Abstract
The effect of methylated N-(4-N,N-dimethylaminocinnamyl) chitosan (TM-CM-CS) was investigated on paracellular permeability and its toxicity towards Caco-2 cells. Fluorescein isothiocyanate dextran 4,400 (FD-4) was used as the model compound for paracellular transport. The factors, i.e. the degree of quaternization (DQ) and the extent of N-substitution (ES) of the derivatives, were studied for the effect on transepithelial electrical resistance (TEER) and permeability. The results revealed that at pH 7.4, TM-CM-CS appeared to increase cell permeability in a dose-dependent manner, and the effect was relatively reversible at lower doses of 0.05–0.5 mM. The difference of the DQ and the ES of TM-CM-CS slightly affected the decrease of TEER values and the FD-4 permeability. The cytotoxicity of TM-CM-CS was concentration-dependent and did not cause an acute cytotoxic effect as analyzed by the MTT assay. These studies demonstrated that this novel modified chitosan has the potential to be used as an intestinal absorption enhancer of therapeutic macromolecules.
Introduction
The oral administration of macromolecules is limited by the intestinal epithelial barrier, which results in greatly reduced bioavailability and unpredictable pharmacokinetics and pharmacodynamics (CitationCano-Cebrian et al., 2005). Hydrophilic macromolecules are not absorbed by enterocytes unless their transport is mediated by a carrier. The paracellular route appears to be the preferred pathway; however, this route is restricted by tight junctions (CitationLapierre, 2000). It is postulated that the transient and reversible opening of tight junctions may increase the permeation and intestinal absorption of therapeutic macromolecules (CitationRoss & Toth, 2005).
Chitosan (CS) [(1→4)-2-amino-2-deoxy-β-D-glucan], a cationic polysaccharide consisting of N-acetyl glucosamine (GlcNAc) and glucosamine (GlcN), obtained from the deacetylation of chitin, has received wide attention as a pharmaceutical excipient because of its unique properties such as biocompatibility, biodegradability, low-immunogenicity, and non-toxicity (CitationThanou et al., 2001). In addition to the application of CS in controlled release drug delivery systems, several studies have highlighted the potential use of CS as an absorption enhancing agent for the administration of hydrophilic drugs (CitationThanoo et al., 1992; CitationTanima et al., 2002). The ability of CS to promote drug absorption across different epithelia (intestinal, nasal, buccal, corneal) is currently well-documented (CitationArtursson et al., 1994; CitationIllum et al., 1994; CitationBorchard et al., 1996). Also, the absorption promoting effect of CS has been studied by many research groups, and this effect is due to a combination of mucoadhesion and the transient opening of tight junctions in the mucosal cell membrane (CitationSchipper et al., 1997; CitationPortero et al., 2002). In vitro studies using Caco-2 cell monolayers have shown that CS induces a transient opening of tight junctions, which increases membrane permeability, particularly to polar drugs such as peptides and proteins (CitationBorchard et al., 1996; CitationPortero et al., 2002).
CS is a weak base; therefore, in neutral and basic environments, CS molecules lose their charge and precipitate from solution. Under these conditions, CS will be ineffective as an absorption enhancer, which limits its use in the more basic environment of the large intestine and colon. Therefore, several CS derivatives have been synthesized in the last few years to obtain a modified carrier with altered physicochemical characteristics. Based on a literature search, it can be hypothesized that the transmucosal drug absorption enhancing properties of quaternized chitosans depend on molecular weight (MW), degree of quaternization (DQ), and other structural features (CitationColo et al., 2004; CitationSandri et al., 2004). Recently, our research group have successfully synthesized modified chitosans such as methylated N-(4-N,N-dimethylaminobenzyl) chitosan (TM-Bz-CS), methylated N-(4-N,N-dimethylaminocinnamyl) chitosan (TM-CM-CS), and methylated N-(4-pyridinylmethyl) chitosan (TM-Py-CS). These modified chitosans are water-soluble at physiological pH; therefore, they may be favored to use as carriers for oral drug delivery. TM-Bz-CS showed in vitro absorption enhancing properties (CitationKowapradit et al., 2008); therefore, in this study, TM-CM-CS was evaluated for in vitro absorption enhancement by assaying transepithelial electrical resistance (TEER) and the permeability of Caco-2 cells monolayers using FITC-dextran (FD-4) as a model drug for hydrophilic macromolecules. Variables that influence absorption enhancing properties and cytotoxicity such as the degree of quaternization (DQ), the extent of dimethylaminocinnamyl substitution (ES) and the pH of culture medium were investigated.
Materials
Chitosan, with a weight-average molecular weight (Mw) of 276 kDa, was purchased from Seafresh Chitosan (lab) Co., Ltd (Thailand). The degree of deacetylation (DD) of this material was 94%. Dimethylaminocinnamaldehyde was purchased from Fluka (Deisenhofen, Germany). Sodium cyanoborohydride, iodomethane and 1-methyl-2-pyrrolidone were purchased from Acros Organics (Geel, Belgium). Sodium iodide was purchased from Carlo Erba Reagent (Italy), and all other reagents were distilled before the synthesis process. 3-(4,5-dimethylthiazol-2-yl)-2,5-diphenyl tetrazolium bromide (MTT) and fluorescein isothiocyanate dextran 4,400 (FD-4) were purchased from Sigma-Chemical Co. (St. Louis, MO). Dulbecco’s modified Eagle’s medium (DMEM), trypsin-EDTA, penicillin-streptomycin antibiotics, and fetal bovine serum (FBS) were obtained from GIBCO-Invitrogen (Grand Island, NY). The Caco-2 cell line was obtained from the American Type Culture Collection (Rockville, MD). Transwell (12-well plates) cell culture chambers inserted with a 3.0 µm pore size were purchased from Corning Life Sciences (MA). All other chemicals were of cell culture and molecular biology quality.
