Abstract
An economical, convenient portable drug delivery system combining osmotic pump with subcutaneous infusion was developed, which was composed of three primary components: water chamber, osmotic pump chamber and support base. Ceftriaxone sodium (CRO) was selected as the model drug and osmotic pump tablets were prepared. The influence of osmotic agents on drug release profiles was evaluated. As the adjustment made by the osmotic agents was limited, the compositions of semipermeable membrane were investigated to determine significant associations of factors based on orthogonal design. The in vitro release profiles of the optimum formulation achieved to the predetermined value (15 ± 3 min for the initial release time Ti and 5.75 ± 0.25 h for the extent release time Te). The pharmacokinetic profiles of this drug delivery system were evaluated in Beagle dogs. In vivo results demonstrated that the osmotic pump subcutaneous infusion administration was equivalent to intravenous injection administration in terms of bioavailability. Moreover, constant drug plasma levels with minimized fluctuations could be achieved with this osmotic pump subcutaneous infusion system, compared with intravenous injection.
Introduction
Besides the drug itself, the right dosage over time is crucial for an effective therapy. Rate-controlled release systems allow maintaining the drug concentration within the body at an optimum level. This minimizes the risk of disadvantageous side effects, poor therapeutic activity or even adverse effects (Herrlich et al., Citation2012). Rate-controlled release of drug can be achieved through different chronic drug administration approaches, such as intravenous infusion, subcutaneous infusion and subcutaneously implanted osmotic pumps.
The first osmotic pumps featuring three compartments were developed by Rose and Nelson in 1955 (Rose & Nelson, Citation1955). Osmotic technologies can be used to improve the pharmacokinetic properties of drugs by better adjustment of the release rate with respect to conventional tablets or pills. Therefore, in the last two decades, various osmotic pump systems had been developed for oral drug delivery and implantable systems. Benefited from the application of microengineering and microelectromechanical technologies, osmotic devices were developed toward miniaturization and system integration. Osmotic micropumps can provide zero-order drug release rates and enable drug delivery either in a site-specific or systemic way. This facilitated drug delivery systems for long-term use not limited by gastrointestinal transit time and first-pass metabolism (Herrlich et al., Citation2012). Up to now, several devices, such as ALZET® (Theeuwes & Yum, Citation1976), LiRIS® (Lee & Cima, Citation2011), IntelliDrug (Scholz et al., Citation2008), and Viadur® (Bayer AG, Leverkusen, Germany), have been commercially available or under active development. The ALZET® mini-osmotic pumps developed by the Alza Corporation were probably the most prominent examples of miniature osmotic pumps and commonly used in the research sector for preclinical animal testing. A single ALZET® pump could provide up to 6 weeks of continuous infusion. Usually, these delicate devices were expensive and must be implanted in the body and withdrawn after depletion of the drug in hospital.
Subcutaneous infusion administration offers a safe, simple, effective alternative to intravenous or intramuscular injections when oral medications cannot be used. Continuous subcutaneous infusion reduces the peaks and valleys in plasma drug concentration, making this route effective in treating diabetes (González-Michaca et al., Citation2002; Fujikawa et al., Citation2012), pain in advanced cancer (Storey et al., Citation1990; Hernandez et al., Citation2008), primary immunodeficiency disorders (Hansen et al., Citation2002; Wasserman et al., Citation2012), cancer (Leahy et al., Citation1992), schizophrenia (Marchese et al., Citation2010), gastrointestinal obstruction (De Conno et al., Citation1991) and hereditary angioedema (Jiang et al., Citation2010). This administration route is suited for home care of ambulatory outpatients. However, conventional portable infusion pumps are expensive and complicated, and are perceived to negatively affect the quality of life because of their bulk and inconvenience (Moulin et al., Citation1991). Moreover, it takes a period of time in training to master the use of infusion pumps (Pasero, Citation2002). Therefore, smaller and more convenient subcutaneous infusion systems, such as the MEDIPAD Drug Delivery System, have been developed (Mikkelsen Lynch et al., Citation2000).
In this study, an economic, convenient portable drug delivery system combining osmotic pump with subcutaneous infusion was developed. Ceftriaxone sodium (CRO), which was a widely used injectable broad-spectrum cephalosporin, was selected as the model drug. The influence of osmotic agents on drug release profiles was evaluated. Because the adjustment of osmotic agents was limited, the compositions of semipermeable membrane were investigated to determine significant associations of factors based on orthogonal design. Furthermore, the pharmacokinetic profiles of continuous subcutaneous infusion with this new drug delivery system were determined, compared with that of intravenous injection in Beagle dogs.
