Abstract
Surface modification of liposomes with polymer to optimize drug delivery was well developed recently. The objective of the present work was to evaluate the feasibility of chitosan-coated liposomes (CSLP) as vehicles for anti-sense oligodeoxynucleotides (ASON). CSLP was obtained by adding chitosan dropwise to liposomes under magnetic stirring. The effect of chitosan content on size, zeta potential, and coating efficiency was investigated, which showed that chitosan increased the size and zeta potential of CSLP, and the coating efficiency increased with chitosan content increasing. Agarose gel electrophoresis was employed to evaluate the loading efficiency of CSLP for ASON, from which one could see ASON was completely combined to CSLP when the mass ratio of total lipids:ASON was more than 50:1. MTT assay showed that CSLP took on very low cytotoxicity, which is much lower than chitosan. At last, cell uptake behavior was investigated by a flow cytometer, which showed that CSLP enhanced significantly the COS7 cells uptake of ASON. All the results indicated that the CSLP could be a promising non-viral ASON vehicle.
Introduction
Anti-sense oligodeoxynucleotides (ASON), the synthetic single-stranded DNA fragments that bind to specific intracellular messenger RNA strands (mRNA) to inhibit gene expression, has opened wide perspectives in therapeutics for the treatment of cancer, infectious and inflammatory diseases, or to block cell proliferation and diseases caused thereby (CitationPirollo et al., 2003; CitationFatta et al., 2008). However, the application of therapies based on ASON is limited by their poor stability toward intra- and extracellular nuclease and their low intracellular penetration through the plasma membrane (CitationLebedeva et al., 2000). To overcome this obstacle, plenty of rationally designed vehicles including viral or non-viral delivery systems are developed. Viral systems are very efficient for in vivo transfection, but their major drawbacks, such as potential toxicity, immunogenicity, and inflammatory response, limits their widespread application (CitationRemaut et al., 2007). So non-viral systems based on biocompatible polymers have received increasing attention due to their safety, stability, relative ease of large-scale production, and the lack of intrinsic immunogenicity (CitationMintzer et al., 2009; CitationDutta et al., 2010). Liposomes are the most frequently used non-viral carriers for nucleic acid delivery. Anionic and neutral liposomes are sometimes ineffective because of their poor entrapment efficiency. So liposomes composed of cationic lipids are often required for entrapping nucleic acids efficiently through the formation of complexes which are called lipoplexes (CitationWasungu & Hoekstra, 2006). Such cationic lipids including N-[1-(2,3-dioleyloxy)propyl]-N,N,N-trimethyl-ammonium chloride (DOTMA), 1,2-dioleoyl-3-trimethylammoniumpropane(DOTAP), dioctadecylamido-glycylspermine (DOGS), and so on are expensive, so they are not good drug vehicles in the economic views. Furthermore, liposomes have shown poor storage stability and rapid clearance from the blood. Recently, surface modification of liposomes is well developed to resolve the problem and to optimize target delivery of therapeutics (CitationVenkatesan et al., 2000; CitationKato et al., 2008; CitationYaroslavov et al., 2008; CitationLi et al., 2009). Taking these together, we develop novel positively charged liposomes through coating conventional liposomes with natural cationic polymer, chitosan, and investigate their feasibility for ASON vehicles.
Chitosan is a hydrophilic, biodegradable polysaccharide obtained from partial N-deacetylation of chitin. Because of its beneficial qualities such as low toxicity, low immunogenicity, excellent biocompatibility, as well as a high positive charge density, chitosan has gained considerable interest as a safe delivery system for gene materials including pDNA, ASON, and siRNA (CitationSpringate et al., 2008; CitationJayakumar et al., 2010; CitationMao et al., 2010). Chitosan was also used as a coating material to offer a flexible technology platform for gene delivery. CitationNafee et al. (2007) proved that chitosan-coated PLGA nanoparticles increased ASON encapsulation efficiency and improved the cellular uptake. CitationTahara et al. (2008) indicated that, by coating with chitosan, the loading efficiency of the nucleic acids increased and the initial burst of nucleic acid significantly reduced, resulting in sustained release. So, in the present research, chitosan-coated liposomes are investigated for ASON delivery. Although chitosan-related polymer as a liposomes coating was developed 20 years ago for drugs, especially peptides, delivery to obtain a specified objective such as enhancing the stability in vitro and in vivo, improving the mucoadhesiveness, and facilitating the cellular penetration, chitosan-coated liposomes (CSLP) as gene delivery was rarely reported, and should be investigated further (CitationKhatri et al., 2008).
