Abstract
Methicillin-resistant Staphylococcus aureus (MRSA) have become increasingly prevalent as nosocomial pathogens, especially in burn patients, which is the leading cause of their death. A drug delivery system of chitosan-collagen hydrogel incorporated with lysostaphin (CCHL) based on the lysostaphin gauze was developed for MRSA infected burn wounds. CCHL scaffold consisted of numerous interconnected sphericles and tubular bodies with an average diameter of 100–200 µm, 20–60-fold swelling, high water retention capacity, and cell proliferation properties. The minimal inhibitory concentration of CCHL was 0.053 U/mL. By the second week after its application on MRSA infected third-degree burn wounds, no bacteria could be detected and the burn wounds had started healing. Therefore, CCHL should be studied further as a promising candidate of burn treatment dressing against MRSA infections for clinics.
Introduction
Methicillin-resistant Staphylococcus aureus strains (MRSA) are increasingly prevalent as tenacious nosocomial pathogens, especially in patients with burn wounds (CitationChurch et al., 2006). The extant drugs available such as silver sulfadiazine, mupirocin, and others are incapable of suppressing the infections (CitationUygur et al., 2009). Biomaterials as drug delivery play a vital role in providing extracellular matrix environments for tissue regeneration and antibacterial properties (CitationMa, 2008). Several studies have reported bioactive materials as burn dressings such as pure chitosan (CitationClasen et al., 2006; CitationWittaya-Areekul & Prahsarn, 2006), chitosan/collagen matrix, and other biomimetic materials strategies (CitationWeng et al., 2008).
Lysostaphin is a proteolytic enzyme (metalloendopeptidase) produced by one species of Staphylococcus (simulans) which is specifically capable of cleaving the cross-linking pentaglycine bridges in the cell walls of Staphylococcus aureus (S.aureus) (CitationKokai-Kun et al., 2003). In 2005, a lysostaphin gauze (Approved by State Food and Drug Administration 2005, No. 3641528) was developed by Shanghai Hi-Tech-Bioengineering Co., Ltd (Shanghai, China), that is fairly effective for the treatment of burn patients with MRSA infection in clinics.
The study aims at the development of a new type of dressing by incorporating lysostaphin into chitosan-collagen hydrogels which were prepared by cross-linking chitosan hydrochloride with collagen to produce some unique properties in swelling, water retention, hygroscopic, rheology, cell proliferation, biocompatibility with lysostaphin, and in vivo efficiency for MRSA infected third-degree burn wounds.
Materials and methods
Chemicals and reagents
Chitosan hydrochloride was purchased from Zhejiang Golden-Shell Biochemical Co., Ltd. (Zhejiang, China). 1-Ethyl-3-(3-dimethylaminopropyl) carbodiimide hydrochloride (EDAC) was obtained from Shanghai Medpep Cp., Ltd. (Shanghai, China). The hydrolyzed collagen powder (Mn = 3000 Da) was obtained from Beijing Huada Jierui Biotechnology Co., Ltd. (Beijing, China). Lysostaphin (139 U/mg) was produced by Shanghai High-Tech United Bio-Technological R&D Co. Ltd. (Shanghai, China).
Cell culture and bacterial strain
Human embryonic lung fibroblast (HLF) cells were obtained from the Institute of Biochemistry and Cell Biology (Shanghai, China). Cells were maintained in α-MEM supplemented with FBS (10%, final concentration), glutamine (2 mM), penicillin (100 U/mL), and streptomycin (100 µg/mL), in a humidified incubator at 37°C with 5% CO2/95% air.
The microbe used in this study was MRSA (ATCC 43300).
Animals
Twenty-five New Zealand White rabbits were purchased from the Animal Care Center, Fudan University. The protocol was reviewed and approved by the Institutional Animal Care and Use Committee, Fudan University, China.