Materials and methods
Materials and animals
Ceftriaxone sodium was provided by Meiya Pharmaceutical Co., Ltd. (Liaoning, China). Polyethylene glycol was purchased from Sasol Germany (Hamburg, Germany). Cellulose acetate was purchased from YouPuHui Pharmaceutical Co., Ltd. (Shenzhen, China). Octylamin was purchased from Merk Schuchardt OHG (Hohenbrunn, Germany). All other chemicals and solvents, which were purchased from Beijing Chemical Agent Co. (China), were of analytical or HPLC grade.
Beagle dogs were purchased from Laboratory Animals Center of Academy of Military Medical Sciences (China). All animals were handled according to the code of ethics in research, training and testing of drugs as laid down by the Animal Care and Use Ethics Committee of Academy of Military Medical Sciences.
Osmotic pump infusion drug delivery system design
The osmotic pump infusion drug delivery system () was composed of three primary components: water chamber, osmotic pump chamber and support base. A cylindrical water chamber was used to store water for injection. There were some pores sealed with films at the bottom of water chamber. At the center of water chamber, a smaller cylindrical osmotic pump chamber was used for placing osmotic pump tablet. At the bottom of osmotic pump chamber, there was a tablet holder with some pores. The infusion channel was located in the center of the tablet holder connected with a needle. Another cylindrical component was an adhesive-backed support base with paracentetic spicule and central pore.
Preparation of the formulations
A dose (250 mg) of CRO and osmotic agent were used in the formulations. The effects of different osmotic agents including sodium chloride (NaCl), lactose, sucrose, glucose and mannitol on the release profiles were examined. Formulations were compressed in ZP-5 rotary tablet press (Shanghai Tianhe Pharmaceutical Device Co., Ltd., China) using a 12-mm diameter punch manually. The hardness of the core tablets was 60 N. The tablets were coated in a pan coater (Jiangsu Taizhou Pharmaceutical Device Co., Ltd., China) with coating solution of cellulose acetate (4.5%, w/v) and diethyl phthalate (DEP) in 95% acetone. Polyethylene glycol was added to the coating solution as a plasticizer. The temperature of inlet air was 45 °C; spray rate was 5 mL/min; pan-rotating rate was 26 rpm. The tablets were coated and then dried at 40 °C for 12 h. A 450 μm orifice for the delivery of CRO was created by a laser-beam drilling machine (Nanjing Rich Electronic Engineering Technology Industry Co., Ltd., China).
Dissolution rate test
In vitro release test was performed according to USP paddle method in a modified dissolution apparatus (D-800LS, Precise Apparatus of Tianjin University Co., Ltd., China). The instant adhesive was applied to the edge of the tablet holder, and then the osmotic pump tablet was pasted to the holder. Markers around the orifice were made to ensure the orifice of tablet opposite the infusion channel. Then the device was fixed in a support. The needlepoint exactly contacted the surface of dissolution medium. The dissolution medium was 500 mL physiological saline (32 °C) stirred at 100 rpm. When the films at the bottom of the water chamber were pierced by paracentetic spicule, water for injection could reflow into osmotic pump chamber through the support base. The time from water contacted osmotic pump tablet to drug solution released from the needlepoint was defined as the initial release time (Ti). The complete drug release time was defined as the extend release time (Te). Samples taken at predetermined time intervals were filtered and then determined by HPLC analysis with a Waters HPLC system (Dual λ Absorbance Detector 2487, Binary Pump 1525, Waters, USA). The UV detector wavelength was set at 254 nm. The separation of CRO was achieved by injection of 20 μL samples into a reversed phase C18 analytical column (Agela Venusil XBP C18, 4.6 × 250 mm, 5 μm, Agela Technologies, Inc., Boston, MA) under isocratic conditions which contained acetonitrile and 0.03 mol/L octylamin solution (72:28, v/v) at a flow rate of 1.0 mL/min.