Materials and methods
Phosphatidylcholine from soybean (95%) was purchased from Lipoid GmbH (Ludwigshafen, Germany), Chitosan with deacetylation degree of 90% and Mw 100 kD was obtained from Zhejiang Aoxing Biotechnology Co., Ltd (Taizhou, China). The anti-sense oligonucleotide with a phosphorothioate backbone (5'-CCC AGC CTT CCA GCT CCT TG-3') was synthesized by Sango (Shanghai, China), the carboxyfluoresceinamine-labeled derivative (5'-FAM-ASON) for the uptake studies was also purchased from Sango. Lipofectamine™ 2000 was purchased from Invitrogen Corporation (Carlsbad,CA). Dulbecco’s modified eagle’s medium (DMEM) and trypsin were purchased from Gibco BRL (Gaithersberg, MD). Fetal bovine serum (FBS) was purchased from Sijiqing Biologic Co., Ltd. (Hangzhou, China). All other chemicals were analytical grade. African Green Monkey SV40-transformed kidney fibroblast cell lines (COS7) were obtained from Institute of Biochemistry and Cell Biology, Shanghai Institutes for Biological Sciences, Chinese Academy of Sciences.
Preparation of liposomes and CSLP
Liposomes were prepared by conventional membrane dispersion method as described previously in the literature with some modifications (CitationGuo et al., 2003). Briefly, appropriate amounts of phosphatidylcholine (200 mg) and cholesterol (50 mg) were dissolved in co-solvent of methanol and dichloroform (1:1). The organic solvent was then evaporated by vacuum rotary evaporator, forming a thin film of lipid at the wall of a 250 ml round-bottomed flask. The film was then hydrated with purified water to make a 20 ml of lipid coarse suspension. Small unilamellar liposomes were produced by probe ultrasonication (JY 92-II ultrasonic processor, Ningbo, China) for 15 min (5 s working and 5 s rest) at 200 W in an ice-water bath.
For the preparation of CSLP, chitosan of various concentrations (0.05, 0.1, 0.2, 0.4, 0.8, and 1%) in acetate buffer solution (pH 4.5) was added dropwise to liposomes of equal volume under magnetic stirring with the speed of 300 rpm and left at room temperature for 2 h.
Characterization of CSLP
Particle size and zeta potential of free liposomes and CLSP were determined by a Zetasizer Nano (Malvern Instruments, Malvern, UK). The effect of the chitosan/lipids ratio on the size and surface charge of the liposomes was studied.
The morphology of chitosan-coated liposomes was characterized using a transmission electron microscope (Hitachi, Tokyo, Japan) by conventional negative staining methods with 2% phosphotungstic acid buffer.
Coating efficiency of CSLP
The coating efficiency of liposomes was determined as the chitosan content coating liposomes vs the total lipids content of CSLP. The CSLP was subjected to ultracentrifugation at 197,000 ×g for 3 h until the sedimentation of chitosan-coated liposomes. The chitosan in supernate was determined by ninhydrin analysis (CitationLeane et al., 2004) and the coating efficiency was calculated using the follow formula:
where CStol was the final concentration of chitosan in complexes, CSsup was the final concentration of chitosan in supernate, and the LP was the final concentration of total lipids in CSLP.