Methods
Synthesis of methylated N-(4-N,N-dimethylaminocinnamyl) chitosan (TM-CM-CS)
Synthesis of N-(4-N,N-dimethylaminocinnamyl) chitosan (3)
Synthesis of 3 was carried out in accordance with a previously reported procedure, as shown in (CitationSajomsang et al., 2008a). CS (2.0 g, 12.22 mmol) was dissolved in 1% v/v HOAc (150 mL). The solution was diluted in EtOH (100 mL). Next, 4-dimethylaminocinnamaldehyde (1.0 meq/GlcN) was added to the solution, and it was stirred at room temperature for 12 h; however, 2.0 meq/GlcN (4.3 g) of 4-dimethylaminocinnamaldehyde was stirred at 60°C for 12 h. The pH of the solution was adjusted to 5 with 15% w/v NaOH. Subsequently, NaCNBH3 (3.08 g, 48.92 mmol) was added, and the solution was stirred at room temperature for 24 h, followed by the adjustment of the pH to 7. The reaction mixture was then dialyzed against distilled water and freeze-dried to produce a powder (3a 2.95 g, 77.6% yield).
ATR-FTIR; ν 3384 (O-H and N-H, GlcN), 2912, 2847, and 2798 (C-H, GlcN), 1611 and 1518 (C=C, Aromatic), 1131 (C-O-C, GlcN), 1056 and 1021 cm−1 (C-O, GlcN), 801 cm−1 (C-H, Aromatic). 1H-NMR (CD3COOD): δ (ppm) 7.5 (m, 4H, Ph), 4.8 (s, 1H, H1), 4.5 (d, 2H, CH2-NH), 4.5–3.0 (m, 6H, H2-H6) 3.1 (s, 6H N(CH3)2), 3.0 (s, 1H H2), 1.9 (s, 3H, NHAc).
Synthesis of methylated N-(4-N,N-dimethylaminocinnamyl) chitosan (4)
Previously, the methylation of chitosan containing aromatic moieties was carried out using iodomethane in the presence of N-methyl pyrrolidone (NMP) and sodium hydroxide (CitationSajomsang et al., 2008b). Therefore, the same procedure was used to synthesize 4, as shown in . A mixture of 3a (1.0 g) and N-methyl pyrrolidone (NMP, 50 mL) was stirred at room temperature for 12 h. Next, 15% (w/v) of aq NaOH (8.0 mL) and sodium iodide (3.0 g) were added and stirred at 60°C for 15 min. Subsequently, iodomethane (8.0 mL) was added in three portions at 3 h intervals and stirred at 60°C for 24 h. The obtained compound was precipitated in acetone (600 mL). The precipitate was dissolved in 15% w/v NaCl solution to replace the iodide ions with chloride ions. The suspension was dialyzed with deionized water for 3 days to remove inorganic materials and then freeze-dried to produce a cotton-like power (4a).
ATR-FTIR; ν 3357, (O-H and N-H, GlcN), 1658, 1510 (C=C, Aromatic), 1465 (C-H, N+(CH3)3), 1107 (C-O-C, GlcN), 1046 and 1013 cm−1 (C-O, GlcN), 847 cm−1 (C-H, Aromatic). 1H-NMR (D2O): δ (ppm) 7.9–7.3 (m, 4H, Ph), 6.7–6.3 (dd, 2H CH=CH), 5.2 (br. s, 1H H1′), 4.6–3.0 (33H, m, 7H CH2-NH and H2-H6; s, 9H N+(CH3)3Ph; s, 6H 3, 6-O-CH3; s, 9H N+(CH3)3), 2.7 (s, 6H N(CH3)2), 1.9 (s, 3H, NHAc).
Characterization
All Fourier Transform Infrared spectrophotometer (FT-IR) spectra were collected with a Nicolet 6700 spectrometer (Thermo company, USA) using single bounce ATR-FTIR spectroscopy (Smart Orbit accessory) with a diamond internal reflection element (IRE) at ambient temperature. The 1H-NMR spectra were measured on the ADVANCE AV 500 spectrometer (Bruker, Switzerland). All measurements were performed at 300 K, using pulse accumulation of 64 scans and an LB parameter of 0.30 Hz. D2O/CF3COOD and D2O were used as the solvents for chitosan and its derivatives, respectively. The weight average molecular weight (Mw), the number average molecular weight (Mn) and the Mw/Mn of chitosan and its derivatives were determined using gel permeation chromatography (GPC) consisting of the Waters 600E Series generic pump and injector, the Ultrahydrogel linear Columns (Mw resolving range 1–20,000 kDa) and the guard column. Pollulan was used as the standard (Mw 5.9–788 kDa) refractive index detector (RI). All samples were dissolved in acetate buffer (pH 4) and then filtered through 0.45 μm syringe filters (VERTIC Vertical chromatography Co., Ltd, Thailand). The mobile phase was 0.5 M AcOH and 0.5 M AcONa (acetate buffer pH 4) at a flow rate of 0.6 mL/min at 30°C, and a 20 μL injection volume was used.
Cell cultures
Caco-2 cells were maintained in Dulbecco’s modified Eagle’s medium (DMEM) at a pH of 7.4, supplemented with 10% fetal bovine serum, 2 mM L-glutamine, 1% non-essential amino acid solution, and 0.1% penicillin-streptomycin solution in a humidified atmosphere (5% CO2, 95% air, 37°C). The cells were grown under standard conditions until 60–70% confluency. Cells from passages 20–40 were used for all of the experiments. The cells were seeded on tissue culture polycarbonate membrane filters (pore size 3.0 µm) in 12-well Transwell® plates (Costar®, corning Inc., Corning, NY) at a seeding density of 2 × 104 cells/cm2. The culture medium was added to both the donor and the acceptor compartment. Medium was changed every second day. The cells were left to differentiate for 15–21 days after seeding with monitoring of trans-epithelial electrical resistance (TEER) values were more than 600 Ω.cm2 using a Millicell® ERS meter (Millipore, Bedford, MA).