Preparation of chitosan-collagen hydrogels incorporated with lysostaphin (CCHL)
Chitosan-collagen hydrogels were prepared according to CitationChen et al. (2006) with modifications. The compositions of them were reported in . Briefly, a 0.1 g portion of chitosan hydrochloride was dissolved in double distilled water to make a 1 wt% aqueous solution. Then collagen was added to the above solution in order to have a mixing weight ratio, x, equal to 1:1, 1:3, 1:6, and 1:8, where x = weight of chitosan hydrochloride/weight of collagen. Then activating agent EDAC was added to a final concentration of 63 mM. The mixture was stirred (100 rpm) for 2 h at room temperature, then transferred to dialysis bags (Mw cut-off 8000∼10,000 Da) and dialyzed exhaustively against distilled water at 4°C for 2 days with several changes of fresh water. After that, the dialyzed specimen was dehydrated using PEG 20,000 to its original volume and centrifuged at 6000 rpm to eliminate the entrapped air bubbles. CCHL were prepared by incorporating lysostaphin into purified hydrogels. They were well-mixed, frozen at −80°C, and, after lyophilization, the CCHL scaffold was stored at 4°C.
Table 1. The optimized composition of the components for chitosan-collagen hydrogels.
Microstructure of the CCHL scaffold
To investigate the microstructure, sections of the CCHL scaffolds were coated with a thin layer of palladium gold alloy and examined by a scanning electron microscope (SEM515, Philips Electronic instruments, The Netherlands).
Rheological analysis
The rheology including the storage modulus G’, loss modulus G’’, and complex viscosity h* of the hydrogels were measured with frequencies ranging from 1–100 rad/s using a rheometer (ARES, TA Instruments, United States). All dynamic rheological data were checked as a function of strain amplitude to ensure that the measurements were performed in the linear viscoelastic region.
Swelling capacity
The swelling behavior of CCHL scaffolds was performed as in CitationLiu et al. (2008), with slight modifications. CCHL scaffolds were incubated in distilled water at room temperature. The scaffolds were weighed (W0), and immersed in glass vials containing distilled water. At defined time intervals, the swollen hydrogels were weighed (Wt) immediately after the removal of the surface water by blotting lightly between two pieces of filter paper.
Degree of swelling, Q, was expressed as the weight of absorbed water per gram of dry CCHL scaffolds during regular time intervals. Q = (Wt − W0)/W0. The gradual erosion of scaffolds was also studied by observing and recording the time at which Wt began to decrease.
Water retention capacity
This was performed as in CitationLiu et al. (2006), with slight modifications. The equilibrium swelling hydrogels (Wi) were kept in a humidity chamber of RH 43% and weighed at regular intervals (Wt). The percentage water loss was calculated using the following formula:
Hygroscopic capacity
The CCHL scaffolds (W0) were kept in a humidity chamber of R.H. 43% and 81%, and weighed at regular intervals (Wt). The percentage water absorption was calculated as follows:
Cell proliferation assay
HLF cells were seeded in wells of a 96-well plate at a density of 5000 cells per well. After 24 h incubation, the culture medium was removed and replaced with 10, 30, and 50 µL of chitosan-collagen hydrogels, and the remaining fresh medium. Untreated cells served as the control. After incubation for 2, 24, and 48 h cell numbers were measured using MTS assay reagents according to the manufacturer’s instructions (Promega, Madison, WI).
Assessment of structural integrity of lysostaphin
The efficiency of chitosan-collagen hydrogel in the protection of incorporated lysostaphin was evaluated as the structural integrity of lysostaphin released from the hydrogels and detected by SDS-polyacrylamide gel electrophoresis (SDS-PAGE) in a comparative study with the native lysostaphin. The lysostaphin released after 3 h from hydrogels were used in the study. Electrophoresis was run at 30 mA until the dye band reached the gel bottom. The gel was then removed, stained with Coomassie blue, and destained.