Cytotoxicity of CSLP
The cell viability in the presence of free liposomes, chitosan, and CSLP prepared with chitosan/lipids ratio of 1:1 was determined using the MTT assay. COS7 cells were seeded in a 96-well plate at a density of 1 × 104cells/well in 200 μL of DMEM and incubated for 24 h at 37°C under 5% CO2 atmosphere. After 80~90% confluence the cells were washed with phosphate buffered saline (pH 7.4, PBS) and incubated with free liposomes, chitosan, or CLSP in the concentrations of 10, 50, 100, 500, 1000 mg/L, respectively. After 5 h incubation, the medium was removed and the cells were washed with PBS, followed by incubation with 100 μL MTT containing medium (1 mg/ mL) for 4 h. The medium was subsequently removed, the cells were rinsed with PBS, and the formazan crystals formed in living cells were dissolved in 150 μL DMSO per well. The relative viability (%) was calculated based on the absorbance at 570 nm using a microplate reader (Spectramax M2e, Molecular Devices, California, USA). The viability of non-treated control cells was arbitrarily defined as 100%.
Combination of CSLP with ASON
The same volume of CSLP and ASON were mixed with various mass ratios (ranging from 100:1 to 1:1) and left for 30 min at room temperature to form the CSLP-ASON complexes. The concentration of ASON was 50 μg/ml, and the concentration of the CSLP was adjusted to 50 μg/ml, 250 μg/ml, l.25 mg/ml, 2.5mg/ml, and 5 mg/ml, respectively. The combination efficiency of CSLP with ASON was then analyzed by 1% agarose gel electrophoresis (0.45 h at 80 V, ethidium bromide included for visualization). The images were taken using a Chemi Doc XRS imaging system (Bio Rad, California, USA).
Cellular uptake of ASON
One day before transfection, COS 7 cells were seeded in a 96-well plate at a density of 1.0 × 104 cells/well. When the confluence of cell culture reached 80~90%, the medium were withdrawn and discarded. FAM-labeled ASON complexed with various ratios of CSLP was added in each well, and then appropriate DMEM was added to reach the final incubation volume of 200 μl, final concentration for ASON of 1 μg/ml, and CSLP of 10, 50, 100, 200, 400 μg/ml, respectively. Every sample was in triplicate and lipofectamine® 2000 for positive controls was carried out as described in the manufacturer’s instructions. After 5 h incubation, cells were washed with cooled PBS, collected by trypsinisation, and resuspended in 0.3 ml PBS. Cell-associated fluorescent intensity was analyzed using a flow cytometer (EPICS-XL, Coulter, Florida, USA). For each sample, more than 10,000 events were collected by list-mode data that consisted of side scatter, forward scatter, and fluorescence emission centered at 530 nm (FL1). Total intracellular fluorescent value was calculated by the cross product of mean fluorescent intensity multiplying positive percent.
To investigate the time course of ASON uptake, CSLP-ASON complexes was added to the well pre-cultured with COS7 cells at the concentration of CLSP 100 μg/ml and 1 μg/ml for ASON. After incubated for 15, 30, 60, 100, 150, 230, and 340 min, cells were collected and the total intracellular fluorescent value determined.
Results and discussion
Characteristics of CSLP
All the size distribution functions, the Z-average mean size, intensity-mean size, volume-mean size, and number-mean size of the CSLP and their polydispersity index were determined by Zetasizer Nano® with PCS analysis. Because of the sample with wide size distribution, there was some difference in the above four kinds of mean diameter. The number-mean diameter is similar to the size obtained by transmission electron microscope (), so in this paper the number-mean diameter was given as main characteristic (). The polydispersity index, which implies the size distribution, and surface zeta potential, which implies the stability of the nanosuspension, was also shown in . After coating with chitosan, the hydrodynamic size of CSLP was enhanced, indicating the formation of coating layer on the surface of the liposomes. The liposomes without chitosan-coating took on negative zeta potential, while after coating all the zeta potentials of CSLP were positive. Since chitosan carried high positive charge, the adsorption of chitosan increased the density of positive charge and made the zeta potential positive (CitationDiebold et al., 2007). The zeta potential increased as the chitosan concentration increased to 0.5%, and then it came to a relatively constant value. The high positive charge might play an important role in the flocculation and coagulation resistance. The structured adsorbed polymer film will serve to stabilize the particles against particle–particle interaction, presumably through a mechanism of steric stabilization (CitationGonzalez-Rodriguez et al., 2007).