Measurement of the trans-epithelial electrical resistance (TEER)
Measurement of TEER was performed to evaluate possible damage of the cellular monolayer during the experiments. The values of TEER were determined by measuring the potential difference between the two sides of the cell monolayer using a Millicell® ERS meter connected to a pair of chopstick electrodes. On the day of experiments, the cells were washed twice with phosphate buffered saline (PBS) and pre-equilibrated for 1 h with Hank balanced salt solution (HBSS) buffered at pH 7.4. After removing the medium, the Caco-2 cell monolayers were treated with CS or CS derivative solutions (0.05–5 mM in HBSS at pH 7.4) in the apical compartment. Chitosan acetate (CSA) solution was prepared by dissolving chitosan base in 1% solution of acetic acid with gentle stirring for 12 h. The pH of CSA solutions was adjusted to pH 6.2 and 7.4 by adding 0.1 N NaOH. CS derivative solutions were prepared by dissolving CS derivative in HBSS at pH 7.4 with gentle stirring. The TEER was measured every 20 min. After 2 h of treatment, the cells were carefully washed twice with PBS and incubated with fresh culture medium. The recovery of TEER values was monitored for 24 h after the treatment.
Transport studies
The transport of FD-4 across the Caco-2 cell monolayers at pH 7.4 was studied. Caco-2 monolayers grown in Transwell® (12-well) plates were used for the transport studies when they had differentiated and the monolayer was intact, as checked by measuring the TEER. Prior to the experiment, the cells were washed twice with PBS and pre-equilibrated for 1 h with HBSS buffered at pH 7.4. After removing the medium, the cells were treated with TM-CM-CS solutions (0.5 mM in HBSS pH 7.4) in the apical compartment for 2 h. In the control wells, the same media without CS derivatives were used. In all cases, HBSS pH 7.4 was used as the basolateral medium. After 2 h of treatment, the cells were carefully washed twice with PBS, and FD-4 solution (1 mg/mL) was added on the apical side of the monolayers. Samples (1 mL) were taken under sink conditions at 30, 60, 90, 120, 180, and 240 min from the basolateral side and replaced with an equal volume of fresh HBSS solution. The amount of FD-4 was determined using a fluorescence 96-well plate reader (Universal Microplate Analyzer, Model AOPUS01 and AI53601, Packard BioScience, CT). The excitation and emission wavelengths were 400 and 535 nm, respectively. Results were expressed as cumulative transport as a function of time. All experiments were done in triplicate at 37°C. The apparent permeability coefficient was calculated according to equation (1):
where Papp is the apparent permeability coefficient (cm/s), dQ/dt (μg/s) is the rate of appearance of FD-4 on the basolateral side, A is the surface area of the monolayers, and C0 (μg/mL) is the initial drug concentration in the donor compartment. All rate constants were obtained from the permeation profiles of each compound. Absorption enhancement ratios (R) were calculated from Papp values by using equation (2) (CitationKotze et al., 1998):
Evaluation of cytotoxicity
The cytotoxic effects of CS derivatives were investigated with Caco-2 cells using the MTT cytotoxicity assay. Cells were seeded at a density of 2 × 104 cells/well in 96-well cell culture plates. After pre-incubation for 24 h, cells were then treated with CS derivatives at various concentrations ranging from 0.05–5 mM in serum-free medium (pH 7.4) and incubated for 24 h. Dilutions of CS derivatives were made using serum-free medium to ensure that the cells did not die from nutrition deficiency. After treatment, CS derivative solutions were removed, fresh medium was added, and the cells were incubated for 4 h. Finally, the cells were incubated with 100 μl MTT-containing medium (0.1 mg/mL MTT in serum-free medium) for 4 h. Next, the medium was removed, and the formazan crystal that formed in the living cells was dissolved in 100 μl DMSO per well. The relative viability (%) was calculated based on absorbance at 550 nm using a microplate reader (Universal Microplate Analyzer, Model AOPUS01 and AI53601, Packard BioScience, CT). The viability of non-treated control cells was arbitrarily defined as 100% (CitationChae et al., 2005). The relative cell viability was calculated according to equation (3), and the IC50 was calculated as a chitosan concentration that inhibited the growth of 50% of the cells relative to non-treated control cells.
Statistical analysis
All experimental measurements were collected in triplicate. Values are expressed as mean ± standard deviation (SD). Statistical significance of differences in permeability enhancer and cell viability were examined using one-way analysis of variance (ANOVA) followed by a LSD post-hoc test. The significance level was set at p < 0.05.
Results
Synthesis of methylated N-(4-N,N-dimethylaminocinnamyl) chitosan (TM-CM-CS)
N-(4-N,N-dimethylaminocinnamyl) chitosan (3) was created in the reaction between primary amino groups of CS (1) and 4-dimethylaminocinnamaldehyde to yield the corresponding Schiff base (2) intermediates and was then converted to 3 upon hydrogenation with sodium cyanoborohydride (). This process is a versatile and specific method that creates a covalent bond between a substrate and the amine function of the CS, as reported by many research groups (CitationCrini et al., 1997; CitationSashiwa & Shigemasa, 1999; CitationRabea et al., 2005; CitationSajomsang et al., 2008a). The extent of N-substitution (ES) was determined by 1H-NMR spectroscopy (CitationCrini et al., 1997). The different mole ratios of aromatic aldehyde to primary amino groups of CS were used to determine the extent of N-substitution. Comparison of the integral area of the H2 + 1/3NHAc signals with the area of the aromatic protons allows the ES of the N-aryl group to be calculated using equation (4).
where ES (%) is the extent of N-substitution, Ar is the integral area of aromatic protons, n is number of aromatic hydrogen atoms per substituent, H2 is the integral area of the protons at C-2 carbon of GlcN, and NHAc is the integral area of GlcNAc protons.
