Abstract
To develop a thermo-sensitive injectable hydrogel that is easy to administer, gels quickly in the body, and allows sustained release of the drug, various poloxamer-based hydrogels containing doxorubicin were prepared with poloxamer and hydrochloric acid under light protection using the cold method. Their rheological characterization, dissolution, and pharmacokinetics after intramuscular administration to rats were evaluated. Hydrochloric acid decreased the viscosity and retarded the gelation time of the injectable gel. The drug was dissolved from the hydrogels by Fickian diffusion through the extramicellar aqueous channels of the gel matrix. P 188 and hydrochloric acid barely affected the dissolution mechanism. However, P 188 increased and hydrochloric acid decreased the dissolution rate of the drug from the injectable gels. The thermo-sensitive injectable gel composed of 0.6% doxorubicin, 15% P 407, 6% P 188, and 0.1% hydrochloric acid was easy to administer intramuscularly and gelled quickly in the body. Moreover, it maintained the plasma concentrations of drug for 60 h and gave an ~ 5-fold higher AUC compared to doxorubicin solution. Thus, it would be useful for delivering doxorubicin in a pattern that allows sustained release for a long time, leading to better bioavailability.
Introduction
Doxorubicin is an anti-neoplastic agent used in the treatment of acute leukemia, lymphomas, and some solid tumors such as breast, ovarian, and endometrial cancers (CitationMinotti et al., 2004; CitationTan et al., 2005). However, clinical utility is hampered by cumulative, dose-limiting cardiotoxicity, myelosuppression, and drug resistance (CitationKlein-Szanto, 1992; CitationArnold et al., 2004). Thermo-sensitive hydrogels that undergo a sol-to-gel transition in response to temperature changes are of great interest in long-acting therapeutic delivery and tissue engineering as an injectable depot system (CitationKang et al., 2006b; CitationJeong & Gutowska, 2002). Poloxamer, a copolymer of poly(oxyethylene)-poly(oxypropylene)-poly(oxyethylene), has been studied as a potential base for thermo-sensitive hydrogels (CitationCabana et al., 1997). It can carry sufficient drug, and shows good water-solubility, tolerability, biodegradability, non-toxicity, and controlled release (CitationVeyries et al., 1999). Furthermore, poloxamer solutions are known to exhibit the phenomenon of reverse thermal gelation, remaining as solutions at low temperatures and gelling following an increase in temperature (CitationLenarets et al., 1987; CitationChoi et al., 1998a). There have been several attempts to modulate the gelation temperature of poloxamer-based liquids. The gelation temperature of poloxamer solutions can be adjusted by modifying cross-linking agents and monomers, mixing different series of poloxamers, changing the weight of the poloxamers and changing the pH and the ionic strength (CitationSchmolka, 1972; CitationChoi et al., 1998a). However, for practical use of pharmaceutical preparations, the gelation temperature of poloxamer solution had been controlled by mixing poloxamer series and adding the appropriate ingredients (CitationChoi et al., 1998a; Citation1999). Such thermo-sensitive hydrogels have been used as a controlled release drug delivery system for injectable (CitationBhattarai et al., 2005; CitationPaavola et al., 2000; CitationRicci et al., 2002; CitationLee & Tae, 2007), ocular (CitationRaymond et al., 2004), and rectal administration (CitationChoi et al., 1998b; CitationYun et al., 1999; CitationYong et al., 2004). However, most previous studies have focused only on rheological theory, and the in vitro and in vivo sustained release action of poloxamer-based thermo-sensitive hydrogels. There is a lack of knowledge on practical administration to the body such as syringe-ability and gelation time, although these two factors are crucial in the design of a desirable thermo-sensitive hydrogel that is easy to administer to the body and gels rapidly, enabling practical use in pharmaceutical preparations.
In this study, to develop a novel temperature-sensitive injectable hydrogel containing doxorubicin, the hydrogels were prepared with poloxamer and hydrochloric acid using the cold method. Their rheological characterization, dissolution, and pharmacokinetics after intramuscular administration to rats were evaluated. Since doxorubicin was poorly soluble but its hydrochloride salt form was practically soluble in water, hydrochloric acid was used as a solubilizer of doxorubicin in this study (CitationKang et al., 2006a; CitationKakinoki et al., 2007).