In vivo study
Healthy male Beagle dogs, having mean weight of 10 ± 1.5 kg, were used for in vivo study. For osmotic pump infusion group, the infusion needle was stuck into depilated back of dog and fixed. Six hours after administration the drug delivery devices were removed. For control group, dogs were injected intravenously with ceftriaxone sodium injection (Rocephin®, F. Hoffmann-La Roche Ltd., Switzerland) at a dose of 250 mg. Blood samples were collected at 0.083, 0.25, 0.5, 1, 1.5, 2, 3, 4, 5, 6, 8, 10, 12, 24, 30 hours after administration. The in vitro analysis of samples was performed in an Agilent 1200 HPLC system (Pump G1312B, UV detector G1316B, Autosampler 1367C, Degasser G1379B, Agilent Technologies, Santa Clara, CA). The UV detector wavelength was set at 254 nm. The separation of CRO and cefalexin (internal standard) was achieved by automated injection of 5 μL samples into a reversed phase C18 analytical column (Agela Venusil XBP C18, 2.1 × 50 mm, 3 μm, Agela Technologies, Inc., Boston, MA). The mobile phase consisted of 20 mM phosphate buffer solution (pH 7.0) and acetonitrile containing 0.125% (w/v) hexadecyltrimethylammonium bromide (64:36, v/v), with a flow rate of 0.3 mL/min.
Statistical analysis
Data were expressed as mean ± standard deviation in the figures and tables, with p < 0.05 regarded as statistically significant. Statistical tests were performed using a one-way analysis of variance with a Student’s t-test. All testing was done using the SPSS 16.0 (SPSS Inc., Somers, New York).
Results and discussion
Osmotic pump infusion drug delivery system design
The system size was about 3 cm (diameter) × 1.5 cm (height). The total weight of this device containing water and tablet was less than 20 g. This infusion device can be inconspicuously worn on the body. During operation, the films at the bottom of water chamber were pierced by paracentetic spicule and the water in water chamber entered the space of the support base and then reflowed into osmotic pump chamber. At the same time, the needle in the tablet holder crossed the central pore in the support base. After contacting with the semipermeable membrane of osmotic pump, water entered and dissolved the active material in the tablet core, consequently pressure formed in the preparation. The pressure induced drug to release at a slow but continuous rate. The time of continuous infusion was decided by the osmotic pump tablet in the device. Osmotic pump tablets of specified drug with predetermined dose and release time may be prepared according to different requirements. Furthermore, this device was small, easy to use and suited for home care and self-help administration without requirement of medical facilities and professionals.
Development of CRO osmotic pump tablets
Influence of the osmotic agents on drug release profile
To obtain the designated drug release profile, various osmotic agents were investigated, including NaCl, lactose, sucrose, glucose and mannitol (). For traditional osmotic pump tablets, osmotic agents, retardant agents, solubilizers and propellants were usually used to control the drug release rate. But most propellants were polymers and could not be used in injection preparation. Therefore, several commonly used additives in injection were screened as osmotic agents. The initial release time (Ti) and the extend release time (Te) were employed to evaluate the release profiles of various formulations. The Te value proposed was six hours, while Ti value was kept as small as possible. The results manifested that both Te and Ti showed a maximum value when lactose was used. The tablets with NaCl or glucose as the osmotic agent had relatively short Te and Ti. The onset of drug release took place at the end of a certain time (lag-time); it apparently began after an osmotic pressure built up in the tablet (Özdemir & Sahin, Citation1997). Different from traditional osmotic pump tablets, the orifices could not contact dissolution medium directly in this device, hence the release of drug solution from orifices did not start until the active materials around orifices had been dissolved. Therefore, the Ti and Te values may be affected not only by osmotic pressure but also by hydrophilicity of osmotic agents. According to Van’t Hoff equation (Grattoni et al., Citation2007), the same amount of mannitol created the lowest osmotic pressure in tablet resulted in the longest Te. The same amount of lactose created relatively high osmotic pressure, but the rate of water hydration of tablet core with lactose as the osmotic agent was lower than that of tablet core with mannitol as the osmotic agent. So, the time of water diffusion into tablet core increased, which lead to the longest Ti and Te.
Figure 2. Influence of (A) the osmotic agents, (B) the amount of glucose on the release of CRO. The asterisk of Ti indicates p < 0.05 versus lactose, sucrose and mannitol. The asterisk of Te indicates p < 0.05 versus lactose and mannitol.