It was determined that the ES and the yield of 3 were in the range of 50 ± 2 to 76 ± 2 and 70–77%, respectively. The 3 was subjected to methylation with iodomethane in the presence of NMP and sodium hydroxide, which yielded 4 (). The methylation was based on a nucleophilic substitution of the primary amino group on the C-2 position of CS, and iodomethane and sodium iodide were used as catalysts. It has been reported that the chloride counter-ion enhances the storable stability of the quaternary ammonium salts of CS compared to the iodide counter-ion (CitationDomard et al., 1986). In this study, the iodide counter-ion was exchanged with the chloride counter-ion by dissolving 3 (iodide formed) in an aqueous solution of 15% w/v sodium chloride and then dialyzed with deionized water. The DQ was generally determined using equation (5) (CitationSieval et al., 1998).
In the experimental results, DQ at the primary amino groups of CS is denoted as DQCS, N+(CH3)3 is the integral area of the N,N,N-trimethyl protons at δ = 3.2 ppm, and H1′ is the integral area of both H1′ and H1 protons in the range of δ = 5.2–4.7 ppm.
It is important to note that the total DQ increased when the ES was increased by a single methylation. In comparison to the single methylation of CS, a DQ of TM-CS that was ≤ 30% was obtained and was lower than the DQ of 4 under the same conditions (data not shown). However, in this study, TM-CS was repeatedly methylated twice. N-methylation led to the quaternization of the N,N-dimethylaminocinnamyl moiety and the primary amino groups of GlcN of CS. This phenomenon was confirmed by 1H-NMR spectra as shown in . The proton signal at δ = 3.1 ppm of N,N-dimethylaminocinnamyl protons was changed to N,N,N-trimethylaminocinnamyl protons at δ = 3.5 ppm. In addition to quaternization, N,N-dimethylation and N-methylation at the primary amino groups of CS were also observed. The results also revealed that 3-O-methylation and 6-O-methylation occurred during the methylation process ().
Table 1. Methylation of chitosan and N-(4-N,N-dimethylaminocinnamyl) chitosan (mean ± SD, n = 3).
Figure 1. 1H NMR spectra of (1) chitosan, (3a) N-(4-N,N-dimethylaminocinnamyl) chitosan, and (4a) methylated N-(4-N,N-dimethylaminocinnamyl) chitosan with ES 50%.
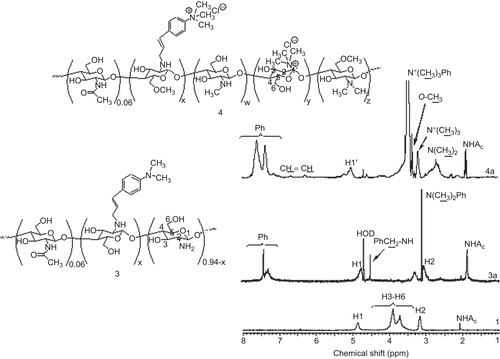
The chemical structures of 3 and 4 were characterized by ATR-FTIR and 1H-NMR spectroscopy. In this study, the solid samples of CS and its derivatives were characterized by using single bounce ATR-FTIR spectroscopy (Smart Orbit accessory) with a diamond internal reflection element (IRE). depicts the characteristic ATR-FTIR pattern of CS (1). The absorption bands at wave numbers 3430 cm−1 are due to OH and NH2 groups. The bands at 1648 and 1377 cm−1 correspond to the stretching of C=O and C–O in the amide group. The band at 1594 cm−1 is due to the N-H deformation of amino groups, and the bands at 1155, 1081, and 1033 cm−1 correspond to the symmetric stretching of the C-O-C and involves the skeletal vibration of the C-O stretching (CitationBrugnerotto et al., 2001). The ATR-FTIR spectrum of 3 was similar to the spectrum of 1 except that additional absorption bands at wave numbers 1611, 1518, and 801 cm−1 were observed. These bands were assigned to the C=C stretching and C–H deformation (out of plane) of the aromatic group. The 4 exhibited the characteristic ATR-FTIR spectrum at wave number 1473 cm−1 which was similar to the ATR-FTIR spectrum of TM-CS and is due to C–H symmetric bending of the methyl substituent of the quaternary ammonium groups (CitationSajomsang et al., 2008b). It was confirmed that methylation successfully occurred at the N,N-dimethylaminocinnamyl group in the CS backbone.
Figure 2. FTIR spectra of (1) chitosan, (3b) N-(4-N,N-dimethylaminocinnamyl) chitosan, (4b) methylated N-(4-N,N-dimethylaminocinnamyl) chitosan with ES 76% and TM-CS.
