Abstract
Topical application of plasmid DNA represents an attractive route of gene delivery. Although chitosan (CS) has been widely investigated as a gene-carrier, there is very limited information about the skin application of CS-based systems for DNA. This study evaluated pDNA-loaded chitosan nanoparticles (CS-NPs) for skin gene delivery. NPs were prepared by inducing the gelation of CS upon interaction with sodium tripolyphosphate. pSV-β-Gal was used as a reporter gene. The size, surface charge, and the other in vitro characteristics of CS-NPs were examined. Primary human dermal fibroblast cells (HDF) and mouse fibroblast NIH 3T3 cell lines (ATCC CCL-92) were used for in vitro transfection studies. In in vivo study, CS-NPs were applied to the skin of baby and adult Sprague Dawley rats by spreading on the shaved area of the back of animals. During a week animals were sacrificed and skin biopsies were taken for β-Gal expression. β-galactosidase enzyme activity was determined spectrophotometrically at 420 nm. The distribution of β-galactosidase expressing cells within the skin tissue was observed by X-gal histochemical method. β-galactosidase was continuously expressed at the nanoparticle-treated skin during the 7 days. High and continuous β-Gal expressions were obtained with CS-NPs, although it was low in the first day. When a comparison was made between the data of baby and adult rats, markedly high transfection were measured in the skin samples of the baby rats. NPs protected pDNA against the enzyme and serum attacks. In conclusion, CS-NPs showed in vivo transfection potential in rats for skin gene delivery.
Introduction
The skin is an attractive organ for the development and clinical administration of both therapeutic and prophylactic gene medicines. Recently skin gene therapy has been extensively studied at the cellular and molecular levels, allowing increased gene expression (CitationHengge et al., 1996; Citation1998).
Non-viral skin gene therapy is an effective method to deliver and transiently express genes in the skin (CitationVogel, 2000). Non-viral therapies for skin diseases require an increased level and duration of gene expression in skin cells. Although various approaches have been developed to deliver pDNA to the skin, most studies have focused on ex vivo approaches.
Liposomes-DNA complexes and free DNA were used for the topical gene delivery (CitationAlexander & Akhurst, 1995; CitationFan et al., 1999; CitationDomashenko et al., 2000). CitationCui and Mumper (2002) reported limited success of delivery of DNA vaccines to epidermal dendritic cells (DC) by administering the nanoparticle-based DNA delivery system using a needle-free jet injection device. Genetic vaccine targeted to DC. CitationYu et al. (1999) topically applied naked plasmid DNA onto mouse skin. CitationFan et al. (1999) topically applied both naked and liposome entrapped plasmid encoding HBsAg for cellular immune response. CitationLi and Hoffman (1995) detected Lac Z gene protein in hair follicles after application of DNA:liposome complex onto mouse skin. CitationMeykadeh et al. (2005) applied topical spray form of pEGFP-N1 DNA-liposome to the skin for vaccination.
Among non-viral gene delivery systems, chitosan is a biodegradable, biocompatible natural polysaccharide that has shown potential for gene delivery (CitationKoping-Hoggard et al., 2001; Citation2003; Citation2004; CitationGan et al., 2005; CitationIssa et al., 2006). Cationic chitosan can effectively bind (CitationCui & Mumper, 2001; CitationMansouri et al., 2004) or encapsulate DNA (CitationAral et al., 2000; CitationAkbuğa et al., 2003; Citation2004; CitationÖzbaş-Turan et al., 2003), and protect it from nuclease degradation. However, most of these previous experiments were limited by low gene transfection efficiency. Although chitosan has been studied as a potential gene delivery carrier, there is limited information on skin gene delivery application of chitosan (CitationCui & Mumper, 2001; Citation2002; CitationJayakumar et al., 2010a; Citationb).
We previously studied different chitosan-based dosage forms such as hydrogel and chitosan-coated liposomes as a skin gene delivery system (CitationAkbuğa & Özbaş-Turan, 2006).
The aims of this study are to evaluate chitosan nanoparticles for a skin gene delivery system and to investigate in vitro and in vivo skin gene transfer transfection efficiency of DNA loaded-chitosan nanoparticles. pSV-β-Gal was used as a reporter gene.