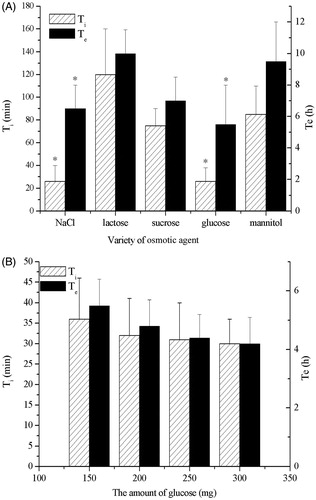
The hygroscopicity of NaCl was larger compared with that of glucose, and thus glucose was chosen as the osmotic agent finally. The influence of glucose amount on drug release profiles () showed that both Ti and Te value decreased as glucose amount increased from 150 to 300 mg. But there was no statistical significance (p > 0.05). The increase of osmotic agent proportion in the tablet core composition increased the rate of water hydration of the core decreasing the lag time (Malaterre et al., Citation2009b). So, the tablet core was hydrated easily when the amount of glucose in tablet core increased. This result could be explained by the fact that the swelling of the drug layer was responsible for the initial drug fraction delivered through the orifice as recently hypothesized in the literature (Malaterre et al., Citation2009a). Meanwhile, a greater amount of osmogent would create a higher osmotic pressure difference, hence a greater driving force to push the drug solution from the system (Makhija & Vavia, Citation2003). But the adjustment of drug release induced by the amount of glucose was limited by tablet weight and filling volume of tablet press. Therefore, the amount of glucose was determined as 250 mg.
Influence of plasticizers and pore formers on drug release profile
The membrane composition was the key to control the drug release rate. The influence of plasticizers and pore formers on drug release profiles was investigated. The tablets core was coated by coating solution with PEG and DEP. showed that the Ti and Te increased as the molecular weight of PEG increased. The effects of 1.5, 2.0 and 2.5% (w/v) PEG 1500 solutions were further investigated (). The results indicated that there was correlation between the Ti or Te and PEG 1500 amount: the higher the level of PEG1500 was, the shorter Ti or Te was. The role of PEG in the membrane had been described in literature with a dual functionality of plasticizer (Guo, Citation1993) and pore former (Rani & Mishra, Citation2004). As PEG was a hydrophilic leachable pore former, it could be leached easily and left behind porous in the semipermeable membrane of the osmotic pump tablets. Because the molecular weight of PEG was higher, it needed more time to dissolve PEG and moisten the tablets. This was consistent with results found in push-pull osmotic pumps (Malaterre et al., Citation2009b). Furthermore, the water permeability through a semipermeable membrane was correlated with the leachable agent proportion in the membrane composition (Bindschaedler et al., 1987). As the proportion of PEG (Makhija & Vavia, Citation2003) or other hydrophilic plasticizer (Shokri et al., Citation2008) increased in the membrane, more of it dissolved in water. Thus, the membrane permeability and drug release rate increased with the content of hydrophilic plasticizer. Moreover, the PEG/CA ratio also affected the porosity and water permeability of membrane. The PEG/CA ratio was 1:3 in the formulation, which could form appropriate pores after PEG leached. Coatings with lower PEG/CA ratio decreased water permeability as a function of decreasing PEG/CA ratio (Kumaravelrajan et al., Citation2010). Maybe below a ratio of 1:6 PEG/CA, the pores were not observable probably due to their low size (Malaterre et al., Citation2009b).
Figure 3. Influence of (A) the PEG, (B) the level of PEG 1500, (C) the amount of DEP on the release of CRO. In Figure A, the asterisk of Ti or Te indicates p < 0.05 versus PEG 6000. In Figure B, the asterisk of Ti or Te indicates p < 0.05 versus 2.5% (w/v) PEG 1500 solutions. In Figure C, the asterisk of Ti indicates p < 0.05 versus 1.0% and 1.5% DEP in coating solution (v/v).
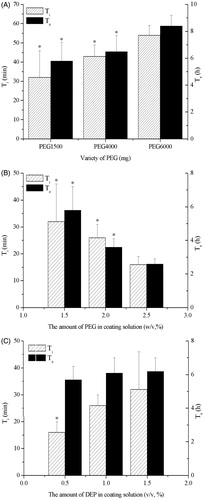
Conversely, with the increase of DEP amount in the coating solution, Ti or Te obviously increased (). DEP was a hydrophobic plasticizer and channeling agent, which could be used to control the drug release rate. Because of the hydrophobic character of DEP, which was similar to other hydrophobic plasticizer such as castor oil, hydrophobic plasticizer decreased the release rate, whereas the presence of hydrophilic plasticizer increased the release rate, when they were incorporated into the membrane (Shokri et al., Citation2008). As DEP was insoluble in water, it was difficult to leach. The residual DEP would resist water diffusion and, as a consequence, the drug release rate decreased (Shokri et al., Citation2008).
