Abstract
The aim of this study was to use different toxicity indices to investigate the effect of N, N-Dimethylacetamide (DMA), Polyethylene glycol 400 (PEG 400), Methyl-Pyrrolidone/aromatic hydrocarbon (MPH), Cremophor® EL, and Dimethylsulfoxide (DMSO) on Calu-3 cells. Membrane perturbation and cytotoxicity were investigated using lactate dehydrogenase (LDH), 3-[4, 5-Dimethylthiazol-2-yl]-2, 5-diphenyl tetrazolinium bromide (MTT), transepithelial electrical resistance (TEER) assessment, sodium fluorescein (SF) permeation, and phalloidin actin staining. The MDH activity of cells treated with DMSO (≤ 4.0 v/v), Cremophor EL (≤ 10% v/v), and PEG 400 (≤ 10% v/v) was not significantly altered (p > 0.5). Similarly, DMSO (≤ 8.0% v/v), Cremophor® EL (≤ 16.0% v/v) and PEG 400 (≤ 32.0% v/v) had no significant effect on LDH (p > 0.05). Conversely, MPH and DMA at very low concentrations (≤ 0.25% v/v) induced cell toxicity. However, up to 2.0% v/v MPH and (DMA) had no effect on TEER and SF permeation. No obvious change in actin staining was observed for DMSO (15%), Cremophor® EL (15%), and MPA (1%). However, the actin architecture for cells treated with DMA (1%) and PEG 400 (15%) appeared significantly different from control. Based on this study Cremophor (≤ 10%), PEG 400 (≤ 5%), and DMSO (≤ 5%), and low concentrations of DMA and MPA (≤ 0.25%) were suitable for in vitro studies with Calu-3 cells.
Introduction
The application of combinatorial chemistry and other drug discovery technologies enables rapid synthesis of large numbers of potential drug candidates that need to be effectively screened for pharmacokinetic parameters along with pharmacological activity (CitationShah et al., 2006). Therefore, an early prediction of bioavailability is critical in the drug selection process to promote lead candidates from discovery to development (CitationSaha, 2002). Very often cultured cells are used early in drug development to assess future therapeutic potential of drug candidates. At times this strategy is challenging considering that more than 70% of new drug candidates are either practically insoluble or very poorly water-soluble. This characteristic may compromise sink conditions, elicit non-specific adsorption, and potentially result in inaccurate estimation of permeability and in vivo bioavailability. Furthermore, most buffers used for in vitro drug delivery studies with tissue culture are very hydrophilic and contain counter ions, which undermines the solubility of poorly water-soluble compounds (CitationDollo et al., 1999). There is a need from the biopharmaceutical standpoint to increase the aqueous solubility of poorly-soluble non-ionizable drugs in order to circumvent low and highly variable absorption generally seen with such compounds (CitationTakahashi et al., 2002). Solubilizers such as polyethylene glycol 400 (PEG 400), N-Dimethylacetamide (DMA), Cremophor® EL, methyl-pyrrolidone/aromatic hydrocarbon (MPH), dimethylsulfoxide (DMSO), cyclodextrins, and co-solvents have been used to optimize the solubility of poorly water-soluble compounds during permeation studies (CitationYamashita et al., 2000; Ginski et al., 2000; CitationSaha & Kou, 2000; CitationTakahashi et al., 2002; CitationWatanabe et al., 2005). In order to avoid potential detrimental effects of solubilizers on epithelial cells, most researchers use arbitrarily chosen low concentrations (usually less than 1%) for transport and metabolism studies. However, some of the solubilizers may in fact be used at much higher concentrations without damaging epithelial cells or significantly altering drug absorption characteristics.