Effect of CCHL on the activity of lysostaphin
Effect of chitosan-collagen hydrogels with different weight ratios of chitosan to collagen on the activity of lysostaphin was assessed by incorporating lysostaphin into them. Then 100 µL samples were withdrawn from the above hydrogels, and their enzymatic activity measured as in the method of CitationZhou et al. (1988). On the other hand, the effect of CCHL on the activity of lysostaphin was also assessed at 39°C. CCHL and lysostaphin aqueous solution were prepared at the same lysostaphin concentration of 10 U/mL, incubated in a water bath (39°C) for 48 h. Then 100 µL samples were withdrawn from CCHL and aqueous solution, respectively, and their enzymatic activity measured.
In vitro release of lysostaphin from CCHL
Lysostaphin released from the CCHL scaffold (1:6) to phosphate buffer solution (PBS) (pH 7.4) was carried out in a shaker incubator of 90 rpm at 37°C. The certain amount of CCHL scaffold was immersed in 15 mL PBS. One milliliter of samples were withdrawn periodically and replaced with the same amount of PBS. The concentration of lysostaphin was analyzed by its enzymatic activity and pooled.
To simulate the released condition in vivo, CCHL (1:6) was placed into wells (7 mm in diameter) in petri dishes and incubated at 37°C for 18 h. The control was the lysostaphin solution. Diffusion of lysostaphin was detected by the Commassie blue staining assay and photographed using an Olympus digital camera.
Antibacterial assay
The antibacterial assay was done as in CitationMourtzinos et al. (2009) with certain modifications. The antimicrobial activity of CCHL was determined with the minimum inhibitory concentration (MIC), the zone inhibition assay and killing rate. CCHL (1.0 mL) were poured into petri dishes with 20 mL sterile media, and the mixture homogenized immediately. When the agar solidified, suspensions of the bacteria (1.0 mL) with the concentration of 107 CFU/mL were spread onto the surface of petri dishes and incubated at 37°C for 24 h. MIC was the concentration at which no bacterial growth was detected.
Suspension of the bacteria (107 CFU/mL) was mixed with media and poured into petri dishes. Several wells with diameters (7 mm) were made, and 40 µL of different doses of CCHL (0, 1, 3, 5, and 10 U/mL) and lysostaphin solution were added. The dishes were incubated at 37°C for 24 h, and the diameters of inhibitory zones (in mm) were measured.
The filter paper discs were individually impregnated with the bacterial suspension, and incubated with different doses (0, 1, 3, 5, and 10 U/mL) of CCHL. After incubation for different time intervals (10 min and 1 h), the paper discs were diluted with sterile saline and the diluted bacterial suspensions were poured onto the dishes. After incubation at 37°C for 48 h, the number of CFU was counted.
Assessment of CCHL in accelerating wound healing of MRSA infected third-degree burn wounds
Rabbits were chosen in these experiments because they were liable to S.aureus infection. In the preliminary study CCHL and lysostaphin gauze were applied to determine their efficacy. Results showed on the 19th day the wounds area was greatly shrunk to 25% and 75% in the CCHL- and lysostaphin gauze-treated MRSA infected third-degree burn wounds, respectively, and no S.aureus could be detected too. However, in the administration of lysostaphin gauze it showed troublesome dressing change, and insufficiency to keep prolonged moisture of the wound. Therefore, in the study the following treatments were applied except lysostaphin gauze: CCHL (1:6) with 10 U/mL, chitosan-collagen hydrogel (1:6), and saline.