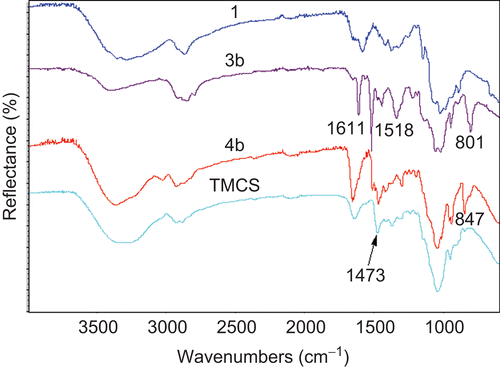
All the 1H-NMR spectra exhibited the characteristic 1H-NMR pattern of 1, i.e. the multiplet at δ = 4.0–3.0 ppm due to H3, H4, H5, H6, and two singlets at δ = 3.1 and 2.1 ppm due to the H2 proton of the GlcN and N-acetyl protons of GlcNAc, respectively (CitationSnyman et al., 2002). The 1H-NMR spectrum of 3 exhibited the broad multiplet protons signals at δ = 7.4 ppm and a singlet proton signal at δ = 3.1 ppm, which were assigned to the aromatic protons and N,N-dimethyl protons at the para-position of the aromatic group, respectively. The 1H-NMR spectra of 4 showed the proton signal at δ = 5.2 ppm, which is unambiguously assigned to the H1′ proton of the GlcN of 4. The proton signals at δ = 3.5, 3.2, 2.7, and 2.3 were assigned to the N,N,N-trimethyl protons of the benzyl substituent, the N,N,N-trimethyl protons, the N,N-dimethyl protons, and the N-methyl protons of GlcN, respectively. Furthermore, O-methylation was also observed at δ = 3.3 and 3.4 ppm and was assigned to 6-O-methylated and 3-O-methylated protons. In this study, the DQ of 4 was determined by the 1H-NMR method in accordance with previous reports by CitationSieval et al. (1998). The results showed that the total DQ of 4a and 4b were 65 ± 2 and 82 ± 2%, respectively.
All weight average molecular weight (Mw), number average molecular weight (Mn) and Mw/Mn of CS and its derivatives were determined by gel permeation chromatography (GPC). Every sample was repeatedly measured three times. The molecular weight average of the parent CS was determined to be Mn = 48.71 kDa, Mw = 276.06 kDa and Mw/Mn = 5.67. A relatively wide molecular weight distribution with a polydispersity index (PDI) of CS was observed. Moreover, the methylation reaction led to a significant reduction in the CS molecular weight that was observed in compound 4. CitationSnyman et al. (2002) found that a decrease in molecular weight of CS depended on the methylation procedure, and the molecular weight of CS much more decreased with the extension of the reaction duration, which correlated to an increase in DQ. Furthermore, a similar result was observed by CitationSajomsang et al. (2008b). They determined that the molecular weight of CS slightly decreased during the N-arylation step. The methylation step leading to methylated CS derivatives was accompanied by a significant molecular weight decrease. An oxidative degradation process and alkaline depolymerization occurred. In addition, the molecular weight of CS decreased with an increase in the number of reaction steps or additional steps (CitationJintapattanakit et al., 2008).
The effect of TM-CM-CS on TEER
The effect of DQ, ES, and the concentrations of TM-CM-CS on the TEER of Caco-2 cell monolayers is summarized in and . The incubation of the monolayers on the apical side with 0.05–5 mM polymers at pH of 7.4 for 2 h resulted in an immediate reduction in TEER values compared to the control group (). All CS derivatives affected the TEER values in a concentration-dependent manner as shown by the significant decrease in TEER values when the concentration of polymers increased. The chitosan with additional cinnamyl groups had a greater effect on decreasing TEER values than the chitosan that possessed only trimethyl functionality, as shown by the comparison at the same % DQ (TM64CS). However, increasing both the DQ and the ES of TM-CM-CS did not significantly affect the decrease of TEER values. All CS derivatives dissolved at a neutral pH of 7.4 and reduced TEER values, except for CSA, which did not dissolve at a neutral pH and reduced TEER values only at an acidic pH of 6.2 (). The reduction in TEER after 2 h of incubation with 0.5 mM of CS and its derivative occurred in the following order: TM65CM50CS (76.44% reduction) > TM82CM76CS (74.78% reduction) > CSA at pH 6.2 (67.82% reduction) > TM64CS (52.89% reduction) ().
Figure 3. The effect of TM-CM-CS on the TEER of Caco-2 cell monolayers: (a) TM65CM50CS, (b) TM82CM76CS. The TEER recovery pattern of the Caco-2 cell monolayers: (c) TM65CM50CS, (d) TM82CM76CS concentrations ranging from 0.05–5 mM, at pH 7.4 (♦) control, (▪) 0.05 mM, (▴) 0.5 mM, (◊) 1.25 mM, (□) 2.5 mM, (Δ) 5 mM. Each point represents the mean of three experiments. * p < 0.05.
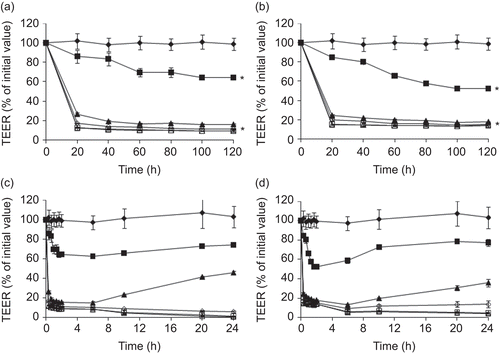
Table 2. The effect of the degree of quaternization (DQ), the extent of N-substitution (ES) of TM-CM-CS, the polymer concentration and the pH on TEER.
Figure 4. The percentage of the reduction in TEER after 2 h incubation with 0.5 mM chitosan acetate (CSA) at pH 6.2 and 7.4 and chitosan derivatives at pH 7.4. Each point represents the mean of three experiments. * p < 0.05.