Despite the rising interest in biopharmaceutical investigation with cells of respiratory origin and the usefulness of solubilizers in drug delivery, we were unable to identify reported work that addressed the selection of solubilizers for in vitro biopharmaceutical studies using various toxicity parameters. Although similar studies have been published for Caco-2 cells (CitationDa Violante et al., 2002), the results of such studies may not be extrapolated to the respiratory cells because of differences in cell lineage, morphology, epithelial modeling, rate of cell turnover, and the expression of transporters capable of altering toxicity via efflux mechanisms (CitationHidalgo et al., 1989; CitationGrainger et al., 2006). Therefore, the aim of this study was to identify the concentration range within which to use the selected solubilizers for in vitro drug delivery studies with respiratory epithelial cells (Calu-3 cells) without alteration of cell membrane integrity and viability. As cell viability and barrier characteristics are determined by different toxicity parameters, we also investigated whether routinely employed barrier function assessment using transepithelial electrical (TEER) measurement and paracellular marker permeation optimally capture potential deleterious effects of solubilizers on epithelial cells during in vitro biopharmaceutical drug screening.
Materials and methods
Chemicals
PEG 400, MPH (MP/AH, 80/20 ratio), DMSO, DMA, Cremophor® EL, phalloidin-FITC, isopropyl alcohol, and 4-2-Hydroxyethylpiperazine-N’-2-ethanesulfonic acid (HEPES) were purchased from Sigma (St. Louis, MO).Tissue culture materials including Dulbecco’s modified eagle’s medium–Ham’s F-12 nutrient (D-MEM/F-12), fetal bovine serum (FBS), phosphate buffered saline (PBS), penicillin/streptomycin, glutamax, and phosphate-buffered saline-trypsin were purchased from Invitrogen (Burlington, ON Canada).
Tissue culture
Calu-3 cells were generously donated by Dr. Elizabeth Cowley, Department of Physiology Dalhousie University (Halifax, Canada). The cells were maintained in culture using a standard protocol. The cells were cultured in 1:1 D-MEM/F-12 supplemented with 10% FBS, 100 U/ml penicillin, and 100 mg/ml streptomycin. Initially they were grown submerged in the culture medium for 3 days. After this period, they were maintained at an air–liquid interface by not including culture medium on the donor compartment of the inserts. The receiver compartment contained 1000 µL of 10% FBS that was changed every 48 h until the cells were used for experiments (usually within 7–10 days post-culture establishment). All cell batches were grown on Costar® Transwell® clear (Corning Inc., MA), diameter 12 mm, 0.4 µm pore-size polyester membrane, in the presence of 5% CO2 and 95% O2 at 37°C. Cells for MTT and LDH studies were grown on 24-well plates. For cytokeratin studies, the cells were grown on 12 mm # 1 borosilicate cover glasses (Nalge Nunc, NY).
3-[4, 5-Dimethylthiazol-2-yl]-2, 5-diphenyl tetrazolinium bromide (MTT) assay
Cells grown on 24-well plates were used for the studies. Before the experiments, the cells were washed three times with PBS, pH 7.4, followed by 30 min equilibration in the buffer. The cells were subsequently incubated with various concentrations of the solubilizers for 2 h. At the end of the incubation, 600 µl of MTT (2 mg/ml) was added to each well and incubated for 3 h at 37°C. At the end of the incubation period, the MTT solution was removed and replaced with 3 ml isopropyl alcohol. Formazan crystals were then extracted with the solvent for 2 h on a plate shaker. Mitochondrial dehydrogenase (MDH) enzyme activity, as reflected by formazan formation, was used to assess the viability of the cells. At the end of the extraction period, the extracted formazan was transferred to disposable plastic micro-cuvettes and the absorbance was measured spectrophotometrically at 570 nm using a Cary 50 Bio UV-Visible Spectrophotometer (Varian PTY LTD, Australia). Isopropyl alcohol was used as blank solvent. To improve data quality, absorbance adjustment was made by subtracting the absorbance unit values obtained at 650 nm from the values at 570 nm.