Table 1. Number-mean diameter (Nmd), polydispersity index (PdI), and surface zeta potential (ZP) of liposomes before and after coating by CS. CS/lipid (w/w) ratios of 1:20, 1:10, 1:5, 2:5, 3:5, 4:5, and 5:5 were evaluated. Each measurement is the mean of at least three measurements and the standard deviation of the mean is reported.
Coating efficiency of CSLP
It is accepted that when the chitosan solution was added to the liposomes colloidal dispersion, the polymer-coated vesicles were produced via the electrostatic interaction between positively charged chitosan and the opposite charge on liposome surface (CitationFilipovic-Grcic et al., 2001). Other mechanisms, such as hydrogen bonding between the polysaccharide and the phospholipid head groups, seem to be also implicated in the process of vesicle coating with chitosan (CitationZaru et al., 2009). The coating efficiency depended on the lipid composition, chitosan/lipids ratios, and so on. In this research, the amount of chitosan coating on the surface of the liposomes indicating the coating efficiency was estimated by measuring the polymer concentration of the supernatant in the centrifuged liposomal suspension after coating. The coating amount of chitosan was found to increase with increasing concentration of chitosan used in coating (). Higher concentration of chitosan solution was not investigated further because of the higher viscosity inducing drop difficulty.
Cytotoxicity of CSLP
The toxicity of CSLP on COS7 cells was assessed by MTT assay. showed the effect of CSLP, liposomes, and chitosan on cell survival after 5-h incubation. Just like liposomes, CSLP showed very low cytotoxicity on COS7 cells, and the cell viability was over 85%, even in the high concentration of 1 mg/ml. The cell viability of chitosan in the same concentration was no more than 30%, which indicated that coating to liposomes could reduce the cytotoxicity of chitosan (p < 0.01). So much more chitosan coating on liposomes than chitosan alone could be selected for drug or gene delivery.
Combination of CSLP with ASON
The surface of CSLP was testified to achieve positive potentials in neutral aqueous solution, so CSLP could combine with negatively charged ASON by the electrostatic interaction to form CSLP-ASON complexes (CitationPeng et al., 2006). To confirm the complex formation, an agarose gel retardation assay was employed. Under the electric field, free negative ASON moved to the positive electrode and displaced in the electrophoresis lane, but the CSLP-ASON complexes were retained in the sample wells because the large size and low charge of the complexes eliminated the electrophoretic mobility. So only excess ASON moved out and presented in the lane. When the mass ratio of total lipids:ASON was more than 50:1, almost all ASON were retarded in the sample wells, suggesting ASON was completely combined to CSLP ().
Cellular uptake of ASON
Flow cytometric (FACS) analysis was performed to evaluate the efficiency of intracellular uptake of the FAM-labeled ASON. To reduce the effect of gating, the values of mean fluorescence intensity and positive ratio was both adopt and their product,which was defined as intracellular total fluorescence, was employed to quantitate the intracellular uptake of FAM-labeled ASON. showed the intracellular total fluorescence after coincubated COS7 cells for 5 h with various complexes containing 1 μg/ml ASON. It was observed that ASON alone or complexed with liposomes poorly penetrated into the cell, while chitosan could improve the intracellular uptake (p < 0.05), and the CSLP improved further (p < 0.05), while the intracellular fluorescence was higher than the lipofectamine™ 2000 in the concentration of vehicle over 50 μg/ml. The enhancement was significantly dependent on concentration of vehicle. Increasing the concentration of vehicle within 100 μg/ ml induced increasing of intracellular fluorescence, but increasing over 100 μg/ml couldn’t enhance intracellular penetration further. On the contrary, the more vehicle over 100 μg/ml was added, the lower the intracellular fluorescence produced. A rational interpretation was that increasing the vehicle within 100 μg/ml would increase the percent of ASON combined to the vehicle, but increasing the vehicle over 100 μg/ml couldn’t increase the percent because almost all ASON was completely combined to the vehicle, which could be seen in . Excess vehicles would compete with ASON-vehicle complexes for cell uptake, which reduced the amount of ASON penetrating into cell. So we also could conclude the uptake of complexes via a saturable mechanism. In all concentrations presented in the studies, the enhancement of CSLP for ASON intracellular uptake was higher than that of chitosan, suggesting there was synergism between chitosan and liposomes. However, the really intracellular uptake mechanism of CSLP complexes required further investigation.