Methods
Chemicals
Chitosan (Medium; mol. wt: ca. 400kDa, viscosity: ca. 200 mPa in 1.0% acetic acid at 20°C) with a deacetylation grade of ~ 87% were purchased from Fluka (Steinheim, Switzerland). Cell culture media, fetal bovine serum, and cell culture supplements were purchased from Gibco-BRL (Grand Island, N.Y., USA). Tripolyphosphate (TPP), o-nitrophenyl-β-D-galactopyranoside (ONPG), and 5-bromo-4-chloro-3-indolyl-beta-D-galactopyranoside (X-Gal) were purchased from Sigma (St. Louis, USA). All other chemicals were of cell culture and molecular biology grade.
Plasmid
The plasmid DNA, pSV-β-Gal (Promega, Madison, WI) containing β-galactosidase gene under the control of SV40 promoter was 6820 bp.
Preparation of CS-NPs
CS-NPs were prepared according to Fernandez-Urrusano et al. (1999) and CitationCalvo et al. (1997). Aqueous acidic solutions of CS (~ pH 4.35, 1% acetic acid) at different concentrations (0.25–0.5%, w/v, 50 ml) were prepared. TPP solution (0.125–0.5%, w/v, 50 ml) containing pDNA (250, 500, and 1000 μg) was dropped into the aqueous acidic solution of CS in the equal volume while mixing at 900–1000 rpm/min at room temperature (RT). The number of drops was adjusted to 10 drops/min. The NP suspension was gently stirred for 30 min at RT for gelation. Formed NPs were separated by centrifugation at 16.000 xg at 20 min and washed three times with bi-distilled water. Freeze-dried (Leybold-Lyovac, Cologne, Germany) NPs were stored at 4°C until use. The supernatant was spectrophotometrically analyzed at 260 and 280 nm for DNA content (CitationMao et al., 2001; CitationAkbuğa & Özbaş-Turan, 2006). Encapsulation efficiency was calculated by measuring the difference between the total amount of DNA added in the preparation medium and the amount of non-entrapped DNA remaining in the aqueous supernatant after processing. Different parameters such as chitosan and TPP concentrations, DNA amount and formulation codes are given in .
Table 1. Composition of CS-NPs and their particle size, encapsulation efficiency, and zeta potential (n = 3).
Characterization of CS-NPs
Size and zeta potential (surface charge) of NPs were measured at least in triplicate using a Malvern 3000 HSA (Malvern, Worcestershire, UK). For both of the above measurements, NPs were suspended in 10 mM acetate buffer at pH 5.5 and in phosphate buffered saline (PBS) at pH 7.4.
The morphological examination of NPs were made by Scanning Electron Microscopy (SEM, Jeol, JSM 5200, Tokyo, Japan).
DNase I and serum stability of CS-NPs
Stability of CS-NPs formulations against DNase I and serum was analyzed. For DNase I stability study, NPs containing 10 µg pDNA were reacted with 10 μl DNase I (10 U, 1 U/μg). Reaction was carried out at 37°C and aliquots were taken at 48 h. For the inhibition of reaction, 0.5 M EDTA was used. DNA degradation was analyzed with agarose gel electrophoresis.
Stability of CS-NPs formulations against serum was studied by incubating the samples in 10% fetal calf serum at 37°C. Samples were taken at 48 h and analyzed with agarose gel electrophoresis.
In vitro release studies
Plasmid DNA release was determined by incubating the NPs (20 μg pDNA) in PBS at 37°C ± 0.5°C. At appropriate time intervals, samples were centrifuged and the supernatant was replaced with fresh medium, and the amount of DNA released in the supernatant was measured spectrophotometrically (Biospec UV 1601-Shimadzu, Kyoto, Japan) at 260 nm.