LDH assay
Cells grown on 24-well plates were also used for the studies. At the beginning of the experiments, the cells were washed three times with PBS, pH 7.4, followed by 30 min equilibration in the buffer. Thereafter, the cells were incubated with various concentrations of the solubilizers for 2 h. At the end of the incubation, the activity of released LDH (incubation medium) and unreleased LDH (within the cells) were measured using a kinetic method. In order to determine the amount of LDH inside the cells, 0.5% triton X-100 in PBS was used to disrupt the cells. LDH activity in the lysate and incubation medium were measured using Cary 50 Bio UV-Visible Spectrophotometer at 340 nm wavelength. The LDH substrate consisted (per 80 ml PBS) of pyruvic acid (sodium salt 18.3 mg), NADH (disodium salt 21.3 mg), and NaHCO3 (31.8 mg). The rate of reduction of pyruvic acid (within 3 min at intervals of 10–15 s) due to the presence of LDH was calculated using Cary WinUV software (Varian PTY LTD, Australia). Only the linear part of the curve with a minimum correlation coefficient of 0.990 was used.
The percentage LDHrelease was calculated as the relative decrease in rate of absorbency using the equation below:
where LDHrate supernatant = LDH released into the medium, LDHrate cell = LDH activity of cell homogenate.
Effect of solubilizers on TEER and permeation of paracellular marker (sodium fluorescein)
Cells grown on Costar® Transwell® clear inserts were used for these studies. Immediately before the studies, the cells were washed twice with Hanks’ balanced salt supplemented with 10 mM HEPES buffer and 25 mM glucose, pH 7.4. Subsequently, they were equilibrated in the medium for 30 min at 37°C. The donor compartment was filled with 250 µl of a specific concentration of the solubilizers to be investigated. At pre-determined time points (0–120 min), the cells were checked for epithelial integrity by measuring TEER using EVOM®, which was connected to ‘chop-stick’ electrodes (World Precision Instruments, FL). The TEER values were corrected for background readings due to the filter membrane. In order to confirm the validity of TEER reduction, the flux of 1 mg/ml sodium fluorescein was measured after transport studies with the solubilizers. This was performed for each batch of experiments that was conducted.
Cytokeratin and microscopic studies
For these studies, Calu-3 cells were seeded into multi-well glass slides. The cells were incubated with various concentrations of the solubilizers for 2 h. Cells incubated with PBS served as negative control. After the incubation, the cells were rinsed in PBS and fixed with a 2% paraformaldehyde solution. The cells were further rinsed three times before incubation with 0.1% Triton-X100 solution. The polymeric and oligomeric actins were then stained with Phalloidin-FITC according to manufacturer’s instructions. In brief, phalloidin-FITC was dissolved in DMSO to create a 5 µg/µl stock solution, which was further diluted into PBS to create 0.05 µg/µl solution. The cells were stained with the 0.05 µg/µl Phalloidin-FITC solution for 20 min in the dark before rinsing twice with PBS. Cell preparations were mounted in a 1:1 solution of PBS and glycerol using glass cover slips. The preparations were examined with a fluorescence microscope (Zeiss Axioplan, Toronto, Canada) under a filter with 450–490 nm excitation and 515–565 nm emission wavelengths. Photographs were taken with a high-resolution digital microscopic camera (AxioCamHR, Carl Zeiss MicroImaging GmbH, Göttingen, Germany).
Data presentation and statistical analysis
Unless stated otherwise, all experiments were conducted in triplicate and the results were expressed as mean ± SD. Differences between control and treated groups with respect to LDH release, MDH activity, TEER reduction, and sodium fluorescein permeation were compared using one-way analysis of variance (ANOVA) followed by Dunnet post-test analysis. p < 0.05 was considered significant.
