion to CORT once MET levels began to decline. Therefore, we determined if we could block the production of CORT using KTZ, which reduces adrenal androgen biosynthesis of CORT and its precursors by inhibition of C17, 20-desmolase (Weber et al. Citation1993). Similar to MET, KTZ was unable to block the 24-h increase in CORT induced by MA because a 239% rebound increase was observed. We, therefore, removed the adrenal glands prior to MA administration to prevent CORT increases with the anticipation that maternal CORT obtained via lactation would be sufficient for normal CNS development in the pups. Although we showed that low levels of CORT were present in the ADX-MA-treated animals, we found that ADX exacerbated MA-induced brain monoamine reductions. Subsequently, ADXA was used to temporarily remove the blood supply and permanently remove the sympathetic inputs to the adrenal such that CORT release would be blunted, but gradually restored through autoengraftment. Similar to ADX, ADXA successfully blunted neonatal MA-induced CORT increases; however, contrary to ADX, ADXA did not exacerbate alterations of brain serotonergic levels 24 h after the first MA dose. Accordingly, we conclude that ADXA is a feasible method of attenuating MA-induced increases in plasma CORT during development, and may be applicable to other models of early stress in which the effects of CORT release are hypothesized to play a role in long-term effects.
Outside of obtaining a method to block MA-induced CORT increases for future investigation, these experiments also provide information regarding the use of various drug manipulations and VEHs in developing animals. For example, pharmacological manipulation of the developing HPA axis was unsuccessful at preventing increased CORT levels for an extended 24-h period, and this suggests that studies employing MET or KTZ should examine CORT levels at some later time points. In this study, we also showed that DMSO should not be used as a VEH for developing animals because it significantly increased CORT compared to weighed-only controls with a tendency to increase CORT above that of SAL-injected controls, whereas the SAL-injected controls did not differ from the weighed-only controls (cf. , inset). Interestingly, with DMSO alone or with MET, we observed similar increases in CORT levels, suggesting that DMSO was interfering with the ability of MET to block the initial increase in CORT produced by DMSO. We, therefore, examined the impact of using a VEH-E for MET, and found that this was better tolerated by the pups (i.e. it did not induce behavioral activation) and did not produce exaggerated CORT increases. These data show that the selection of VEH is especially important in developing animals considering the influence that DMSO had on CORT release compared to ethanol.
ADXA is a procedure that has been used in humans to treat various tumors and diseases including Cushing's disease (Srougi and Gittes Citation1978; Xu et al. Citation1992; O'Riordain et al. Citation1994; Okamoto et al. Citation1996). In adult animals, it has been helpful at determining how adrenal tissue regenerates (Okamoto et al. Citation1992b; Taniguchi et al. Citation2004). The results of this experiment show that this procedure enables neonatal adrenal glands to slowly engraft such that CORT responses can be attenuated and yet prevents significant additional alterations in brain monoamines. This is important since neonatal MA treatment on P11 decreases hippocampal 5-HT, and developmental 5-HT decreases have been associated with later learning and memory deficits (Mazer et al. Citation1997). CORT levels in the ADXA+MA group were significantly lower than those in the SHAM+MA animals, but not different from those in the ADXA+SAL controls () unlike the case of ADX+MA vs. SHAM+MA animals. Additionally, the ADX+SAL animals had higher 5-HT levels than the SHAM+SAL animals, and hippocampal 5-HIAA levels were also decreased in the ADX+MA animals compared to the SHAM+MA animals, suggesting further disruption of the 5-HT system (). The ADX method also altered the dopaminergic system. Neostriatal DA and DOPAC levels were decreased in the ADX+MA animals compared to those in the SHAM+MA animals (), whereas there was no difference in DA or DOPAC levels between the analogous ADXA groups ().
It has been shown in humans that MA produces increases in circulating cortisol levels, the main glucocorticoid in humans. Certain populations of women will continue to abuse MA throughout pregnancy including the later half of gestation, which is the time period we are modeling using neonatal rats (Smith et al. Citation2003; Garcia-Bournissen et al. Citation2007). Cortisol readily crosses the placenta and could explain the finding that children born to mothers that abused MA throughout pregnancy exhibit the psychological disruptions and learning deficits as previously mentioned. This method may be useful for testing how maternal stress negatively affects offspring cognition, behavior, and emotional development (reviewed by van den Bergh et al. Citation2005).