Third-degree burn wounds were established on the dorsum skin of New Zealand White rabbits. MRSA (ATCC 43300) was inoculated in agar medium at 37°C for 48 h. The rabbits were anesthetized by chloral hydrate (0.3 g/kg) and two symmetrical burn wounds (3 cm × 3 cm) were made on the dorsum skin by an electronic temperature controller (80°C, 15 s). Two days after burning the eschar was excised, 0.2 ml (109 CFU/mL) of bacterial suspension was injected on each wound, after infection the wound covered with vaseline gauze, and cast cap. Elizabeth circle was worn on the rabbit’s neck to prevent its licking the wounds. As the wounds were infected, the above treatments were applied separately. CCHL and chitosan-collagen hydrogel were applied at 0, 3, 7, and 14 days after infection, and saline was administered once daily. Then pictures of the wound were taken using a digital camera at 3, 7, and 14 days. The zero day photographs were not taken due to the interference of the cap, which dropped off at 3 day. The dimension of the wound was measured by a ruler, and the wound area change was calculated using % wound area = At/A0 × 100, where A0 was the original wound area after infection and At was the area of wound at 1 and 2 weeks, accordingly.
The bacterial load on the wound was obtained as below: Two pieces of sterilized Xinhua filter paper (1.0 cm2 × 1.0 cm2) were covered on the wound area for 10 s, then mixed with 1 mL of sterile saline. The diluted bacterial suspension was made for bacteria count. Two weeks after treatment, the rabbits were sacrificed and the tissues were fixed in 10% formaldehyde solution,sectioned, stained using hematoxylin and eosin, and examined microscopically.
Statistical analysis
Data were expressed as mean ± standard deviation (SD). Statistical analysis was performed using SPSS v14.0 (SPSS, Inc, Chicago, IL). The one-way analysis of variance (ANOVA) test was followed by the Bonferroni test to evaluate the statistical significance of the effect of different treatments on wound healing and MRSA load in MRSA infected third-degree burn wounds.
Results
Structure of the CCHL scaffolds
The CCHL scaffolds in section under SEM () revealed an inner structure of inter-connected sphericles and tubules with average diameters of 100–200 μm. Lysostaphin was found randomly on linings of the scaffold ().
Figure 1. Microstructure of CCHL scaffold. Sections of CCHL scaffolds of different ratios of chitosan hydrochloride to collagen (w/w): a, 1:1; b, 1:3; c, 1:6; d, 1:8, showing interconnected spherical and tubular bodies of 100–200 µm in diameter. Lysostaphin was seen dispersed randomly on linings (solid arrow).
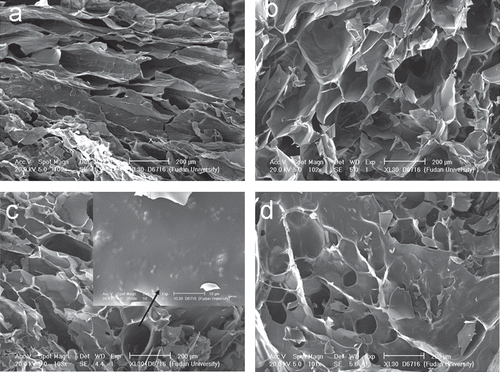
Rheological analysis
The modulus changes were subsequently monitored on gelatin and structure of hydrogels. The frequency dependencies of the storage modulus G’; the loss modulus G” and the complex viscosity were shown in . For the hydrogel (1:1) G” was higher than G’ in the whole frequency range. For the hydrogel (1:3) G’ dominated over G” over the entire range of frequencies covered and G’ remained almost unchanged with different frequency indicating that it behaved like a highly elastic physical gel. With the increase of collagen, a decrease in storage, loss modulus, and complex viscosity were observed. As the chitosan hydrochloride-to-collagen ratio (w/w) reached 1:8, G” had reverted to higher value than G’. The complex viscosity decreased with increasing shear rate, indicating its pseudoplastic characteristic.
Swelling, water retention, and hygroscopic capacity
Swelling capacity was shown in . All three scaffolds of different chitosan hydrochloride-to-collagen ratio reached the maximum swelling ratio within 4 h. The swelling degree of the CCHL scaffold (1:3) was 28, lower than that of the other two (1:1 and 1:6), that were 53 and 55, respectively. In comparison, the CCHL scaffold (1:8) degraded rapidly and disintegrated upon handling for 15 min.