Results
Effect of selected solubilizers on respiratory cells (Calu-3) viability
shows the effect of the DMSO, Cremophor EL®, PEG 400, MPH, and DMA on the viability of Calu-3 cells. The MDH activity of cells treated with PBS (0.6–1.04 ± 0.2 A.U.) served as control. The level of MDH activity as shown by formazan formation was comparable in cells incubated with DMSO and Cremophor EL®. Between 0.25–8.0% v/v DMSO and Cremophor EL® had no significant effect on MDH activity (p > 0.05). For instance, when the concentration of the solubilizers was increased to 32.0% v/v, the enzyme activity was further reduced to ≈ 35 and 45% of their initial activities, respectively. At the same concentration (32% v/v) PEG 400 also had a significant effect on cell viability (p < 0.05). Unlike DMSO, Cremophor® EL and PEG 400 showed no significant MDH activity at relatively high concentrations, while much lower concentrations (0.5% v/v) of MPH and DMA decreased MDH activity by ~ 30%.
Figure 1. Effect of dimethylsulfoxide (DMSO), Cremophor® EL, Polyethylene glycol 400 (PEG 400), Methyl-Pyrrolidone/aromatic hydrocarbon (MPH), and N, N-Dimethylacetamide (DMA) on mitochondrial dehydrogenase activity (OD570–650) of Calu-3 cells. Cells were incubated with solubilizers for 2 h prior to MTT measurement. Data are presented as mean ± SD, n = 3.
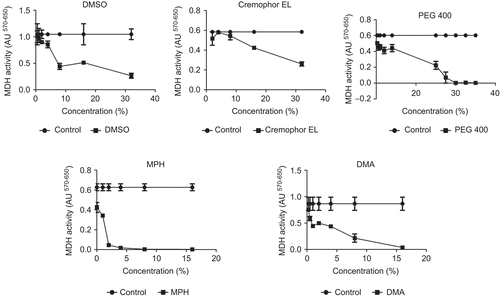
summarizes the effect of the solubilizers on LDH release by the Calu-3 cells. In order to accurately determine the quantity of LDH release due to the solubilizers, it was important to establish the basal release caused by the incubation buffer (PBS). Cells exposed to PBS, without solubilizers, served as negative control. The mean LDH activity in PBS, without solubilizers (supernatant) and within the cells (intracellular) was 138.7 ± 42.3 U/L and 1257 ± 302.7 U/L, respectively. The corresponding percentage LDH release for the negative control was ≈ 10%. This implies that most of the enzyme remained within the cell cytoplasm following incubation with PBS. As observed from MTT assay, Cremophor® EL, DMSO, and PEG 400 were better tolerated by the cells than MPH and DMA. DMSO (1–8% v/v), Cremophor® EL (2–16%) and PEG 400 (2–32% v/v) had no significant effect on LDH (p > 0.05). However, DMSO (16% v/v), Cremophor EL (32.0% v/v), and PEG 400 (40.0% v/v) caused significant LDH release (p < 0.05%). Conversely, MPH and DMA at low concentrations (0.25–0.5% v/v) significantly increased LDH release by 30.0–45.0%. The LDH release after incubating the cells with 4.0% v/v MPH and DMA, respectively, was more than 90.0%.
Table 1. Effect of solubilizers on lactate dehydrogenase activity of Calu-3 cells.