Materials and methods
Materials
Doxorubicin was supplied by Dong-A chemical (Suwon, South Korea). Poloxamer 407 (P 407) and poloxamer (P 188) were obtained from BASF (Ludwigshafen, Germany). Semi-permeable membrane tubing (Spectra membrane tubing No.1) was purchased from Spectrum Medical Industries Inc. (Los Angeles, CA). Hydrochloric acid was of USP grade. All other chemicals were of reagent grade and were used without further purification.
Animals
Male Sprague-Dawley rats weighing 250 ± 20 g were fasted for 12 h prior to the experiments, but allowed free access to water. Twelve rats were divided into two groups. Each rat was intramuscularly administered with doxorubicin solution or injectable hydrogel, respectively. All animal care and procedures were conducted according to the Guiding Principles in the Use of Animals in Toxicology, as adopted in 1989, revised in 1999, and amended in 2008 by the Society of Toxicology (CitationSOT, 2008). Furthermore, the protocols for the animal studies were approved by the Institute of Laboratory Animal Resources of Yeungnam University.
Preparation of doxorubicin-loaded injectable hydrogels
The poloxamer-based injectable gels were prepared with various ratios of doxorubicin, P 407, P 188, and hydrochloric acid under light protection using the cold method (CitationChoi et al., 1998a; CitationYong et al., 2004). The detailed compositions of doxorubicin-loaded injectable gels are given in . P 188 and P 407 were dissolved in distilled water at 4°C with gentle stirring. The poloxamer solution was left overnight in a refrigerator until a clear solution was formed. Various amounts of hydrochloric acid and doxorubicin were subsequently added to the poloxamer solution with gentle stirring, and then kept overnight at 4°C.
Table 1. Composition and gelation time of doxorubicin-loaded injectable hydrogel.
Reological properties of doxorubicin-loaded injectable hydrogel
The rheological behavior of the doxorubicin-loaded injectable hydrogels was investigated at 25°C and 36.5°C using a rheometer (Physica MCR; Anton Paar, Ashland, VA, USA) (CitationRicci et al., 2002). Temperature was controlled by a circulating water bath and (TC10, Germany) and the UDS 200 program was used to control and perform calculations in the rheometer. The instrument was set up with a parallel plate geometry using 25-mm diameter plates at two temperatures and samples of ~ 1 mm in thickness.
Dissolution
Each poloxamer-based hydrogel containing 60 mg doxorubicin was inserted into a semi-permeable membrane tube. Both sides of the tube were tied with a thread to prevent leakage. The semi-permeable membrane tube was then placed in a dissolution tester (DST-600; Fine Chemical, Hwasung, South Korea). The dissolution test was performed at 36.5°C using the paddle method at 100 rpm with 60 ml distilled water as a dissolution medium. At pre-determined intervals, 0.1 ml of the medium was sampled and filtered (CitationFan & Dash, 2001; CitationYong et al., 2004). The resulting solution was then analyzed by HPLC as described below.
Animal study
The doxorubicin solution or injectable hydrogel (0.3 ml/kg; equivalent to 10 mg doxorubicin/kg) were administered intramuscularly to the hind leg of each rat. Then, 0.3 ml of blood samples were obtained at various intervals from the left or right ear vein into heparinized glass tubes and centrifuged at 12,000 g for 10 min using a 5415C centrifuge (Eppendorf, Hauppauge, NY) (CitationÁlvarez-Cedrón et al., 1999).
Blood sample analysis
Plasma (0.1 ml) was thoroughly mixed with 0.1 ml of acetonitrile and 25 µl of acetonitrile solution containing daunomycin (100 µg/ml) as an internal standard. It was centrifuged at 12,000 g for 10 min to precipitate the proteins. The supernatant layer (0.2 ml) was evaporated under N2 (g). The residue was reconstituted in 50 µl of mobile phase. The resulting solution (20 µl) was analyzed by an HPLC system (Varian ProStar 210/215; Varian, Chicago, IL) equipped with an Inertsil ODS-3 C18 column (GL science, 0.5 µm, 15 cm × 0.46 cm i.d.; Tokyo, Japan) and a UV detector (Model L-7450). The mobile phase consisted of water, acetinitrile, and acetic acid (77:22:1, v/v/v) adjusted to pH 2.8 with 1 M hydorchloride. The eluent was monitored at 505 nm with a flow rate of 0.5 ml/min (CitationZhao & Dash, 1999; CitationFan & Dash, 2001; CitationArnold et al., 2004).