Figure 3. Swelling, water retention and hygroscopic capacity of CCHL scaffolds of different ratios of chitosan hydrochloride to collagen (w/w) (mean ± SD, n = 3). A, Swelling ratio of different chitosan-collagen hydrogels in water; B, Water loosing rate of different hydrogels; C, Hygroscopic rate of different hydrogels in R.H. 81%; and D, Hygroscopic rate of different hydrogels and in R.H. 43%.
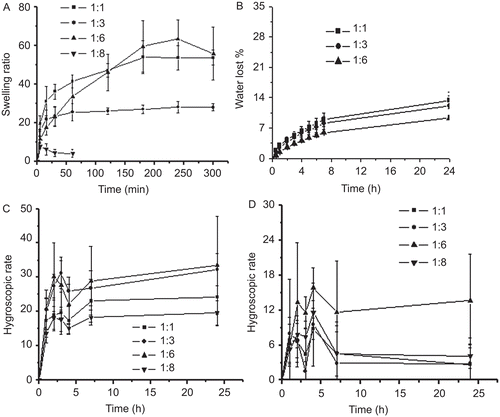
Water loss of equilibrium swelling hydrogels (1:1, 1:3, and 1:6) was ∼ 15% within 24 h (). The four CCHL scaffolds kept under R.H. 81% showed their ranges of hygroscopic rate from 19–33% () and from 2.6–13% under R.H. 43% (). The CCHL scaffold (1:6) showed a relative higher hygroscopic rate than all the others under both R.H. conditions.
Cell proliferation assay
Cell proliferation of chitosan-collagen hydrogel effect on HLF cells was shown in . The results showed that the hydrogel induced HLF proliferation. After 24 h incubation, with 20 and 30 µL of the hydrogel, they induced 4–5-fold increments in the number of cells compared to the control.
Assessment of structural integrity of lysostaphin
The stability and integrity of the released lysostaphin was assessed using SDS-PAGE. The band of released lysostaphin was identical to that of lysostaphin aqueous solution (), showing that the handling and the environment did not affect the integrity of lysostaphin.
Effect of CCHL on the activity of lysostaphin
The enzymatic activities of lysostaphin in chitosan-collagen hydrogels with different weight ratios of chitosan to collagen 1:1, 1:3, 1:6, and 1:8 were 9.0, 12.8, 14.3, and 13.5 U/mL compared to 11.7 U/mL of lysostaphin solution. Lysostaphin in solution incubated at 39°C for 48 h lost ∼ 50% of its activity. On the other hand, the enzymatic activity of lysostaphin incorporated in CCHL remained intact, or showed even slightly higher activity than that of the lysostaphin aqueous solution during the incubation period.
In vitro release of lysostaphin from CCHL
The accumulative lysostaphin released from CCHL to PBS was shown in . About 60% of the drug was released after 3 h. Blue bands which indicated the diffusion of lysostaphin around the well appeared in the petri dish () and CCHL shared similar blue bands to that of lysostaphin aqueous solution.
Antibacterial properties of CCHL
CCHL showed strong bacterial activity against MRSA with the minimum inhibitory concentration (MIC) 0.053 U/mL. Clear-cut inhibitory zones of the CCHL and lysostaphin solution were observed for MRSA too (). The inhibitory zones were consistent with the diffusion of lysostaphin in the petri dish, which indicated the diffusion of lysostaphin from CCHL leading to the subsequent growth inhibition of S.aureus in the culture medium. The viable colonies of bacteria decreased as the contact time extended. As lysostaphin doses increased to 10 U/mL, complete clearance of MRSA was noted.
MRSA load after treatment with CCHL
Three days after MRSA inoculation, cultures of wound areas yielded high bacteria counts of 6–7 log10 CFU/mL (), suggesting infection of the wounds. The wounds of the CCHL treated group showed the rapid decrease of bacteria compared to the saline group (one-way ANOVA, Bonferroni test, p < 0.05) (), and no bacteria could be detected by the second week. The other two groups reached bacterial counts above 8 log10 CFU/mL.