Effect of solubilizers on epithelial barrier characteristics
shows the effect of DMSO, Cremophor® EL, PEG 400, MPH, and DMA on the TEER of Calu-3 cells after incubation for 2 h. TEER measured in HBSS (supplemented with 10 mM glucose, pH 7.4) served as control. Unlike the viability studies (MTT and LDH) where 0.25–0.5% MPH and DMA caused significant toxicity, no significant reduction in TEER was observed after incubating the cells with up to 2.0% v/v MPH and DMA, respectively, for 2 h. However, a 10.0% v/v solution of the solubilizers decreased the TEER of the cells by ~ 80.0%. Similarly, DMSO and PEG 400 (10.0% v/v) reduced TEER by ≈ 50.0% and 40.0%, respectively. In contrast, 10.0% v/v Cremophor® EL had no significant effect on the TEER of the cells. The corresponding TEER changes for 10.0% v/v solubilizer solutions were as follows: 700 ± 1 → 353 ± 5 Ωcm2 (DMSO), 768 ± 1 → 894 ± 5 (Cremophor® EL), 843 ± 34 → 519 ± 18 (PEG 400), 758 ± 56 → 153 ± 4 (MPH), and 751 ± 33 → 169 ± 1 (DMA). Although TEER was significantly reduced (p < 0.05) in most 10% v/v solubilizer solutions, only MPH and DMA resulted in TEER values lower than 300 Ωcm2. Considering that most investigators conduct permeation studies using cells with TEER as low as 250 Ωcm2, it was necessary to investigate whether the observed TEER reduction correlates with significant paracelluar marker (sodium fluorescein) permeation to suggest tight junction disruption.
Figure 2. Effect of dimethylsulfoxide (DMSO), Cremophor® EL, Polyethylene glycol 400 (PEG 400), Methyl-Pyrrolidone/aromatic hydrocarbon (MPH), and N, N-Dimethylacetamide (DMA) on transepithelial electrical resistance (TEER) of Calu-3 cells. Cells were incubated with solubilizers for 2 h, with TEER monitored over the period of time. Data are presented as mean ± SD, n = 3.
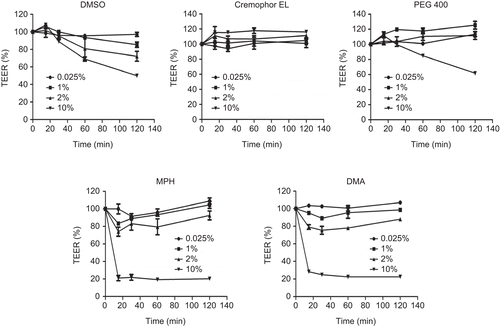
shows the permeation of sodium fluorescein across Calu-3 cells after incubation with various concentrations of the solubilizers. For DMSO, a maximum of ≈ 1.0% sodium fluorescein permeation was observed for a 10% v/v solution. This amount corresponds to ~ 50% TEER reduction from 700 ± 1 → 353 ± 5 Ωcm2. Comparable results (≤ 1.0% permeation) were obtained for 10.0% v/v PEG 400, Cremophor® EL, and 0.25–2.0% v/v MPH and DMA. Conversely, 10.0% v/v DMA and MPH resulted in 17.0 ± 1.0 and 11.9 ± 4.1% sodium fluorescein permeation, respectively. The significant sodium fluorescein permeation for these compounds is a reflection of ~ 80% TEER reduction observed at 10.0% v/v concentration.
Figure 3. Permeation of paracellular marker (sodium fluorescein) following incubation of Calu-3 cells with dimethylsulfoxide (DMSO), Cremophor® EL, Polyethylene glycol 400 (PEG 400), Methyl-Pyrrolidone/aromatic hydrocarbon (MPH), and N, N-Dimethylacetamide (DMA).
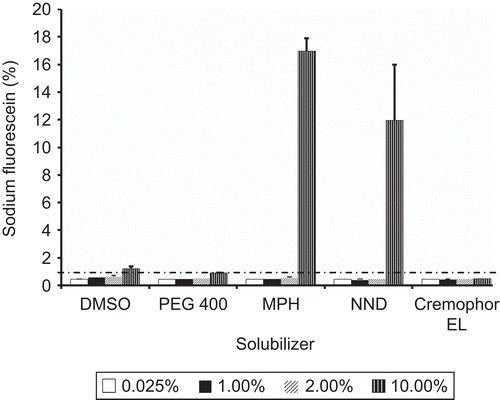
highlights the results of F-actin staining studies. Cells incubated with PBS were used as control. The appearance of actin fluorescent signals in the control (PBS) and test conditions (Cremophor® EL, PEG 400, MPH, DMA, DMSO) were strong. No obvious change in actin staining was observed for the control (PBS), DMSO (1–15%), Cremophor® EL (1–15%), and MPA (0.025–1%). The actin architecture for cells treated with DMA (0.5–1%) and PEG 400 (10–15%) appeared significantly different from control. Although actin staining appeared dense in the two conditions, cells treated with PEG 400 showed continuous actin structure with some cytoskeletal fragments. This observation was peculiar to PEG 400 only. Cells incubated with DMA (0.5–1.0% v/v) appeared round with apparent loss of typical ‘cobble stone’ epithelial appearance.