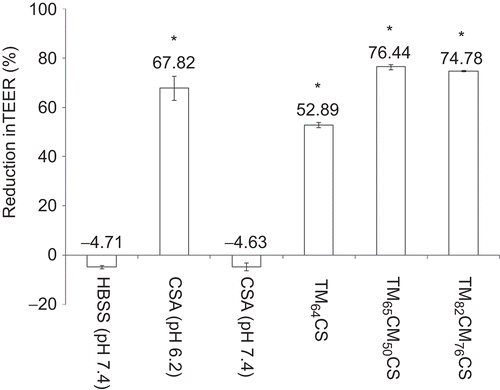
After the polymer solutions were removed, the cells were repeatedly washed and subsequently supplied with fresh medium and an increase in resistance towards the initial values was found in the control and in cells treated with 0.05–5 mM CS derivatives by 24 h ( and ). Nevertheless, the TEER recovery was obvious within 24 h if the cells were treated with CS derivatives at low concentrations of 0.05–0.5 mM, suggesting that the effects of TM-CM-CS were reversible at the low concentrations used. For the subsequent transport study, the concentration of 0.5 mM was chosen because it was the safest and most effective dose.
The effect of TM-CM-CS on the transport of FD-4
To further evaluate the direct ability of TM-CM-CS to increase the permeability of hydrophilic macromolecules, we studied the effect of the DQ and the ES of these derivatives on the transport of hydrophilic macromolecules such as FD-4 across Caco-2 cell monolayers. FD-4 is a negatively charged polymer; therefore, its aggregation resulted from strong electrostatic interactions with the positively charged TM-CM-CS. In the present studies, Caco-2 cells were pre-incubated with TM-CM-CS for 2 h before the addition of FD-4 in the acceptor compartments, and the accumulation of FD-4 in the basolateral compartments was measured. shows the transport of FD-4 across the Caco-2 cell monolayers in the presence of TM-CM-CS with different DQ and ES values at the concentration of 0.5 mM and in the absence of TM-CM-CS as a control. The cumulative transport of FD-4, Papp values and absorption enhancement ratios (R) were calculated and are shown in . Under the conditions described, very low baseline permeability was determined. Incubation with TM-CM-CS resulted in an accumulation of FD-4 in the acceptor compartment. A difference in the transport of FD-4 was found at different DQ and ES. The cumulative amounts transported up to 4 h after incubation with FD-4 are in the following order: TM65CM50CS (12.71 ± 1.6 µg) > TM82CM76CS (8.11 ± 2.2 µg) > TM64CS (3.40 ± 1.2 µg).
Table 3. The effect of different degrees of quaternization (DQ) and the extent of N-substitution (ES) of TM-CM-CS on the transport of FD-4.
Cytotoxicity of TM-CM-CS
Cationic polymers have been known to be cytotoxic materials, which is most likely due to their positive charges. Therefore, in this study, the effect of DQ and ES of TM-CM-CS on cytotoxicity was determined. The results showed that CSA at pH 7.4 incubated with Caco-2 cells for 24 h did not remarkably change the viability of Caco-2 cells, whereas at pH 6.2, it showed concentration-dependent cytotoxicity with an IC50 of 0.9 ± 0.01 mM (). Both TM-CM-CSs tested showed concentration-dependent cytotoxicity in Caco-2 at pH 7.4 incubated for 24 h. IC50 value of TM65CM50CS and TM82CM76CS was 0.14 ± 0.01 and 0.12 ± 0.01 mM, respectively.
Table 4. Cytotoxicity TM-CM-CS incubated with Caco-2 cells for 2 and 24 h (n = 8).
Discussion
It is well known that CS solutions cause a significant and dose-dependent decrease of TEER of the Caco-2 cell monolayers. The first evidence demonstrating this phenomenon was reported in the early 1990s. It has been proposed that CS acts on negatively charged sites at the cell surfaces and tight junctions, and it has been shown that CS is able to induce changes in F-actin distribution (CitationArtursson et al., 1994). Although F-actin is directly or indirectly associated with proteins in the tight junctions (CitationMadara, 1987), CS most likely promotes the paracellular transport of hydrophilic compounds by an indirect mechanism, whereby the integrity of the tight junctions is altered by changes in intracellular F-actin. Changes in paracellular barrier properties occur as a cellular action mediated by polycations rather than as a consequence of direct action on the junctional complex (CitationMcEwan et al., 1993). This hypothesis is supported by a recent study that concluded that the interaction of CS with the cell membrane results in a structural reorganization of tight junction-associated proteins, followed by enhanced transport through the paracellular pathway. Therefore, binding of CS to the Caco-2 cells precedes absorption enhancement, and this increase in absorption is mediated by the positive charges on the polymer (CitationSchipper et al., 1997). However, CS demonstrates its absorption enhancer activity only in acidic conditions due to solubility problems. Many attempts have thus been made in the synthesis of CS derivatives to overcome this solubility problem.