Table 2. Mean MRSA counts (log10 CFU/mL) from burn wound treated with CCHL, chitosan-collagen hydrogel, and saline.
Wound healing
The healing of burn wounds at 1 and 2 weeks after treatment was shown in and . The wound size in the CCHL treated group was the smallest of the three groups (one-way ANOVA, Bonferroni test, p < 0.01), and 1 week after treatment tissue granulation formed, and the burn wounds had started healing. The H&E staining wound tissues applied with CCHL at 1 and 2 weeks (results not shown) demonstrated that there was extensive inflammatory cell infiltration in infected wounds at 1 week, whereas the skin was almost healed by the second week, and intact hair follicle cells with prominent blood vessels were found. Extended suppuration appeared in burn wounds of the other two groups, and wound size increased 2–4-fold compared to the original wound size.
Figure 8. Development of wound healing in MRSA infected New Zealand rabbits treated with (A) CCHL; (B) chitosan-collagen hydrogel; and (C) saline during 2 weeks. Solid arrow indicated suppuration (S).
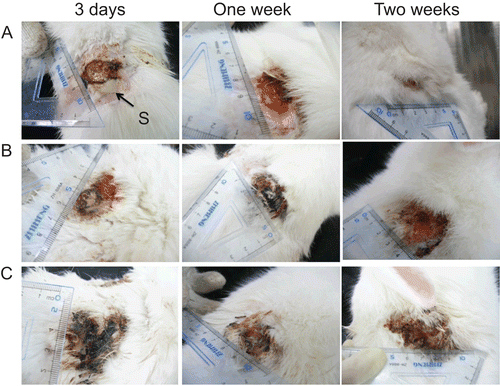
Figure 9. Wound size changes in MRSA infected New Zealand rabbits treated with CCHL, chitosan-collagen hydrogel, and saline during 2 weeks (mean ± SD, n = 6~9). ** p < 0.01 compared with other groups (one-way ANOVA, Bonferroni test); **# p < 0.01 compared with the saline group (one-way ANOVA, Bonferroni test).
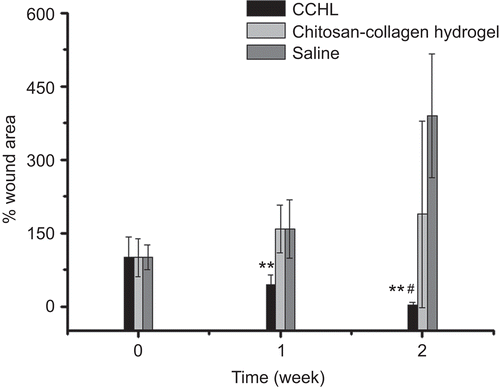
Discussion
The development of CCHL in the present study showed that the new-type wound dressing could accelerate burn wound healing rate and clear out MRSA in MRSA infected third-degree burn wounds. CCHL as burn wound dressing overcame disadvantages relative to application of the lysostaphin gauze such as its poor absorbency, insufficiency to keep prolonged moisture of the wound, and tissue granulation into the gauze mesh causing secondary damage to the healing wound, etc. At the same time CCHL provided an environment different from lysostaphin aqueous solution which was liable to enzymatic and thermal degradation, and short duration of drug retention at wound sites. Besides, CCHL in the form of hydrogel was of easy application for a variety of irregular burn wounds.