Discussion
Water-soluble organic solubilizers such as DMSO, PEG 400, Cremophor® EL, DMA, and MPA are often necessary for increasing the aqueous solubility of many compounds during in vitro permeation and metabolism studies. One of the major concerns when using such compounds is the possibility to induce epithelial damage and cell toxicity. Although the toxic potential of the compounds depend on concentration and exposure time, it possibly varies from tissue-to-tissue and cell line-to-cell line. Therefore, concentration ranges reported to be tolerated by intestinal epithelial cells may be toxic to respiratory cells due to differences in cell physiology, solute transport, and efflux mechanisms. In this study we investigated the effects of DMSO, PEG 400, Cremophor® EL, DMA, and MPA on Calu-3 cells. Cytotoxicity assays and epithelial tight junction integrity screening methods were used to capture various aspects of cell toxicity. While the MTT assay specifically detects mitochondrial metabolic activity, the LDH assay detects leakage of cytosolic LDH, an indication of cell membrane dysfunction or damage. On the other hand TEER measurement, sodium fluorescein permeation, and actin staining provided information on epithelial tight junction integrity. Calu-3 cell line was selected as a model for the respiratory system because of its wide range of applications as an in vitro drug delivery model (CitationForbes & Ehrhardt, 2005). The chosen solubilizers have been used for solubilizing many pharmaceutical compounds (CitationIngels & Augustijns, 2003).
A comparison of the TEER results of the solubilizers with paracelluar marker permeation revealed that only 10% v/v MPH and DMA resulted in remarkable perturbation of the Calu-3 tight junctions, whereas DMSO, PEG 400, and Cremophor® EL (up to 10 v/v) had negligible effects. Also, cytoskeletal staining indicated no cell structural damage by DMSO and Cremophor® EL for concentrations as high as 15.0% v/v. However, evidence of actin damage was seen for PEG 400 (15%) and DMA (0.5%). Based on TEER, sodium fluorescein permeation, and actin staining studies, DMSO, Cremophor EL, and PEG 400 (≤ 10%), as well as DMA and MPA (≤ 0.5%) appeared suitable for in vitro studies. Although TEER and paracelluar marker permeation are generally accepted indicators of tight junction permeability, these methods do not detect non-tight junction related epithelial membrane perturbation and as such are not good indicators of cell viability (CitationCalabro et al., 2008). Therefore, more accurate indicators of cytotoxicity such as MTT and LDH were recommended as additional toxicity screening tools during in vitro studies (CitationSambruy et al., 2001). In order to suggest the suitable concentration ranges of the tested solubilizers for in vitro biopharmaceutical screening with Calu-3 cells, a comparison of epithelial junction perturbation and cytotoxicity data is important. The results of the cytotoxicity studies did not correlate with the TEER and sodium fluorescein permeation studies for all the solubilizers, especially for DMA and MPH. For instance, MPH and DMA significantly decreased cell viability based on formazan formation and LDH leakage at 0.25% v/v, but had no effect on epithelial integrity at up to 2% v/v. The lack of correlation may stem from the fact that, whereas toxicity studies with MTT and LDH yielded information on cell membrane damage, TEER and related studies estimated tight junction perturbation. As tight junction disruption and cytotoxicity assays provide different toxicity information, both types of studies ought to complement rather than confirm each other. Therefore, based on the combined results of the various toxicity indices used in this study, we suggest that Cremophor® EL (≤ 10), PEG 400 (≤ 5%), and DMSO (≤ 5%) can be used for in vitro biopharmaceutical studies with Calu-3 cells, without significantly altering epithelial membrane and tight junction integrity. We do not recommend the use of MPH and DMA because the concentrations of these compounds that resulted in cell toxicity may not sufficiently increase the solubility of test compounds for in vitro studies. However, if these compounds must be used, low concentrations should be used.