In vitro transfection studies
Two different cell lines (NIH 3T3 and HDF) were used. All cell lines were cultured in DMEM supplemented with 10% FBS, L-glutamine, and antibiotic solution, and incubated at 37°C and at 5.0% CO2. Cells were seeded in 24-well plates (5.0 × 104 cells/well) and grown in standard culture conditions for 24 h. CS-NPs (0.1 µg pDNA for each well) were added to in 24-well plates and incubated for 1–6 days post-transfection. The cells were washed twice with ice-cold PBS and lysed in 100 µl of lysis buffer by freezing and thawing. The β-galactosidase assay was performed in a microtiter dish using ONPG as substrate solution. The absorbance of samples was measured spectrophotometrically at 420 nm. Purified recombinant β-Gal was used as standard (CitationAral et al., 2000; CitationAkbuğa et al., 2003; CitationÖzbaş-Turan et al., 2003).
In vivo studies
The animal experiments were approved by The Animal Ethical Committee of Marmara University. One week-old baby (18–20 g) and 7 weeks-old adult (175–200 g) Sprague-Dawley rats were used.
Rats were kept in individual plastic cages in a 12:12 light–dark cycle (light-on period, 6:00 am–6:00 pm) in a controlled temperature chamber on sawdust bedding. They were fed a standard diet and had access to tap water. Body weight was recorded at regular intervals.
The hairs on the back of adults were shaved. Animals were anesthetized with atropine and ketamin (0.05 mg/kg, 100 mg/kg) and the back area of rats was swabbed with povidone before alcohol application. CS-NPs containing 100 µg pDNA in 250 µl PBS were topically applied to an area of 2 cm2 on the back of rats. PBS and empty NPs were used as control. The animals were sacrified 1, 2, 3, 4, 5, 6, and 7 days after pDNA treatment to evaluate gene expression. Full thickness skin samples were taken and β-galactosidase expression was measured as mentioned below.
β-galactosidase assay
Full thickness skin samples were homogenized after lysis solution addition and centrifuged at 12,000 xg at 15 min. β-gal activity was determined using ONPG according to the guidance leaflet of of Promega (2006). The ONPG solution was added to cell lysate and incubated at 37°C. The activity was assayed spectrophotometrically at 420 nm. Total protein contents of skin samples were assayed according to Bradford’s (1976) method.
Histological studies
Skin samples were histologically examined after X-Gal staining by a light microscope (Olympus BX-20, Tokyo, Japan) (CitationBancroft & Gamble, 2003). Skin sections were cut at 40 μm on a cryostat and washed in 0.1 M phosphate-buffered saline (PBS) for 10 min twice. Sections were stained in X-Gal reaction buffer (0.8% NaCl, 8 mM Na2HPO4, 2.7 mM KCl, 1.5 mM KH2PO4, 2 mM MgCl2, 35 mM K4[Fe(CN)6].3H2O, 35 mM K3[Fe(CN)6], 0.02% Nonidet P-40, 0.01% deoxycholic acid) containing 1 mg/ml of X-gal to visualize β-gal activity. The reaction was performed for 20 h at 37°C in darkness. Subsequent to X-Gal staining, sections were washed in 0.1 M phosphate buffer for 10 min twice. Sections were mounted on gelatin-coated slides and cover slipped.
Statistics
All data were compared using Mann Whitney Rank Sum Test and Student’s t-test. A probability (p < 0.05) was considered significant.
Results
Characterization study
In this study we investigated whether simple CS-NPs could be used to deliver DNA to skin cells after topical application and also we studied the properties of in vitro and in vivo transfection efficiencies of CS-NPs. CS in different concentrations was used for the formulation of NPs. The size of NPs ranged from 190.51 ± 19.05 to 287.25 ± 14.12 nm (). Formulation variables such as DNA amount, CS, and TPP concentrations affected the particle size of NPs (p < 0.05). SEM photograph of CS-NPs are given in . This data shows similarity with the earlier reports (CitationCalvo et al., 1997; Fernandez-Urrusuno et al., 1990). By use of the ionotropic gelation method, cationic NPs were obtained. Zeta potential values of CS-NPs changed pH 5.5 between 17.09 ± 0.71 and 41.45 ± 0.43 mV and pH 7.4 between 10.91 ± 0.98 and 32.05 ± 0.39mV (). As expected, zeta potential values decreased as the amount of DNA increased. High DNA encapsulation was obtained, as seen in . The DNA encapsulation ranged from 88.79 ± 0.64 to 91.98 ± 0.40%.
pDNA release from NPs was observed during the 60 days that pDNA release was completed (–). Burst DNA release was not seen in the first day; 10–20% of pDNA was released from NPs within the first 10 days. pDNA remained intact during the release studies (). The concentration of released DNA changed with the formulation variables examined. On the other hand, serum and enzyme stabilities of encapsulated pDNA were investigated and DNA degradation was not observed during this study (). Stability results showed similarity with the previous studies (CitationAral et al., 2000; CitationAkbuğa et al., 2003; CitationÖzbaş-Turan et al., 2003).