Pharmacokinetic data analysis and statistical analysis
The area under the drug concentration–time curve from zero to infinity (AUC), the mean residence time (MRT), the elimination constant (Kel), and half-life (t1/2) were calculated using a non-compartmental analysis (WinNonlin; professional edition, version 2.1; pharsight Co., Mountain View, CA). The maximum plasma concentration of drug (Cmax) and the time taken to reach the maximum plasma concentration (Tmax) were obtained directly from the plasma data (CitationGibaldi & Perrier, 1982). Levels of statistical significance (p < 0.05) were assessed using the Student-t-test between two means for unpaired data. All data are expressed as mean ± standard deviation (SD) or as the median (ranges) for Tmax.
Results and discussion
The gelation temperature is the temperature at which the liquid phase makes its transition to a gel. The gelation temperature range that would be suitable for an injectable gel is 30–36°C (CitationChoi et al., 1998a; CitationYong et al., 2004). If the gelation temperature of the injectable gel is lower than 30°C, gelation occurs at room temperature, leading to difficulty in manufacturing, handling, and administration. If the gelation temperature is higher than 36°C, the injectable gel remains as a liquid at body temperature, and thus does not control the release of the drug in the body. Therefore, the injectable gel must have a suitable gelation temperature of 30–36°C to be a liquid at room temperature and to form a gel phase instantly in the body.
As potential bases of injectable gel with suitable gelation temperatures (30–36°C), poloxamer mixtures of P 407 and P 188 were selected due to their thermo-sensitive gelling properties. In addition, P 407 and P 188 are known to have low toxicity, relatively low levels of skin irritation, excellent water-solubility, high solubilizing capacity, good drug release characteristics and compatibility with other chemicals (CitationVeyries et al., 1999; CitationChoi et al., 1998a).
In the development of thermo-sensitive injectable gels, the syringe-ability is an important factor, influencing the ease of administration of the injectable gels to the body. In a preliminary study, the threshold of syringe-ability suitable for an injectable gel was investigated by administering injectable gels into the muscle of a rat using a syringe with a needle. Below the threshold of syringe-ability, it was easy to administer without separating the needle from the syringe. However, above the upper threshold, it was difficult to administer intramuscularly and the needle became separated from the syringe. In the gels containing poloxamer, the threshold in syringe-ability was a viscosity of ~ 300 mPa·s at 25°C ().
Figure 1. Viscosity of injectable gel at 25°C. Each value represents the mean ± SD (n = 3). The dotted line represents the viscosity threshold for injectable gels at 25°C.
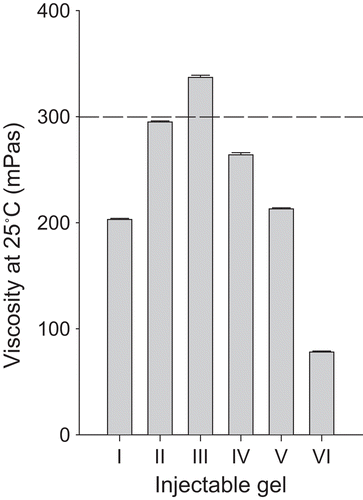
Another factor to take into consideration is the gelation time, which is the time taken for injectable gels to change from a liquid state to a gel at 36.5°C. Injectable gels with a relatively fast gelation time would show better control of the release of the drug in the body. In a preliminary study, the gelation threshold was investigated by tilting the injectable gels at 36.5°C (CitationDumortier et al., 1991; CitationRicci et al., 2002). Below the gelation threshold during tilting, the injectable gels flowed like a liquid. However, above the threshold, the injectable gels maintained the gel state and did not flow. In the gels containing poloxamer, the threshold in syringe-ability was a viscosity of ~ 4000 mPa·s at 36.5°C. Therefore, in this study, the gelation time meant the time taken for the injectable gel to reach a viscosity of ~ 4000 mPa·s in 36.5°C. The gelation time was obtained directly from the viscosity data at 36.5°C ( and ).