The use of reasonably high concentrations of solubilizers for in vitro permeation studies with Caco-2 and other epithelial cells have been reported (CitationIngels & Augustijns, 2003; CitationHamid et al., 2009). The results of our study were in agreement with some of the published data for DMSO, Cremophor EL, and PEG 400, but different for DMA and MPH. For instance, CitationHamid et al. (2009) reported that up to 10% PEG 400, DMSO, and Cremophor® EL were suitable for drug delivery studies with Caco-2 cells and isolated rat intestine. CitationIngels and Augustijns (2003) recommended the use of 3% DMA and 2.5% MPH for in vitro studies with Caco2 cells. The disparity between the studies from which the recommendation was made and our data may be explained by differences in toxicity indices. In our study, both tight junction and cytotoxcity parameters were used for assessing toxicity, whereas their studies used only tight junction perturbation as the sole toxicity endpoint. As TEER mainly represents the resistance of the epithelial tight junctions (Rt) and not sensitivity to mucosal (Rm) and serosal (Rs), TEER values reported during transport studies may be misleading as cell recovery from tight junction damage does not necessary imply recovery from cytotoxicity (CitationMukherjee et al., 2004). Furthermore, it may be possible that Caco-2 cells intrinsically tolerate DMA and MPH better than Calu-3 cells.
In addition to toxicity, the selection and use of high concentrations of solubilizers for in vitro drug delivery studies may depend on study design (e.g. passive diffusion vs active uptake or efflux). Some solubilizers may have little or no effect on passive diffusion, but significantly inhibit active uptake and efflux transport mechanisms, respectively (CitationMudra & Borchardt, 2010). Therefore, if active transport mechanisms are envisaged, it is important to screen the effect of the selected solubilizers on active drug transport.
Conclusions
In this study, we identified the concentration ranges within which to use the selected solubilizers for in vitro drug delivery studies with Calu-3 cells without significant alteration of cell membrane integrity and cell viability. Cremophor® EL (≤ 10), PEG 400 (≤ 5%), and DMSO (≤ 5%) may be used for in vitro biopharmaceutical studies with Calu-3 cells without altering epithelial membrane and tight junction integrity. We do not recommend the use of MPH and DMA with Calu-3 cells because the concentrations of these compounds that resulted in cell toxicity may not sufficiently increase the solubility of test compounds to warrant their use. Furthermore, data from this study buttressed the fact that the use of TEER measurement and paracellular marker permeation alone as toxicity indicators after permeation studies may not adequately capture all aspects of cell toxicity following exposure to drugs or excipients during in vitro permeation studies.
Declaration of interest
This study was supported by a grant from the Dalhousie University Pharmacy Endowment Fond and College of Pharmacy, Dalhousie University, Nova Scotia, Canada. The authors report no conflicts of interest. The authors alone are responsible for the content and writing of the paper.
References
- Calabro, A.R., Konsoula, R., Barile, F.A. (2008). Evaluation of in vitro cytotoxicity and paracellular permeability of intact monolayers with mouse embryonic stem cells. Toxicol In Vitro. 22:1273–84.
- Da Violante, G., Zerrouk, N., Richard, I., Provot, G., Chaumeil, J.C., Arnaud, P. (2002). Evaluation of the cytotoxicity effect of dimethyl sulfoxide (DMSO) on Caco2/TC7 colon tumor cell cultures. Biol Pharm Bull. 25:1600–3.