In vitro transfection studies
NIH 3T3 cells and HDF were used in in vitro transfection studies. CS-NPs prepared with different CS concentrations (0.25, 0.375, and 0.5%) were applied to cell lines and expression was observed during 6 days (). In two cell lines, β-gal expression peaked on the 6th day after the transfection in both cell types (p < 0.05). Expression level of β-gal changed with CS concentration used in the NP formulations. Highest expressions were determined when the CS was used in low concentration.
Similar β-gal expression profiles were obtained in HDF (); however, lower β-gal production was measured in HDF than 3T3 cell lines (p < 0.05).
Animal studies
Following the preliminary in vitro studies, the best formulation (L2) was selected and used in animal studies CS-NPs were topically applied to the back of rats. Adult and baby rats were used in experiments, and the results were compared.
As seen in , topical treatment with CS-NPs resulted in significantly higher levels of β-gal expression compared to the control (day 3) (p < 0.05).
Figure 7. In vivo expression of β-galactosidase in Sprague Dawley rats transfected with CS-NPs (n = 5).
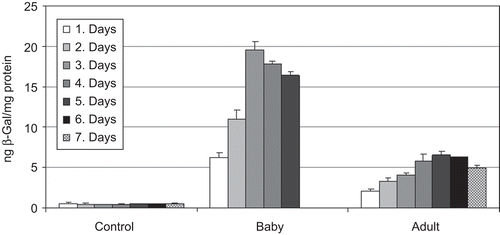
After 24 h of NPs application, β-gal gene expression was measured in the skin samples of baby and adult rats. During the study, β-gal expression had continued. In baby samples, rapid β-gal expression was measured after the 2nd day and a peak was observed at the 3rd day (). Higher β-gal expression was measured in the baby rats than adults (p < 0.05). Four-fold increases in transgene expression was determined in baby rats. On the other hand, during the topical treatment, skin irritation and redness in the application area were not observed.
Skin specimens were histologically investigated after X-gal staining. As an indicator of DNA expression, blue color was observed with X-Gal staining according to CitationBancroft and Gamble’s (2003) method. No staining was observed in control cells after X-gal staining of skin specimens ().
However, blue color stained cells were seen in CS-NP applied specimens. β-gal expression was mainly localized in the dermis and hypodermis, especially, staining cells were determined close to the hair follicles.
Discussion
Recently skin has been investigated for pDNA delivery as an alternative to i.m. administration of pDNA; however, the commercial availability of gun technology or the needle-free devices is not clear (CitationCui & Mumper, 2001). pDNA-CS nanocomplexes, pDNA-coated CS-CMC NPs were used for topical genetic immunization (CitationCui & Mumper, 2001). Reports are limited to immunization efficiency of cutaneous application of NPs. However, a detailed study is not present in the literature on CS-NPs.
In this study, we investigated whether simple CS-NPs could be used to enhance pDNA transfection after topical application. CS-NPs were characterized and their potential in skin gene therapy was evaluated. All the CS-NPs had positive surface charge and were smaller than 300 nm. In this context, the effect of different formulation parameters such as CS, TPP concentration, and initial plasmid amount on release properties of DNA-loaded CS-NPs was investigated. Three levels of CS concentrations and DNA amount, and four levels of TPP concentration were studied. DNA release from CS-NPs increased proportionally with chitosan () and TPP () concentrations, but release conversely changed with DNA concentration. As suggested by CitationCsaba et al. (2009), pDNA irreversibly bound to CS and released from the structure. On the other hand, TPP concentration and CS/TPP ratio are important factors influencing the properties of the CS-TPP NPs. As seen in , DNA release from NPs increased when the TPP concentration is higher. When the concentration of TPP was low and CS/TPP ratio was high, TPP might have cross-linked dominantly inter- and intra-molecularly with CS and formed NPs, and DNA release from this structure may be slow. In contrast, when the TPP concentration increased, the structure of NP may release DNA more rapidly. In vitro transfection studies, in two different cell lines (NIH 3T3 and human dermal fibroblast), have revealed that CS-NPs are suitable delivery systems for DNA, and CS concentration used in this experiment is an effective parameter.