Figure 2. Effect of P188 on the viscosity of injectable gel at 36.5°C. The injectable gels were composed of doxorubicin, P 407, and P 188. The dotted line represents the viscosity threshold for injectable gels at 36.5°C. Each value represents the mean ± SD (n = 3).
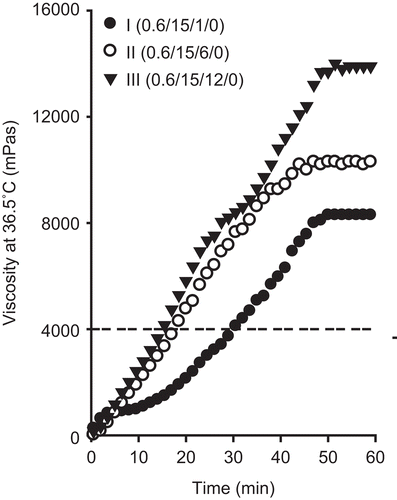
Figure 3. Effect of hydrochloric acid on the viscosity of injectable gel at 36.5°C. The injectable gels were composed of doxorubicin, P 407, P 188, and hydrochloric acid. The dotted line represents the viscosity threshold for injectable gels at 36.5°C. Each value represents the mean ± SD (n = 3).
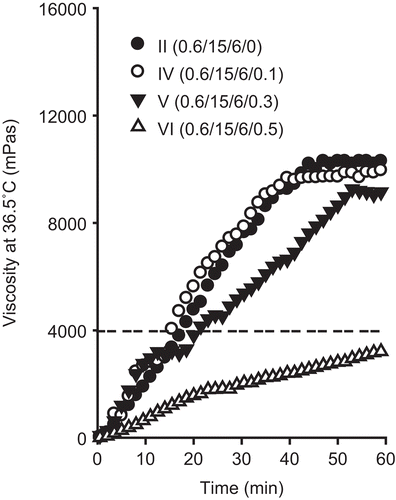
The thermo-sensitive gelation behavior of the poloxamer-based injectable gels was studied by measuring the viscosity of the samples at 25 and 36.5°C. The viscosity measurement was viewed as a quality control method in order to assess the behavior of the gels at room temperature and biological temperature.
In this study, the concentration of doxorubicin loaded in injectable gel was fixed to 0.6%. It has previously been reported that mixtures of 15% P 407 and 15–20% P 188 are a liquid at room temperature and a gel at body temperature (CitationYun et al., 1999; CitationChoi et al., 1998a). However, these poloxamer mixtures added with 0.6% doxorubicin was impossible to administer intramuscularly due to relatively very high viscosity of 800–1500 mPa·s, above the viscosity threshold of 300 mPa·s at 25°C. In practice, the needle became separated from the syringe when it was intramuscularly administered to rats. Thus, they were not suitable for formulation of doxorubicin-loaded injectable gel because they formed very viscous gels at room temperature.
The injectable gels were prepared using the cold method with 0.6% doxorubicin, 15% P 407 and 1–12% P 188, and their rheological characteristics were evaluated (; composition I–III). As the P 188 concentration in the injectable gel increased, the viscosity increased at 25°C (; composition I–III). The injectable gel containing 0.6% doxorubicin, 15% P 407, and less than 6% P 188 was easy to administer intramuscularly due to a viscosity of 200–290 mPa·s at 25°C, which is below the viscosity threshold of 300 mPa·s at 25°C. However, the injectable gel with 12% P 188 was difficult to administer intramuscularly due to a viscosity of ~ 320 mPa·s at 25°C, which is above the viscosity threshold of 300 mPa·s at 25°C. As time elapsed, the viscosity of all injectable gels gradually increased at 36.5°C, and then maintained a constant intrinsic viscosity (). The higher the P 188 concentration was in the injectable gel, the higher the constant intrinsic viscosity was. Similarly, P 188 shortened the gelation time of the injectable gels; that is, the time taken to reach a viscosity of ~ 4000 mPa·s at 36.5°C (; ; composition I–III). The injectable gels containing 0.6% doxorubicin, 15% P 407, and 6% P 188 gave the fastest gelation time of ~ 20 min (; composition II).