The success of the developmental ADXA procedure at blunting CORT release caused by MA treatment and the ability of the adrenal gland to regain function will allow for the study of the impact of CORT in neonatal stress-related insults and its effect on adult behavior. With this procedure, there is no need to supplement the animal's water with salt because the nursing dam supplies the animal with sufficient hormone until the adrenal engraftment occurs and the animal is self-sufficient.
Acknowledgements
Early portions of these data were presented at the 12th annual meeting of the International Behavioral Neuroscience Society meeting in San Juan, Puerto Rico, 2003, and more recent portions at the Society for Neuroscience meeting in San Diego, California, 2007. This study is supported by National Institutes of Health grants DA006733 (CVV), DA007427 (GAG), and training grant ES007051 (TLS, CEG).
Declaration of interest: The authors report no conflicts of interest. The authors alone are responsible for the content and writing of the paper.
References
- Aisa B, Tordera R, Lasheras B, Del RJ, Ramirez MJ. 2007. Cognitive impairment associated to HPA axis hyperactivity after maternal separation in rats. Psychoneuroendocrinology. 32:256–266.
- Bayer SL, Altman J, Russo RJ, Zhang X. 1993. Timetables of neurogenesis in the human brain based on experimentally determined patterns in the rat. Neurotoxicology. 14:83–144.
- Chang L, Smith LM, LoPresti C, Yonekura ML, Kuo J, Walot I, Ernst T. 2004. Smaller subcortical volumes and cognitive deficits in children with prenatal methamphetamine exposure. Psychiatry Res. 132:95–106.
- Chang L, Cloak C, Jiang CS, Farnham S, Tokeshi B, Buchthal S, Hedemark B, Smith LM, Ernst T. 2009. Altered neurometabolites and motor integration in children exposed to methamphetamine in utero. Neuroimage. 48:391–397.
- Clancy B, Kersh B, Hyde J, Darlington RB, Anand KJS, Finlay BL. 2007. Web-based method for translating neurodevelopment from laboratory species to humans. Neuroinformatics. 5:79–94.
- Cloak CC, Ernst T, Fujii L, Hedemark B, Chang L. 2009. Lower diffusion in white matter of children with prenatal methamphetamine exposure. Neurology. 72:2068–2075.
- de Kloet ER, Vreugdenhil E, Oitzl MS, Joels M. 1998. Brain corticosteroid receptor balance in health and disease. Endocr Rev. 19:269–301.
- Garcia-Bournissen F, Rokach B, Karaskov T, Koren G. 2007. Methamphetamine detection in maternal and neonatal hair: Implications for fetal safety. Arch Dis Child Fetal Neonatal Ed. 92:F351–F355.
- Gould E, Woolley CS, Cameron HA, Daniels DC, McEwen BS. 1991. Adrenal steroids regulate postnatal development of the rat dentate gyrus: II. Effects of glucocorticoids and mineralocorticoids on cell birth. J Comp Neurol. 313:486–493.
- Grace CE, Schaefer TL, Herring NR, Skelton MR, McCrea AE, Vorhees CV, Williams MT. 2008. (+)-Methamphetamine increases corticosterone in plasma and BDNF in brain more than forced swim or isolation in neonatal rats. Synapse. 62:110–121.
- Lauder JM. 1993. Neurotransmitters as growth regulatory signals: Role of receptors and second messengers. Trends Neurosci. 16:233–240.
- Lauder JM, Krebs H. 1978. Serotonin as a differentiation signal in early neurogenesis. Dev Neurosci. 1:15–30.
- Liu H, Kaur J, Dashtipour K, Kinyamu R, Ribak CE, Friedman LK. 2003. Suppression of hippocampal neurogenesis is associated with developmental stage, number of perinatal seizure episodes, and glucocorticosteroid level. Exp Neurol. 184:196–213.
- Mazer C, Muneyyirci J, Taheny K, Raio N, Borella A, Whitaker-Azmitia P. 1997. Serotonin depletion during synaptogenesis leads to decreased synaptic density and learning deficits in the adult rat: A possible model of neurodevelopmental disorders with cognitive deficits. Brain Res. 760:68–73.