Figure 5. The effect of vehicles and its concentration on extent of ASON uptake in COS7. (a) Total intracellular fluorescence of COS7 after being co-cultured for 5 h with FAM-modified ASON combined to liposomes (


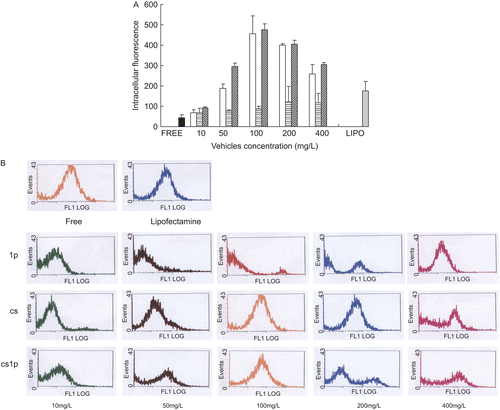
The internalization process was then studied as a function of time with a non-saturating concentration of 100 μg/ml for vehicle and 1 μg/ml for ASON. It was observed in that the intracellular total fluorescence increased as a function of time up and then slop to a relatively constant value, at which the uptake of ASON into cell was offset by the intracellular elimination. The cell uptakes of ASON combined with chitosan at various times were all lower than that combined with CSLP (p < 0.01). These also confirmed that there was synergism between chitosan and liposomes.
Figure 6. The uptake dynamics of ASON in COS7. (A) Total intracellular fluorescence of COS7 after being co-cultured for various times with FAM-modified ASON combined to chitosan (□) and chitosan-coated liposomes (◊). (B) Flow cytometric profiles of COS7 after being co-cultured with FAM-modified ASON combined to chitosan (cs) and chitosan-coated liposomes (cslp) for 15, 30, 60, 100, 150, 230, and 340 min (from left to right).
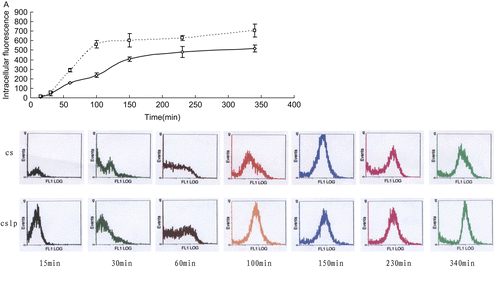
Conclusion
In this work, chitosan-coated liposomes were introduced as vehicles for ASON. Adding chitosan solution dropwise to liposomes under magnetic stirring could produce CSLP with nano size and positive surface potential. The CSLP took on very low cytotoxity, high combination efficiency for ASON, and could enhance the uptake of ASON remarkably, suggesting CSLP may be a promising gene vehicle for further investigation.
Declaration of Interest
The present work was supported by Natural Science Foundation of Zhejiang Province (No.Y206534).
References
- Diebold Y, Jarrín M, Sáez V, Carvalho EL, Orea M, Calonge M, Seijo B, Alonso MJ. (2007). Ocular drug delivery by liposome-chitosan nanoparticle complexes (LCS-NP). Biomaterials. 28:1553–64.
- Dutta T, Jain NK, Nigel, AJ, Parekh HS. (2010). Dendrimer nanocarriers as versatile vectors in gene delivery. Nanomed: Nanotechnol Biol Med. 6:25–34.
- Fatta E, Bochot A. (2008). State of the art and perspectives for the delivery of antisense oligonucleotides and siRNA by polymeric nanocarriers. Int J Pharm. 364:237–48.
- Filipovic-Grcic J, Skalko-Basnet N, Jalsenjak I. (2001). Mucoadhesive chitosan-coated liposomes: characteristics and stability. J Microencaps. 18:3–12.
- Gonzalez-Rodriguez ML, Barros LB, Palmaa J, González-Rodríguez PL, Rabasco AM. (2007). Application of statistical experimental design to study the formulation variables influencing the coating process of lidocaine liposomes. Int J Pharm. 337:336–45.