To investigate the effect of hydrochloric acid on the viscosity of the injectable gels, the gels were prepared with 0.6% doxorubicin, 15% P 407, 6% P 188, and 0.1–0.5% hydrochloric acid, and their rheological characteristics were evaluated (; compositions I, IV–VI). Hydrochloric acid was used as a solubilizer of doxorubicin in this study (CitationKakinoki et al., 2007). These injectable gels were clear and homogeneous, since the drug is soluble in acidic poloxamer solution. Generally, hydrochloric acid decreased the viscosity of the injectable gels at 25°C and 36.5°C ( and ; compositions II, IV–VI). Furthermore, hydrochloric acid lengthened the gelation time of the injectable gels (; ; compositions II, IV–VI). However, 0.1% hydrochloric acid affected the viscosity and gelation time of the injectable gels a little because of its very small amounts. These injectable gels were easy to administer intramuscularly due to their viscosity of 70–290 mPa·s at 25°C (), which is below the viscosity threshold of 300 mPa·s at 25°C. However, the gels containing 0.5% hydrochloric acid flowed like a liquid and did not form a gel, because their viscosity threshold was below 4000 mPa·s at 36.5°C (; composition VI).
Our results of the rheological behavior of injectable gels indicated that hydrochloric acid significantly affected the rheological characteristics of the poloxamer hydrogels. All the injectable gels tested in this study underwent an apparent sol-to-gel transition ( and ). At room temperature, the solutions were viscous liquids that flowed easily. With the exception of formulation III, these injectable gels were easy to inject intramuscularly through a 20-gauge needle, since they had a viscosity below the threshold of 300 mPa·s at 25°C. As the solutions were heated to biological temperature, they transformed into gels. The gels reverted back to solutions when the temperature dropped to 25°C. The temperature-dependent gelation of poloxamer solutions could be explained by a configuration change (CitationChoi et al., 1998a; Citation1999). Poloxamer molecules exhibit a well-arranged zigzag configuration. With increasing temperature, the zigzag configuration of poloxamer may be transformed into a close-packed meander configuration, forming a more closely-packed and more viscous gel (CitationChoi et al., 1998a). Hydrochloric acid decreased the viscosity of injectable gels and lengthened the gelation time of the injectable gel. As a possible mechanism by which hydrochloric acid affected the rheological characteristics of the injectable gel base, it is speculated that it could reduce the hydrogen bonding in the cross-linked reticular poloxamer gel (injectable gel base) as a result of its placement between the poloxamer molecules in the gel matrix (CitationSchmolka, 1972; CitationChoi et al., 1998a; Citation1999; CitationYong et al., 2001).
The dissolution profiles of drug from the formulations with different ratios of P188 and hydrochloric acid, which had different viscosities and gelation times, are plotted in and . This part of the study was carried out to determine the effect of P188 and hydrochloric acid content on the dissolution of formulations. Our results showed that P188 decreased significantly the dissolution rates of drug from the injectable gels (). On the other hand, hydrochloric acid increased the dissolution rates of drug from the injectable gels (). However, the injectable gel with 0.1% hydrochloric acid gave no significant dissolution rates of drug compared to that without hydrochloric acid. Thus, the dissolution profile of the drug was generally affected by the formulation, because P 188 and hydrochloric acid had an effect on the viscous properties of the injectable gel.
Figure 4. Effect of P188 on the drug release (a) and release kinetics (b). The injectable gels were composed of doxorubicin, P 407, P 188, and hydrochloric acid. Each value represents the mean ± SD (n = 6).
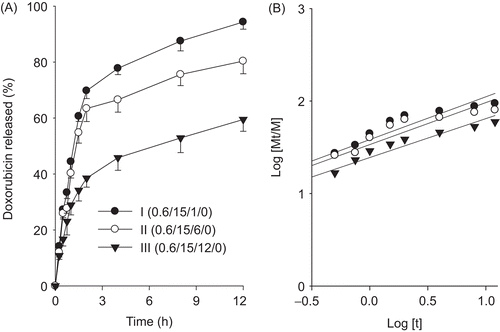
Figure 5. Effect of hydrochloric acid on the drug release (a) and release kinetics (b). The injectable gels were composed of doxorubicin, P 407, P 188, and hydrochloric acid. Each value represents the mean ± SD (n = 6).