To increase the solubility at neutral physiological pH, the quaternary ammonium moiety in CS is required. The above-mentioned properties of cationic polymers have been known to be cytotoxic materials (CitationKotze et al., 1998). Due to the amphiphilic nature of the cell membrane, an increase in the interaction between the cell membrane and the CS derivative may be favored and more safe when the macromolecule contains hydrophobic residues. As a result, CS derivatives containing quaternary ammonium functionality in addition to different hydrophobic substitutions were excellent candidates for novel absorption enhancers. Recently, our research group successfully synthesized modified chitosans and determined that methylated N-(4-N,N-dimethylaminobenzyl) chitosan (TM-Bz-CS) showed in vitro absorption enhancing properties. Furthermore, this chitosan showed decreased cytotoxicity to Caco-2 cells when the %ES of the benzyl groups on the C-2 position of the backbone of the CS was increased (CitationKowapradit et al., 2008). To investigate different hydrophobic substitutions, TM-CM-CS was evaluated for its in vitro absorption enhancement on the transepithelial electrical resistance (TEER) and permeability of Caco-2 cell monolayers using FD-4 as the model drug of hydrophilic macromolecules. TM-CM-CS was synthesized by the covalent bond formation between the primary amino groups of CS and the dimethylaminocinnamyl group to provide a hydrophobic moiety, which improves the hydrophobic interaction with the cell membrane. The methylation of the CS molecule containing hydrophobic moieties was carried out using iodomethane to render CS soluble. The results showed that increasing concentrations of TM-CM-CS (0.05–0.5 mM) resulted in a dose-dependent effect on tight junction permeability at pH 7.4. Increasing the concentration of the polymers from 0.5 mM to 5 mM did not result in significant decreases in TEER values compared with the reduction measured with 0.05–0.5 mM. A similar trend in the decrease of TEER as a function of low CS concentration was previously reported (CitationKotze et al., 1998; CitationKowapradit et al., 2008). The effect on TEER values appears to be saturable because no significant differences were found when higher concentrations of polymers (0.5–5 mM) were used. This phenomenon may be due to the ability of Caco-2 cells to recover from the treatment of polymers. When the cells were treated with TM-CM-CS (0.05–0.5 mM), the recovery of TEER could be gradually observed by 24 h, whereas it was not observed when the cells were treated with 1.25–5 mM of TM-CM-CS. This finding is consistent with previous reports (CitationKotze et al., 1998) in which the complete removal of the polymers, without damaging the cells, proved to be difficult due to the high viscosity of the solution. This finding may be the reason why the increase in resistance was only gradual and did not reach 100%. Our results showed that higher concentrations of TM-CM-CS resulted in higher cytotoxicity. This finding is in agreement with the observations of a previous study that showed that CS had a concentration-dependent cytotoxic effect on Caco-2 cells (CitationChae et al., 2005). Therefore, this high concentration (1.25–5 mM) of TM-CM-CS may damage the cells, which resulted in the lack of TEER recovery. The results in demonstrate that CS containing cinnamyl functionality affected the decrease of TEER values and FD-4 transport (). These results indicated that the introduction of the trimethylaminocinnamyl group into the CS polymer backbone enhanced the hydrophobicity, which improved the hydrophobic interaction between the polymer and the cell membrane. These improvements helped the water-soluble CS to be an efficient absorption enhancer. However, we did not observe a decrease in the TEER values when the degree of DQ and ES of TM-CM-CS was increased. As reported previously, hydrophobic modified cationic polymers with optimal substitutions may act as absorption enhancers by increasing cell membrane/polymer interactions or via the destabilization of the cell membranes. Our previous results on TM-Bz-CS showed an optimal degree of DQ and ES at TM56Bz42CS (CitationKowapradit et al., 2008). In this study, TM65CM50CS had the optimal degree of DQ and ES for TM-CM-CS. This finding may be due to the cytotoxicity of the high degree of DQ and ES. According to IC50 at 24 h of these modified CS molecules, TM82CM76CS appeared to be the most toxic. Therefore, an increase in the degree of DQ and ES did not enhance the TEER value and FD-4 transport. The structure of the hydrophobic substitutions of TM-Bz-CS and TM-CM-CS with the same %DQ and %ES were compared, and the recovered TEER values of TM65CM50CS and TM56Bz42CS were 0.5 and 1.25 mM, respectively. The IC50 of these modified CS molecules indicated that TM65CM50CS (IC50 was 0.14 ± 0.01) had a more cytotoxic effect on Caco-2 cells than TM56Bz42CS (IC50 was 1.70 ± 0.01) (CitationKowapradit et al., 2008). These findings suggest that the addition of the aliphatic hydrocarbon (alkyl group) to the structure of the hydrophobic substitution may induce a toxic effect on the cells. Further studies are in progress to verify these hypotheses and to clarify the mechanisms responsible for these findings. Limitations of in vitro Caco-2 cell monolayer tests exist; such as the absence of villi and a mucus layer have to be noted. Additionally, Caco-2 cells have tighter junctions in comparison with human or animal small intestine cells, which are explained by the colonic origin of these cells (CitationBalimane & Chong, 2005). Therefore, the in vivo permeability of these CS derivatives require further investigation. However, the obtained results suggest that an optimal degree of DQ and ES of TM65CM50CS may be a potential candidate for an oral absorption enhancer.
Conclusion
TM-CM-CS is a novel polymeric permeation enhancer. TM65CM50CS proved to be the best compound that was superior in water-solubility, caused a reversible decrease in the TEER of Caco-2 cell monolayers, and increased the paracellular permeability of hydrophilic markers. This modified chitosan has the potential to be used as intestinal absorption enhancers of therapeutic macromolecules.
Declaration of interest
The authors wish to thank The Commission of Higher Education (Tailand), The Thailand Research Funds through the Golden Jubilee Ph.D. Program (Grant No. PHD/0114/2550), the National Research Council of Thailand, and the Silpakorn University Research and Development Institute for financial support.
References
- Artursson, P., Lindmark, T., Davis, S.S., Illum, L. (1994). Effect of chitosan on the permeability of monolayers of intestinal epithelial cells (Caco-2). Pharm Res. 11:1358–61.
- Balimane, P.V., Chong, S. (2005). Cell culture-based models for intestinal permeability: a critique. Drug Discov Today. 10:335–43.
- Borchard, G., Lueszen, H.L., Boer, D.A.G., Verhoef, J.C., Lehr, C.M., Junginger, H.E. (1996). The potential of mucoadhesive polymers in enhancing intestinal peptide drug absorption. III. Effects of chitosan-glutamate and carbomer on epithelial tight junctions in vitro. J Contr Rel. 39:131–8.
- Brugnerotto, J., Lizardi, J., Goycoolea, F.M., Argüelles-Monal, W., Desbrières, J., Rinaudo, M. (2001). An infrared investigation in relation with chitin and chitosan characterization. Polymer. 42:3569–80.
- Cano-Cebrian, M.J., Zornoza, T., Granero, L., Polache, A. (2005). Intestinal absorption enhancement via the paracellular route by fatty acids, chitosans and others: a target for drug delivery. Curr Drug Deliv. 2:9–22.