- McNaughton BL, Barnes CA, Meltzer J, Sutherland RJ. 1989. Hippocampal granule cells are necessary for normal spatial learning but not for spatially-selective pyramidal cell discharge. Exp Brain Res. 76:485–496.
- O'Riordain DS, Farley DR, Young WFJr, Grant CS, van Heerden JA. 1994. Long-term outcome of bilateral adrenalectomy in patients with Cushing's syndrome. Surgery. 116:1088–1093.
- Oitzl MS, Reichardt HM, Joels M, de Kloet ER. 2001. Point mutation in the mouse glucocorticoid receptor preventing DNA binding impairs spatial memory. Proc Natl Acad Sci USA. 98:12790–12795.
- Okamoto T, Fujimoto Y, Obara T, Ito Y, Kodama T. Experimental study on adrenal autografts in rats to preserve normal adrenocortical function after bilateral adrenalectomy. Eur Surg Res. 1992a; 24:112–118.
- Okamoto T, Fujimoto Y, Obara T, Ito Y, Kodama T. Experimental study on adrenal autografts in rats to preserve normal adrenocortical function after bilateral adrenalectomy. Eur Surg Res. 1992b; 24:112–118.
- Okamoto T, Obara T, Ito Y, Yamashita T, Kanbe M, Iihara M, Hirose K, Yamazaki K. 1996. Bilateral adrenalectomy with autotransplantation of adrenocortical tissue or unilateral adrenalectomy: Treatment options for pheochromocytomas in multiple endocrine neoplasia type 2A. Endocr J. 43:169–175.
- Roozendaal B, Okuda S, Van der Zee EA, McGaugh JL. 2006. Glucocorticoid enhancement of memory requires arousal-induced noradrenergic activation in the basolateral amygdala. Proc Natl Acad Sci USA. 103:6741–6746.
- Schaefer TL, Ehrman LA, Gudelsky GA, Vorhees CV, Williams MT. 2006. Comparison of monoamine and corticosterone levels 24 h following (+)methamphetamine, (+/ − )3,4-methylenedioxymethamphetamine, cocaine, (+)fenfluramine or (+/ − )methylphenidate administration in the neonatal rat. J Neurochem. 98:1369–1378.
- Schaefer TL, Skelton MR, Herring NR, Gudelsky GA, Vorhees CV, Williams MT. 2008. Short- and long-term effects of (+)-methamphetamine and (+/ − )-3,4-methylenedioxymethamphetamine on monoamine and corticosterone levels in the neonatal rat following multiple days of treatment. J Neurochem. 104:1674–1685.
- Skelton MR, Blankenmeyer TL, Gudelsky GA, Brown-Strittholt CA, Vorhees CV, Williams MT. 2004. Metyrapone attenuates the sequential learning deficits but not monoamine depletions following d,l-fenfluramine administration to adult rats. Synapse. 54:214–222.
- Skelton MR, Williams MT, Schaefer TL, Vorhees CV. 2007. Neonatal (+)-methamphetamine increases brain derived neurotrophic factor, but not nerve growth factor, during treatment and results in long-term spatial learning deficits. Psychoneuroendocrinology. 32:734–745.
- Smagin GN, Goeders NE. 2004. Effects of acute and chronic ketoconazole administration on hypothalamo–pituitary–adrenal axis activity and brain corticotropin-releasing hormone. Psychoneuroendocrinology. 29:1223–1228.
- Smith L, Yonekura ML, Wallace T, Berman N, Kuo J, Berkowitz C. 2003. Effects of prenatal methamphetamine exposure on fetal growth and drug withdrawal symptoms in infants born at term. J Dev Behav Pediatr. 24:17–23.
- Smith LM, Lagasse LL, Derauf C, Grant P, Shah R, Arria A, Huestis M, Haning W, Strauss A, Grotta SD, Fallone M, Liu J, Lester BM. 2008. Prenatal methamphetamine use and neonatal neurobehavioral outcome. Neurotoxicol Teratol. 30:20–28.