- Dollo, G., Le Corre, P., Chollet, M., Chevanne, F., Bertault, M., Burgot, J.L., Le Verge, R. (1999). Improvement in solubility and dissolution rate of 1, 2-dithiole-3-thiones upon complexation with beta-cyclodextrin and its hydroxypropyl and sulfobutyl ether-7 derivatives. J Pharm Sci. 88:889–95.
- Forbes, B., Ehrhardt, C. (2005). Human respiratory epithelial cell culture for drug delivery applications. Eur J Pharm Biopharm. 60:193–205.
- Ginski, M.J., Taneja, R., Polli, J.E. (1999). Prediction of dissolution-absorption relationships from a continuous dissolution/Caco-2 system. AAPS PharmSci. 1:E3.
- Grainger, C.I., Greenwell, L.L., Lockley, D.J., Martin, G.P., Forbes, B. (2006). Culture of Calu-3 cells at the air interface provides a representative model of the airway epithelial barrier. Pharm Res. 23:1482–90.
- Hamid, K.A., Katsumi, H., Sakane, T., Yamamoto, A. (2009). The effects of common solubilizing agents on the intestinal membrane barrier functions and membrane toxicity in rats. Int J Pharm. 379:100–8.
- Hidalgo, I.J., Raub, T.J., Borchardt, R.T. (1989). Characterization of the human colon carcinoma cell line (Caco-2) as a model system for intestinal epithelial permeability. Gastroenterology 96:736–49.
- Ingels, F.M., Augustijns, P.F. (2003). Biological, pharmaceutical, and analytical considerations with respect to the transport media used in the absorption screening system, Caco-2. J Pharm Sci. 92:1545.
- Mudra, D.R., Borchardt, R.T. (2010). Absorption barriers in the rat intestinal mucosa. 3: Effects of polyethoxylated solubilizing agents on drug permeation and metabolism. J Pharm Sci. 99:1016–27.
- Mukherjee, T., Squillantea, E., Gillespieb, M., Shao, J. (2004). Transepithelial electrical resistance is not a reliable measurement of the Caco-2 monolayer integrity in Transwell. Drug Deliv. 11:11–8.
- Saha, P. (2002). Effect of bovine serum albumin on drug permeability estimation across Caco-2 monolayers. Eur J Pharm Biopharm. 54:319–24.
- Saha, P., Kou, J.H. (2000). Effect of solubilizing excipients on permeation of poorly water-soluble compounds across Caco-2 cell monolayers. Eur J Pharm Biopharm. 50:403–11.
- Sambruy, Y., Ferruzza, S., Ranaldi, G., De Angelis, I. (2001). Intestinal cell culture models: applications in toxicology and pharmacology. Cell Biol Toxicol. 17:301–17.
- Shah, P., Jogani, V., Bagchi, T., Misra, A. (2006). Role of Caco-2 cell monolayers in prediction of intestinal drug absorption. Biotechnol Prog. 22:186–98.
- Takahashi, Y., Kondo, H., Yasuda, T., Watanabe, T., Kobayashi, S., Yokohama, S. (2002). Common solubilizers to estimate the Caco-2 transport of poorly water-soluble drugs. Int J Pharm. 246:85–94.
- Watanabe, E., Kinoshita, N., Takahashi, M., Hayashi, M. (2005). Prediction of absorbability of poorly water-soluble drugs based on permeability obtained through modified in vitro chamber method. Drug Metab Pharmacokinetic. 20:428–34.
- Yamashita, S., Furubayashi, T., Kataoka, M., Sakane, T., Sezaki, H., Tokuda, H. (2000). Optimized conditions for prediction of intestinal drug permeability using Caco-2 cells. Eur J Pharm Sci. 10:195–204.