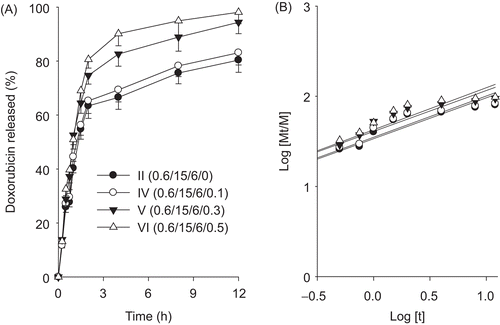
To understand the mechanism of dissolution of drugs from injectable gels, we described the dissolution rate using the following equations:
where Mt/M is the fraction of dissolved drug at time t, k is a characteristic constant of the hydrogel, and n is an indication of the dissolution mechanism.
The n value of 1 corresponds to zero-order dissolution kinetics, 0.5 < n < 1 means a non-Fickian dissolution model and n = 0.5 indicates Fickian diffusion (Higuchi model) (CitationChoi et al., 1998a). From the plot of log (Mt/M) vs log (t) ( and ), the kinetic parameters n and k were calculated. shows that most n values were close to 0.5. These results suggested that the drug was dissolved from hydrogels by Fickian diffusion through the extramicellar aqueous channels of the gel matrix (CitationChoi et al., 1998a; CitationYong et al., 2004). Furthermore, their similar n values indicated that P 188 and hydrochloric acid barely affected the dissolution mechanism. As the k value increases, dissolution becomes faster (CitationChoi et al., 1998a). The less P 188 and more hydrochloric acid were, the greater k value and the faster dissolution of drug from the injectable gel were.
Table 2. Release kinetic parameters.
From these findings, the injectable hydrogel composed of 0.6% doxorubicin, 15% P 407, 6% P 188, and 0.1% hydrochloric acid was selected, because it was easy to administer intramuscularly, most gelled quickly in the body and gave slowest dissolution of drug from the injectable gel. Furthermore, these hydrogels prepared with more than 0.6% doxorubicin showed the drug precipitation.
shows the mean plasma concentration–time profiles of doxorubicin after intramuscular administration of doxorubicin solution and injectable gel to rats at the dose of 10 mg/kg doxorubicin. The doxorubicin solution gave maximum plasma concentrations of drug at 0.08 h followed by a gradual decrease up to 8 h. However, after intramuscular administration of the injectable gel to rats, the plasma concentrations of doxorubicin remained at 0.04–0.06 µg/ml from 0.25 h to 60 h and finally fell to less than 0.003 µg/ml at the end of 96 h. The plasma concentration of doxorubicin in the injectable gel was significantly different from that in the doxorubicin solution (CitationFundarò et al., 2000). Thus, this doxorubicin-loaded injectable gel might reduce the side-effect of doxorubicin due to its no high initial burst concentration (CitationTan et al., 2005).
Figure 6. Blood concentration–time profiles of doxorubicin after intramuscular administration of doxorubicin solution and injectable hydrogel (IV) to rats. The injectable hydrogel (IV) was composed of 0.6% doxorubicin, 15% P 407, 6% P 188, and 0.1% hydrochloric acid. Each value represents the mean ± SD (n = 6). * p < 0.05 compared with doxorubicin solution.