- Squire LR, Zola SM. 1998. Episodic memory, semantic memory, and amnesia. Hippocampus. 8:205–211.
- Srougi M, Gittes RF. 1978. Adrenal autotransplantation. Urol Surv. 28:41–48.
- Struthers JM, Hansen RL. 1992. Visual recognition memory in drug-exposed infants. J Dev Behav Pediatr. 13:108–111.
- Taniguchi A, Tajima T, Nonomura K, Shinohara N, Mikami A, Koyanagi T. 2004. Expression of vascular endothelial growth factor and its receptors Flk-1 and Flt-1 during the regeneration of autotransplanted adrenal cortex in the adrenalectomized rat. J Urol. 171:2445–2449.
- van den Bergh BR, Mulder EJ, Mennes M, Glover V. 2005. Antenatal maternal anxiety and stress and the neurobehavioural development of the fetus and child: Links and possible mechanisms. A review. Neurosci Biobehav Rev. 29:237–258.
- Vazquez DM. 1998. Stress and the developing limbic–hypothalamic–pituitary–adrenal axis. Psychoneuroendocrinology. 23:663–700.
- Vorhees CV, Inman-Wood SL, Morford LL, Broening HW, Fukumura M, Moran MS. 2000. Adult learning deficits after neonatal exposure to d-methamphetamine: Selective effects on spatial navigation and memory. J Neurosci. 20:4732–4739.
- Vorhees CV, Herring NR, Schaefer TL, Grace CE, Skelton MR, Johnson HL, Williams MT. 2008. Effects of neonatal (+)-methamphetamine on path integration and spatial learning in rats: Effects of dose and rearing conditions. Int J Dev Neurosci. 26:599–610.
- Weber MM, Lang J, Abedinpour F, Zeilberger K, Adelmann B, Engelhardt D. 1993. Different inhibitory effect of etomidate and ketoconazole on the human adrenal steroid biosynthesis. Clin Investig. 71:933–938.
- Williams MT, Inman-Wood SL, Morford LL, McCrea AE, Ruttle AM, Moran MS, Rock SL, Vorhees CV. Preweaning treatment with methamphetamine induces increases in both corticosterone and ACTH in rats. Neurotoxicol Teratol. 2000a; 22:751–759.
- Williams MT, Inman-Wood SL, Morford LL, McCrea AE, Ruttle AM, Moran MS, Rock SL, Vorhees CV. Preweaning treatment with methamphetamine induces increases in both corticosterone and ACTH in rats. Neurotoxicol Teratol. 2000b; 22:751–759.
- Williams MT, Vorhees CV, Boon F, Saber AJ, Cain DP. 2002. Methamphetamine exposure from postnatal day 11 to 20 causes impairments in both behavioral strategies and spatial learning in adult rats. Brain Res. 958:312–321.
- Williams MT, Moran MS, Vorhees CV. Refining the critical period for methamphetamine-induced spatial deficits in the Morris water maze. Psychopharmacology (Berl). 2003a; 168:329–338.
- Williams MT, Morford LL, Wood SL, Wallace TL, Fukumura M, Broening HW, Vorhees CV. Developmental d-methamphetamine treatment selectively induces spatial navigation impairments in reference memory in the Morris water maze while sparing working memory. Synapse. 2003b; 48:138–148.
- Williams MT, Brown RW, Vorhees CV. 2004. Neonatal methamphetamine administration induces region-specific long-term neuronal morphological changes in the rat hippocampus, nucleus accumbens and parietal cortex. Eur J Neurosci. 19:3165–3170.
- Williams MT, Schaefer TL, Furay AR, Ehrman LA, Vorhees CV. 2006. Ontogeny of the adrenal response to (+)-methamphetamine in neonatal rats: The effect of prior drug exposure. Stress. 9:153–163.
- Woolley CS, Gould E, McEwen BS. 1990. Exposure to excess glucocorticoids alters dendritic morphology of adult hippocampal pyramidal neurons. Brain Res. 531:225–231.
- Xu YM, Chen ZD, Qiao Y, Jin NT. 1992. The value of adrenal autotransplantation with attached blood vessels for the treatment of Cushing's disease: A preliminary report. J Urol. 147:1209–1211.