- Chae, Y., Jang, M.K., Nah, J.W. (2005). Influence of molecular weight on oral absorption of water soluble chitosans. J Contr Rel. 102:383–94.
- Colo, D.G., Burgalassi, S., Zambito, Y., Monti, D., Chetoni, P. (2004). Effects of different N-trimethylchitosans on in vitro/in vivo ofloxacin transcorneal permeation. J Pharm Sci. 93:2851–62.
- Crini, G., Torri, G., Guerrini, M., Morcellet, M., Weltrowski, M., Martel, B. (1997). NMR characterization of N-benzyl sulfonated derivatives of chitosan. Carbohyd Polym. 33:145–51.
- Domard, A., Rinaudo, M., Terrassin, C. (1986). New method for the quaternization of chitosan. Int J Biol Macromol. 8:105–7.
- Illum, L., Farraj, N.F., Davis, S.S. (1994). Chitosan as a novel nasal delivery system for peptide drugs. Pharm Res. 11:1186–9.
- Jintapattanakit, A., Mao, S., Kissel, T., Junyaprasert, V.B. (2008). Physicochemical properties and biocompatibility of N-trimethyl chitosan: effect of quaternization and dimethylation. Eur J Pharm Biopharm. 70:563–71.
- Kotze, A.F., Lueβen, H.L., Leeuw, B.J., Boer, D.B.G., Verhoef, J.C., Junginger, H.E. (1998). Comparison of the effect of different chitosan salts and N-trimethyl chitosan chloride on the permeability of intestinal epithelial cells (Caco-2). J Contr Rel. 51:35–46.
- Kowapradit, J., Opanasopit, P., Ngawhiranpat, T., Apirakaramwong, A., Rojanarata, T., Ruktanonchai, U., Sajomsang, W. (2008). Methylated N-(4-N,N-dimethylaminobenzyl) chitosan, a novel chitosan derivative, enhances paracellular permeability across intestinal epithelial cells (Caco-2). AAPS PharmSciTechnol. 9:1143–52.
- Lapierre, L.A. (2000). The molecular structure of the tight junction. Adv Drug Deliv Rev. 41:255–64.
- Madara, J.L. (1987). Intestinal absorptive cell tight junction are linked to cytoskeleton. Am J Physiol. 253:C171–5.
- McEwan, G., Jepson, M., Hirst, B., Simmons, N. (1993). Polycation-induced enhancement of epithelial paracellular permeability is independent of tight junctional characteristics. Biochim Biophys Acta. 1148:51–60.
- Portero, A., Remunan-Lopez, C., Nielsen, H.M. (2002). The potential of chitosan in enhancing peptide and protein absorption across the TR146 cell culture model-an in vitro model of the buccal epithelium. Pharm Res. 19:169–74.
- Rabea, E.I., Badawy, I.M.E., Rogge, T.M., Stevens, C.V., Hofte, M., Steurbaut, W., Smagghe, G. (2005). Insecticidal and fungicidal activity of new synthesized chitosan derivatives. Pest Manag Sci. 61:951–60.
- Ross, B.P., Toth, I. (2005). Gastrointestinal absorption of heparin by lipidization or coadministration with penetration enhancers. Curr Drug Deliv. 2:277–87.
- Sajomsang, W., Tantayanon, S., Tangpasuthadol, V., Daly, W.H. (2008b). Synthesis of methylated chitosan containing aromatic moieties: chemoselectivity and effect on molecular weight. Carbohyd Polym. 72:740–50.
- Sajomsang, W., Tantayanon, S., Tangpasuthadol, V., Thatte, M., Daly, W.H. (2008a). Synthesis and characterization of N-aryl chitosan derivatives. Int J Biol Macromol. 43:79–87.
- Sandri, G., Rossi, S., Ferrari, F., Bonferoni, M.C., Muzzarelli, C., Caramella, C. (2004). Assessment of chitosan derivatives as buccal and vaginal penetration enhancers. Eur J Pharm Sci. 21:351–9.
- Sashiwa, H., Shigemasa, Y. (1999). Chemical modification of chitin and chitosan 2: preparation and water soluble property of N-acylated or N-alkylated partially deacetylated chitins. Carbohyd Polym. 39:127–38.
- Schipper, N.G., Olsson, S., Hoogstraate, J.A., Boer, D.A.G., Varum, K.M., Artursson, P. (1997). Chitosans as absorption enhancers for poorly absorbable drugs II: mechanism of absorption enhancement. Pharm Res. 14:923–9.
- Sieval, A.B., Thanou, M., Kotze, A.F., Verhoef, J.C., Brussee, J., Junginger, H.E. (1998). Preparation and NMR characterization of highly substituted trimethyl chitosan chloride. Carbohyd Polym. 36:157–65.
- Snyman, D., Hamman, J.H., Kotze, J.S., Rollings, J.E., Kotzé, A.F. (2002). The relationship between the absolute molecular weight and the degree of quaternisation of N-trimethyl chitosan chloride. Carbohyd Polym. 50:145–50.
- Tanima, B., Susmita, M., Ajay, K.S. (2002). Preparation, characterization and biodistribution of ultrafine chitosan nanoparticles. Int J Pharm. 243:93–105.
- Thanoo, B.C., Sunny, M.C., Jayakrishnan, A. (1992). Crosslinked chitosan microspheres: preparation and evaluation as a matrix for the controlled release of pharmaceuticals. J Pharm Pharmacol. 44:283–6.
- Thanou, M., Verhoef, J.C., Junginger, H.E. (2001). Chitosan and its derivatives as intestinal absorption enhancers. Adv Drug Deliv Rev. 50:91–101.