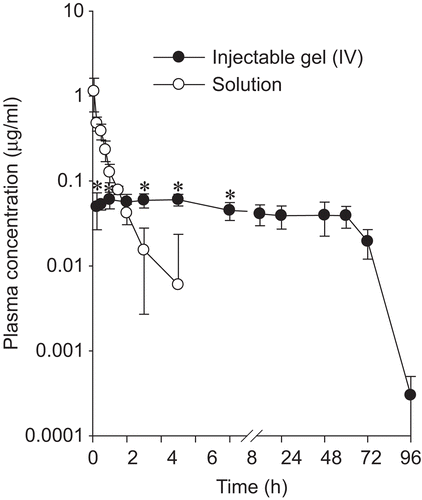
The corresponding pharmacokinetic parameters are listed in . The injectable gel gave a significantly higher AUC and hence ~ 5-fold better bioavailability compared to the doxorubicin solution. Moreover, the MRT, t1/2, and Kel value of doxorubicin from the injectable gel were significantly (p < 0.05) different from those of the doxorubicin solution. Our results suggest that a doxorubicin-loaded injectable gel would be useful for delivering doxorubicin in a pattern that allows sustained release for a long time, leading to better bioavailability. Our results also showed that it gels rapidly in the body, comes into contact with a very small amount of body fluid in the muscle, and very slowly releases the drug by Fickian diffusion (CitationBhattarai et al., 2005; CitationLee & Tae, 2007).
Table 3. Pharmacokinetic parameters of doxorubicin.
Conclusion
The thermo-sensitive injectable gel composed of 0.6% doxorubicin, 15% P 407, 6% P 188, and 0.1% hydrochloric acid was easy to administer intramuscularly and gelled quickly in the body. Moreover, it maintained the plasma concentrations of drug without high initial burst concentration for 60 h and gave an ~ 5-fold higher AUC compared to doxorubicin solution, indicating that it sustained the release of drug for a long time. Thus, it could be an effective form of the delivery of doxorubicin for chronic pain management.
Declaration of Interest
This work was supported by the research fund of Hanyang University (HY-2010-N).
References
- Álvarez-Cedrón, L., Sayalero, M.L., Lanao, J.M. (1999). High-performance liquid chromatographic validated assay of doxorubicin in rat plasma and tissues. J Chromatogr. 721:271–8.
- Arnold, R., Slack, J.E., Straubinger, R.M. (2004). Quantification of Doxorubicin and metabilites in rat plasma and small volume tissue samples by liquid chromatography/electrospray tandem mass spectroscopy. J Chromatogr B. 808:141–52.
- Bhattarai, N., Ramay, H.R., Gunn, J., Matsen, F.A., Zhang, M. (2005). PEG-grafted chitosan as an injectable thermo-sensitive hydrogel for sustained protein release. J Contr Rel. 103:609–24.
- Cabana, A., Ait-kadi, A., Juhasz, J. (1997). Study of the gelation process of polyethylene oxide-polypropylene oxide-polyethylene oxide copolymer (poloxamer 407) aqueous solution. J Colloid Interface Sci. 190:307–12.
- Choi, H.G., Jung, J.H., Ryu, J.M., Yoon, S.J., Sung Oh, Y.K., Kim, C.K. (1998a). Development of in situ-gelling and mucoadhesive acetaminophen liquid suppository. Int J Pharm. 165:33–44.
- Choi, H.G., Kim, M.H., Lee, M.K., Kim, C.K. (1999). Effect of additives on the physicochemical properties of liquid suppository. Int J Pharm. 190:13–19.
- Choi, H.G., Oh, Y.K., Kim, C.K. (1998b). In-situ gelling and mucoadhesive liquid suppository containing acetaminophen: enhanced bioavailability. Int J Pharm. 165;23–32.
- Dumortier, G., Zumer, M., Courarraze, G., Chaumeil, J.C., Grossiord, J.L. (1991). Rheological study of a thermoreversible morphine gel. Drug Dev Ind Pharm. 17:1255–65.
- Fan, H., Dash, A.K. (2001). Effect of cross-linking on the in vitro release kinetics of doxorubicin from gelatin implants. Int J Pharm. 213:103–16.
- Fundarò, A., Cavalli, R., Bargoni, A., Vighetto, D., Zara, G.P., Gasco, M.R. (2000). Non-stealth and stealth solid lipid nanoparticles (SLN) carrying doxorubicin: pharmacokinetics and tissue distribution after i.v. administration to rats. Pharm Res. 42:337–43.
- Gibaldi, M., Perrier, D. (1982). Pharmacokinetics. 2nd ed. New York: Marcel-Dekker.