In animal studies we tested and compared CS-NPs in baby and adult rats because of the differences. In baby rats, the hair follicles are virtually in the anagen phase so that the size of the anagen follicles is enlarged and the cells are growing in a synchronous manner (CitationRaghavachari & Fahl, 2002). Therefore, we tested our NPs in adult and baby rats and, as shown in and , higher expression was measured in the baby rats than adults. Also, similarity between histological and enzymatic results was obtained. CitationHofmann et al. (1996) noted that early anagen follicular cells were the primary cell population that was transformed during skin gene transfer. Our data confirmed this earlier report.
Contradictory results are present concerning the charge of skin applied particles (CitationShi et al., 1999; CitationCui & Mumper, 2001; CitationKohli & Alpar, 2004). CitationCui and Mumper (2001) reported that negative charged NPs resulted higher gene expression and immune responses; however the reason for these results is not yet understood. Similar results were noted by CitationKohli and Alpar (2004). The studies of CitationShi et al. (1999) and CitationFan et al. (1999) demonstrated the feasibility of topical genetic immunization with cationic particles. However, in this study, high β-gal expression was measured after skin application of CS-NPs having positive surface charge. Our result exhibits similarities with CitationShi et al. (1999) and CitationBadea et al. (2007) data. CitationBadea et al. (2007) evaluated cationic NPs as a topical formulation for interferon gamma (IFN-γ) gene in an IFN-γ deficient mouse model and obtained increased gene expression in the skin. They reported that higher degree of DNA compaction might contribute to better interaction of nanoparticles with the skin and better protection of genetic material.
According to histological studies, β-gal expression was mainly localized in dermis and hypodermis particularly close to the hair follicles. The stratum corneum (sc) acts as the major barrier to penetration of large molecules across the skin. Large, negatively-charged, DNA do not easily penetration the sc, as they have low lipid solubility (CitationWraight & White, 2001). However, we found extensive β-gal expression into dermis; on the other hand CitationElias et al. (1981) noted that epidermis offers much lower resistance to polar drugs than sc. CitationLauer et al. (1997) suggest that hair follicles have fewer penetration of polar compound. Our results show similarity with previous works which have demonstrated that topically-applied DNA can access cellular targets via the hair follicles.
Moreover, whether intercellular or transcellular routes of transportation play a role has not been solved, as reported by CitationMeykadeh et al. (2005). CitationBrand and Iversen (2000) reported that if oligonucleotides pass through the keratinocytes or viable cells of the skin then systemic delivery should be considered. Therefore, our results may be important for systemic DNA delivery also.
Conclusions
TPP-chitosan nanoparticles appeared to be an effective and suitable pDNA delivery system for topical gene delivery. High and sustained gene expression can be obtained with skin application of CS-NPs
Declaration of interest
The authors state no conflicts of interest and have received no funding for the research or in the preparation of this manuscript.
References
- Akbuğa, J., Aral, C., Özbaş-Turan, S., Kabasakal, L., Keyer-Uysal, M. (2003). Transfection efficiency of chitosan microspheres effect of DNA topology. STP Pharm Sci. 13:99–103.
- Akbuğa J, Özbaş-Turan S. (2006). Comparison of chitosan-based systems for skin gene delivery. XIVth Annual Congress of The European Society for Gene Therapy, Athens, Greece, 9–12 November.
- Akbuğa, J., Özbaş-Turan, S., Erdoğan, N. (2004). Plasmid-DNA loaded chitosan microspheres for in vitro IL-2 expression. Eur J Pharm Biopharm. 58:501–7.