- Guo J, Ping Q, Jiang G, Huang L, Tong Y. (2003). Chitosan-coated liposomes: characterization and interaction with leuprolide. Int J Pharm. 260:167–73.
- Jayakumar R, Chennazhi KP, Muzzarelli RA, Tamura H, Nair SV, Selvamurugan N. (2010). Chitosan conjugated DNA nanoparticles in gene therapy. Carbohyd Polym. 79:1–8.
- Kato C, Kajiwara T, Numazaki M, Takagi H, Kojima N (2008). Oligomannose-coated liposomes activate ERK via Src kinases and PI3K/Akt in J774A.1 cells. Biochem Biophys Res Commun. 372:898–901.
- Khatri K, Goyal AK, Gupta PN, Mishra N, Mehta A, Vyas SP. (2008). Surface modified liposomes for nasal delivery of DNA vaccine. Vaccine. 26:2225–33.
- Leane MM, Nankervis R, Smith A, Illum L. (2004). Use of the ninhydrin assay to measure the release of chitosan from oral solid dosage forms. Int J Pharm. 271:241–9.
- Lebedeva I, Benimetskaya L, Stein CA, Vilenchik M. (2000). Cellular delivery of antisense oligonucleotides. Eur J Pharm Biopharm. 50:101–19.
- Li N, Zhuang CY, Wang M, Sun X, Nie S, Pan W (2009). Liposome coated with low molecular weight chitosan and its potential use in ocular drug delivery. Int J Pharm. 379:131–8.
- Mao, S., Sun, W., Kissel, T. (2010). Chitosan-based formulations for delivery of DNA and siRNA. Adv Drug Deliver Rev. 62:12–27.
- Mintzer, M.A., Simanek, E.E. (2009). Nonviral vectors for gene delivery. Chem Rev. 109:259–302.
- Nafee N, Taetz S, Schneide M, Schaefer UF, Lehr CM. (2007). Chitosan-coated PLGA nanoparticles for DNA/RNA delivery: effect of the formulation parameters on complexation and transfection of antisense oligonucleotides. Nanomed: Nanotechnol Biol Med. 3:173–83.
- Peng J, He X, Wang K, Tan W, Li H, Xing X, Wang Y. (2006). An antisense oligonucleotide carrier based on amino silica nanoparticles for antisense inhibition of cancer cells. Nanomed: Nanotechnol Biol Med. 2:113–20.
- Pirollo KF, Rai A, Sleer LS, Chang EH. (2003). Antisense therapeutics: from theory to clinical practice. Pharm Ther. 99:55–77.
- Remaut K, Sanders NN, Geest BG, Braeckmans K, Demeester J. and De Smedt S.C. (2007). Nucleic acid delivery: where material sciences and bio-sciences meet. Mater Sci Eng R. 58:117–61.
- Springate CM, Jackson JK, Gleave ME, Burt HM. (2008). Clusterin antisense complexed with chitosan for controlled intratumoral delivery. Int J Pharm. 350:53–64.
- Tahara K, Sakai T, Yamamoto H, Takeuchi H, Kawashima Y (2008). Establishing chitosan coated PLGA nanosphere platform loaded with wide variety of nucleic acid by complexation with cationic compound for gene delivery. Int J Pharm. 354:210–6.
- Venkatesan, N., Vyas, S.P. (2000). Polysaccharide coated liposomes for oral immunization -development and characterization. Int J Pharm. 203:169–77.
- Wasungu, L., Hoekstra, D. (2006). Cationic lipids, lipoplexes and intracellular delivery of genes. J Contr Rel. 116:255–64.
- Yaroslavov AA, Rakhnyanskaya AA, Yaroslavova EG, Efimova AA, Menger FM. (2008). Polyelectrolyte-coated liposomes: stabilization of the interfacial complexes. Adv Colloid Interfac. 142:43–52.
- Zaru, M., Manca, M.L., Fadda, A.M. (2009). Chitosan-coated liposomes for delivery to lungs by nebulisation. Colloids Surfaces B. 71:88–95.