Chitosan hydrochloride and collagen were chosen as the material of forming the hydrogel due to their dissolution, biocompatibility, and bioactive properties such as hemostasis and promotion of skin fibroblasts (CitationYates et al., 2007). The rheology, swelling, and water retention capacity of the forming hydrogels could be tailored by changing the composition of chitosan hydrochloride and collagen. The storage moduli of hydrogels (1:3 and 1:6) were much higher than other hydrogels, indicating their greater stability of inter-chain junctions, but not so with less or more collagen amounts, and leading in formation of the mechanically weak hydrogels. Therefore, hydrogels (1:3 and 1:6) could provide elasticity and mechanical stability associated with the underlying connective tissues as burn dressings (CitationJeong et al., 2005). CCHL scaffolds (1:1 and 1:6) had higher swelling behavior than the scaffold (1:3), which might be due to their lower cross-linking, which correlated well with our previous studies that described the swelling behavior of chitosan-ethylenediaminetetraacetic acid hydrogel films (CitationCui et al., 2009). It is interesting that the hydrogel (1:6) with higher swelling capacity and elasticity also had a higher water retention and hygroscopic capacity. Therefore, the hydrogel will be able to provide an environment to the wound surface for much extended time, and thereby slow down scar formation (CitationDe Coninck et al., 1996). The research also indicated that the hydrogel had excellent biocompatibility with HLF cells, and was capable of promoting the branching and proliferation of the cells.
Its appearance that lysostaphin randomly distributed on linings of CCHL scaffolds could stabilize the integrity and activity of lysostaphin. The exudate level may have a significant influence on the lysostaphin release from CCHL (CitationSteffansen & Herping, 2008). Therefore, the release properties of lysostaphin in high and low exudate conditions were both evaluated in vitro. The results indicated that CCHL were capable of efficiently killing MRSA in vitro, and it also showed that the chitosan-collagen hydrogels without lysostaphin did not have bactericidal activity, which demonstrated that it was lysostaphin that had anti-MRSA activity.
Bacterial infection was one of the leading causes to delayed wound healing. The wound area became expanded in the hydrogel and saline treated groups due to MRSA infection. Lysostaphin was highly effective in lysing S.aureus cells with MIC 0.053 U/mL. CCHL and lysostaphin gauze both containing lysostaphin could efficiently clear out MRSA infected in third-degree burn wound, and lead to wound contraction compared to the control. CitationDesbois et al. (2010) also showed that lysostaphin could synergistically combine with the antimicrobial peptide ranalexin to significantly reduce MRSA compared with the untreated control in a rabbit model of wound infection. However, they just applied gauze as the wound dressing and did not study the wound healing rate. Our results showed that CCHL has a significant effect upon wound healing compared to the lysostaphin gauze. It found up to a 100% healing rate for CCHL in a 2-week period. Previous studies mentioned nearly the same percentages of healing with pancreas-derived stem cells on scaffold, but this was not infection wounds (CitationSalem et al., 2009). In general, the results suggested that CCHL was a promising dressing for MRSA infected burn wounds, although in our studies there were not sufficient positive controls such as bactroban, silver sulfadizine, and others.
Conclusions
We had developed a new-type burn dressing CCHL which provided an environment to keep prolonged moisture of the wound and stabilize the activity of lysostaphin. It could accelerate burn wound healing rate and clear out MRSA in MRSA infected third-degree burn wounds. Therefore, we suggested CCHL might be used as a candidate of burn dressing, in particular against MRSA infection for clinical application.
Acknowledgements
We sincerely express our gratitude to Professor Deming Su for writing assistance.
Declaration of interest
This work was supported by a grant from the Science and Technology Commission of Shanghai Municipal Govt. (No. 08441900700).
References
- Chen, R.N., Wang, G.M., Chen, C.H., Ho, H.O., Sheu, M.T. (2006). Development of N,O-(Carboxymethyl) chitosan/collagen matrix as a wound dressing. Biomacromolecules. 7:1058–64.
- Church, D., Elsayed, S., Reid, O., Winston, B., Lindsay, R. (2006). Burn wound infections. Clin Microbiol Rev. 19:403–34.
- Clasen, C., Wilhelms, T., Kulicke, W.M. (2006). Formation and characterization of chitosan membranes. Biomacromolecules. 7:3210–22.
- Cui, F.Y., He, C.B., He, M., Tang, C., Yin, L.C., Qian, F., Yin, C.H. (2009). Preparation and evaluation of chitosan-ethylenediaminetetraacetic acid hydrogel films for the mucoadhesive transbuccal delivery of insulin. J Biomed Mater Res A. 89:1063–71.