- Alexander, M.Y., Akhurst, R.J. (1995). Liposome-mediated gene transfer and expression via the skin. Hum Mol Genet. 4:2279–85.
- Aral, C., Özbaş-Turan, S., Kabasakal, L., Keyer-Uysal, M., Akbuğa, J. (2000). Studies of effective factors of plasmid DNA-loaded chitosan microspheres: I. Plasmid size, chitosan concentration and plasmid addition technique. STP Pharm Sci. 10:83–8.
- Badea, I., Wettig, S., Verrall, R., Foldvari, M. (2007). Topical non-invasive gene delivery using gemini nanoparticles in interferon γ deficient. EJPB. 65:414–22.
- Bancroft, J.D., Gamble, M. (2003). Theory and practice of histological techniques. 5th ed. Edinburgh, London: Churchill Livingstone.
- Bradford, M.M. (1976). A rapid and sensitive method for the quantitation of microgram quantities of protein utilizing the principle of protein-dye binding. Anal Biochem. 72:248–54.
- Brand, R.M., Iversen, P.L. (2000). Transdermal delivery of antisense compounds. Adv Drug Del Rev. 44:51–7.
- Calvo, P., Remunan-Lopez, C., Vila Jato, J.L., Alonso, M.J. (1997). Chitosan and chitosan/ethylene oxide-propylene oxide block copolymer nanoparticles as novel carriers for proteins and vaccines. Pharm Res. 14:1431–6.
- Csaba, N., Köping-Höggård, M., Alonso, M.J. (2009). Ionically crosslinked chitosan/tripolyphosphate nanoparticles for oligonucleotide and plasmid DNA delivery. Int J Pharm. 382:205–14.
- Cui, Z., Mumper, R.J. (2001). Chitosan-based nanoparticles for topical genetic immunization. J Contr Rel. 75:409–19.
- Cui, Z., Mumper, R.J. (2002). Topical immunization using nanoengineered genetic vaccines. J Contr Rel. 81:173–84.
- Domashenko, A., Gupta, S., Cotsarelis, G. (2000). Efficient delivery of transgenes to human hair follicle progenitor cells using topical lipoplex. Nat Biotechnol. 18:420–3.
- Elias, P.M., Cooper, E.R., Korc, A., Brown, B.E. (1981). Percutaneous transport in relation to stratum corneum structure and lipid composition. J Invest Dermatol. 76:297–301.
- Fan, H., Lin, O., Morrissev, G.R., Khavari, P.A. (1999). Immunization via hair follicles by topical application of naked DNA to normal skin. Nat Biotechnol. 17:870–2.
- Fernandez-Urrusuno, R., Calvo, P., Remunan-Lopez, C., Vila Jato, J.L. (1999). Chitosan nanoparticles: a new vehicle for increasing the nasal absorption of peptides. Proceed Intern Symp Control Rel Bioact Mater. 26:353–4.
- Gan, Q., Wang, T., Cochrane, C., McCarron, P. (2005). Modulation of surface charge, particle size and morphological properties of chitosan-TPP nanoparticles intended for gene delivery. Colloid Surfaces B. 44:65–73.
- Hengge, U.R., Pfützner, W., Williams, M., Goos, M., Vogel, J.C. (1998). Efficient expression of naked plasmid DNA in mucosal epithelium: Prospective for the treatment of skin lesions. J Invest Dermatol. 111:605–8.
- Hengge, U.R., Walker, P.S., Vogel, J.C. (1996). Expression of naked DNA in human, pig, and mouse skin. J Clin Invest. 97:2911–6.
- Hofmann, U., Tokura, Y., Nishijima, T., Takigawa, M., Paus, R. (1996). Hair cycle-dependent changes in skin immune functions: anagen-associated depression of sensitization for contact hypersensitivity in mice. J Invest Dermatol. 106:598–604.
- Issa, M.M., Koping-Hoggard, M., Tommeraas, K., Varum, K.M., Christensen, B.E., Strand, S.P., Artursson, P. (2006). Targeted gene delivery with trisaccharide-substituted chitosan oligomers in vitro and after lung administration in vivo. J Contr Rel. 28:103–12.