- Jeong, B., Gutowska, A. (2002). Lessons from nature: stimuli-responsive polymers and their biomedical applications. Trends Biotechnol. 20:305–11.
- Kakinoki, S., Taguchi, T., Saito, H., Tanaka, J., Tateishi, T. (2007). Injectable in situ forming drug delivery system for cancer chemotherapy using a novel tissue adhesive: characterization and in vitro evaluation. Eur J Pharm Biopharm. 66:383–90.
- Kang, G.D., Cheon, S.H., Khang, G., Song, S.C. (2006b). Thermo-sensitive poly (organophosphazene) hydrogels for a controlled drug delivery. Eur J Pharm Biopharm. 63:340–6.
- Kang, G.D., Cheon, S.H., Song, S.C. (2006a). Controlled release of doxorubicin from thermosensitive poly(organophosphazene) hydrogels. Int J Pharm. 319:29–36.
- Klein-Szanto, A.J. (1992). Carcinogenic effects of chemotherapeutic compounds. Prog Clin. 374:167–74.
- Lee, S.Y., Tae, G. (2007). Formulation and in vitro characterization of an in situ gelable, photo-polymerizable Pluronic hydrogel suitable for injection. J Contr Rel. 119:313–9.
- Lenarets, V., Triqueneaux, C., Quarton, M., Falson, F.R., Couvreur, P. (1987). Temperature-dependent rheological behavior of Pluronic F-127 aqueous solutions. Int J Pharm. 31:121–7.
- Minotti, G., Menna, P., Salvatorelli, E., Cairo, G., Gianni, L. (2004). Anthracyclines: molecular advances and pharmacologic developments in antitumor activity and cardiotoxicity. Pharmacol Rev. 56:185–229.
- Paavola, A., Kilpeläinen, I., Yliruusi, J., Rosenberg, P. (2000). Controlled release injectable liposomal gel of ibuprofen for epidural analgesia. Int J Pharm. 199:85–93.
- Raymond, J., Metcalfe, A., Salazkin, I., Schwarz, A. (2004). Temporary vascular occlusion with poloxamer 407. Biomaterials. 25:3983–9.
- Ricci, E.J., Bentley, M.V., Farah, M., Bretas, R.E., Marchetti, J.M. (2002). Rheological characterization of Poloxamer 407 lidocaine hydrochloride gels. Eur J Pharm Sci. 17:161–7.
- Schmolka, I.R. (1972). Artificial skin I. Preparation and properties of Pluronic F-127 gels for treatment of burns. J Biomed Mat Res. 6:571–82.
- Society of Toxicology (SOT), December. (2008). Guiding principles in the use of animals in toxicology. Available online at: www.toxicology.org/AI/FA/guidingprinciples.pdf, accessed
- Tan, E.C., Lin, R., Wang, C.H. (2005). Fabrication of double-walled microspheres for the sustained release of doxorubicin. J Colloid Interf Sci. 291:135–43.
- Veyries, M.L., Couarraze, G., Geiger, S., Agnely, F., Massias, L., Kunzli, B., Faurisson, F., Rouveix, B. (1999). Controlled release of vancomycin from poloxamer 407 gels. Int J Pharm. 192:183–93.
- Yong, C.S., Choi, J.S., Quan, Q.Z., Rhee, J.D., Kim, C.K., Lim, S.J., Kim, K.M., Choi, H.G. (2001). Effect of sodium chloride on the gelation temperature, gel strength and bioadhesive force of poloxamer gels containing diclofenac sodium. Int J Pharm. 226:195–205.
- Yong, C.S., Yang, C.H., Rhee, J.D., Lee, B.J., Kim, D.C., Kim, D.D., Kim, C.K., Choi, J.S., Choi, H.G. (2004). Enhanced rectal bioavailability of ibuprofen in rats by poloxamer 188 and menthol. Int J Pharm. 269:169–76.
- Yun, M.O., Choi, H.G., Jung, J.H., Kim, C.K. (1999). Development of thermoreversible insulin liquid suppository with sodium salicylate. Int J Pharm. 189:137–45.
- Zhao, P., Dash, A.K. (1999). A simple HPLC method using a microbore column for the analysis of doxorubicin. J Pharmaceut Biomed. 20:543–8.