- De Coninck, A., Draye, J.P., Van Strubarq, A., Vanpée, E., Kaufman, L., Delaey, B., Verbeken, G., Roseeuw, D. (1996). Healing of full-thickness wounds in pigs: effects of occlusive and non-occlusive dressings associated with a gel vehicle. J Dermatol Sci. 13:202–11.
- Desbois, A.P., Gemmell, C.G., Coote, P.J. (2010). In vivo efficacy of the antimicrobial peptide ranalexin in combination with the endopeptidase lysostaphin against wound and systemic methicillin-resistant Staphylococcus aureus (MRSA) infections. Int J Antimicrob Agents. 35:559–65.
- Jeong, S.I., Kwon, J.H., Lim, J.I., Cho, S.W., Jung, Y., Sung, W.J., Kim, S.H., Kim, Y.H., Lee, Y.M., Kim, B.S., Choi, C.Y., Kim, S.J. (2005). Mechano-active tissue engineering of vascular smooth muscle using pulsatile perfusion bioreactors and elastic PLCL scaffolds. Biomaterials. 26:1405–11.
- Kokai-Kun, J.F., Walsh, S.M., Chanturiya, T., Mond, J.J. (2003). Lysostaphin cream eradicates Staphylococcus aureus nasal colonization in a cotton rat model. Antimicrob Agents Chemother. 47:1589–97.
- Liu, B.S., Yao, C.H., Fang, S.S. (2008). Evaluation of a non-woven fabric coated with a chitosan Bi-layer composite for wound dressing. Macromol Biosci. 8:432–40.
- Liu, T.Y., Chen, S.Y., Lin, Y.L. (2006). Synthesis and characterization of amphiphatic carboxymethyl-hexanoyl chitosan hydrogel: water-retention ability and drug encapsulation. Langmuir. 22:9740–5.
- Ma, P.X. (2008). Biomimetic materials for tissue engineering. Adv Drug Deliv Rev. 60:184–98.
- Mourtzinos, I., Konteles, S., Kalogeropoulos, N., Karathanos, V.T. (2009). Thermal oxidation of vanillin affects its antioxidant and antimicrobial properties. Food Chem. 114:791–7.
- Salem, H., Ciba, P., Rapoport, D.H., Egana, J.T., Reithmayer, K., Kadry, M., Machens, H.G., Kruse, C. (2009). The influence of pancreas-derived stem cells on scaffold based skin regeneration. Biomaterials. 30:789–96.
- Steffansen, B., Herping, S.P.K. (2008). Novel wound models for characterizing ibuprofen release from foam dressings. Int J Pharm. 364:150–5.
- Uygur, F., Öncül, O., Evinç, R., Diktas, H., Acar, A., Ülkür, E. (2009). Effects of three different topical antibacterial dressings on Acinetobacter baumannii-contaminated full-thickness burns in rats. Burns. 35:270–3.
- Weng, L.H., Romanov, A., Rooney, J., Chen, W. (2008). Non-cytotoxic, in situ gelable hydrogels composed of N-carboxyethyl chitosan and oxidized dextran. Biomaterials. 29:3905–13.
- Wittaya-Areekul, S., Prahsarn, C. (2006). Development and in vitro evaluation of chitosan-polysaccharides composite wound dressings. Int J Pharm. 313:123–8.
- Yates, C.C., Whaley, D., Babu, R., Zhang, J., Krishna, P., Beckman, E., Pasculle, A.W., Wells, A. (2007). The effect of multifunctional polymer-based gels on wound healing in full thickness bacteria-contaminated mouse skin wound models. Biomaterials. 28:3977–86.
- Zhou, R.Q., Chen, S.G., Recsei, P. (1988). A dye release assay for determination of lysostaphin activity. Anal Biochem. 171:141–4.