- Jayakumar, R., Chennazhi, K.P., Muzzarelli, R.A.A., Tamura, H., Nair, S.V., Selvamurugan, N. (2010a). Chitosan conjugated DNA nanoparticles in gene therapy. Carbohydr Polym. 79:1–8.
- Jayakumar, R., Menon, D., Manzoor, K., Nair, S.V., Tamura, H. (2010b). Biomedical applications of chitin and chitosan based nanomaterials-A short review. Carbohydr Polym. 82:227–32.
- Kohli, A.K., Alpar, H.O. (2004). Potential use of nanoparticles for transcutaneous vaccine delivery: effect of particle size and charge. Int J Pharmceut. 275:13–7.
- Koping-Hoggard, M., Mel’nikova, Y.S., Varum, K.M., Lindman, B., Artursson, P. (2003). Relationship between the physical shape and the efficiency of oligomeric chitosan as a gene delivery system in vitro and in vivo. J Gene Med. 5:130–41.
- Koping-Hoggard, M., Tubulekas, I., Guan, H., Edwards, K., Nilsson, M., Varum, K.M., Artursson, P. (2001). Chitosan as a nonviral gene delivery system. Structure-property relationship and characteristics compared with polyethyleneimine in vitro and after lung administration in vivo. Gene Ther. 8:1108–21.
- Koping-Hoggard, M., Varum, K.M., Issa, M.M., Danielsen, S., Christensen, B.E., Stokke, B.T., Artursson, P. (2004). Improved chitosan-mediated gene delivery based on easily dissociated chitosan polyplexes of highly defined chitosan oligomers. Gene Ther. 11:1441–52.
- Lauer, A.C., Elder, J.T., Weiner, N.D. (1997). Evaluation of the hairless rat as a model for in vivo percutaneous absorbtion. J Pharm Sci. 86:13–8.
- Li, L., Hoffman, R.M. (1995). The feasibility of targeted selective gene therapy of the hair follicle. Nat Med. 1:705–6.
- Mansouri, S., Lavigne, P., Corsi, K., Benderdour, M., Beaumont, E., Fernandes, J. (2004). Chitosan-DNA nanoparticles as non-viral vectors in gene therapy: strategies to improve transfection efficacy. Eur J Pharm Biopharm. 57:1–8.
- Mao, H.Q., Roy, K., Troung-Le, V.L., Janes, K.A., Lin, K.Y., Wang, Y., August, J.T., Leong, K.W. (2001). Chitosan-DNA nanoparticles as gene carriers: synthesis, characterization and transfection efficiency. J Contr Rel. 70:399–421.
- Meykadeh, N., Mirmohammadsadegh, A., Wang, Z., Basner-Tschakarian, E., Hengge, U.R. (2005). Topical application of plasmid DNA to mouse and human skin. J Mol Med. 83:897–903.
- Özbaş-Turan, S., Aral, C., Kabasakal, L., Keyer-Uysal, M., Akbuğa, J. (2003). Co-encapsulation of two plasmids in chitosan microspheres as a non-viral gene delivery. J Pharm Pharmaceut Sci. 6:27–32.
- Promega Corporation. Technical Bulletin. (2006). ß-Galactosidase enzyme assay system with reporter lysis buffer. Parts#TB097. 2800 Woods Hollow Road, Madison, WI 53711-5399, USA.
- Raghavachari, N., Fahl, W.E. (2002). Targeted gene delivery to skin cells in vivo: a comparative study of liposomes and polymers as delivery vehicles. J Pharm Sci. 91:615–22.
- Shi, Z., Curiel, D.T., Tang, D.C. (1999). DNA-based non-invasive vaccination onto the skin. Vaccine. 17:2136–41.
- Vogel, J.C. (2000). Nonviral skin gene therapy. Human Gene Therapy. 11:2253–9.
- Wraight, C.J., White, P.J. (2001). Antisense oligonucleotides in cutaneous therapy. Pharmacol Therapeut. 90:89–104.
- Yu, W.H., Kashani-Sabet, M., Liggitt, D., Moore, D., Heath, T.D., Debs, R.J. (1999). Topical gene delivery to murine skin. J Invest Dermatol. 112:370–5.