Influence of weight gain of tablet on drug release profile
To investigate the effect of weight gain of tablet on the release of CRO, osmotic pump tablets with weight gain of 8%, 11% and 14%, respectively, were prepared. Ti and Te values increased as the weight gain of tablet increased (). The higher weight gain of tablet induced not only slower release rate but also longer lag time. The drug release rate was directly related to the rate that water entered the tablet core. The rate of water ingress was dependent on the osmotic pressure of the core and the permeability of the coating: the thicker coating, the lower water permeability. According to studies of Ofori-Kwakye et al. (Ofori-Kwakye & Fell, Citation2003) and Liu et al. (Liu et al., Citation2000), the increase of coating level would result in the decrease of water imbibing through the membrane; thus, the rate of hydration of the drug layer decreased, which resulted in decrease of release rate of drugs.
Optimization of the formulations
Based on the influence of individual factor on the drug release, the amount of PEG1500, the amount of DEP and the weight gain of tablet were determined as the key factors for further optimization of the formulations. Osmotic pump tablets with various formulations were prepared according to L9 (34) orthogonal design (). Comprehensive grading method was employed to evaluate the release profiles of various formulations compared with the ideal release profiles. The score of various formulations was calculated by the following equation:
Where S was total score of the formulations, t0.25, t2.75 and t5.75 were the cumulative released percentage at 0.25, 2.75 and 5.75 h, respectively. The ideal cumulative released percentages at 0.25, 2.75 and 5.75 h were supposed to be 10%, 50% and 100%, respectively. Apparently, the smaller the total score was, the closer to the ideal release profile was. The level which got the smallest score was chosen as the optimal level of each factor. The results of orthogonal design showed that the optimal formulation contained 1.5% (w/v) PEG1500 and 0.5% (v/v) DEP in coating solution, and 11% weight gain of tablet. The osmotic pump tablets with the optimal formulation were prepared and in vitro release was tested. The Ti and Te of optimal formulation were 15 ± 3 min and 5.75 ± 0.25 h, respectively. As shown in , the optimal osmotic pump tablet was able to deliver CRO at an approximate zero-order up to 6 h (N = 6). The linear fitting equation for the release of the optimized formulation was Y = 14.736X + 3.642, with linear correlation coefficient 0.997.
Table 1. Results of orthogonal design (L9 (34)).
In vivo study
The mean plasma concentration of CRO versus time for subcutaneous infusion and intravenous injection was shown as . Obviously, the plasma concentration of CRO reached peak as soon as intravenous injection and the Cmax was 85.92 ± 9.82 μg/mL. But subsequently the plasma concentration of CRO decreased rapidly and remained about 10% of Cmax after 1.5 h. However, as for subcutaneous infusion, the plasma concentration of CRO rose gradually, almost reached the plateau level at about 0.5–1 h. The Cmax for subcutaneous infusion was 5.07 ± 0.48 μg/mL, which was remarkably lower than that for intravenous injection. After 12 h the plasma concentration of CRO for subcutaneous infusion was about 1 μg/mL, which was above the MIC90 (0.12–0.25 μg/mL). Remarkably, the fluctuation of plasma concentration for this osmotic pump subcutaneous infusion system was significantly smaller, compared with intravenous injection, which were similar to osmotic micropump or subcutaneous infusion (Mikkelsen Lynch et al., Citation2000; Fisher et al., Citation2003; Herrmanna et al., Citation2009). Steady drug serum levels produced by this delivery device would result in the desired pharmacodynamic efficacy and mild adverse effects (Mikkelsen Lynch et al., Citation2000; Wright et al., Citation2001). The AUC(0–12) for subcutaneous infusion was essentially equal to that for intravenous injection (46.11 ± 0.59 μg·h/mL for intravenous injection and 42.98 ± 2.09 μg·h/mL for subcutaneous infusion). The F ratio for subcutaneous infusion compared to intravenous injection was 97 ± 6% for AUC(0-inf), which suggested that the osmotic pump subcutaneous infusion administration was equivalent to intravenous injection administration in terms of bioavailability.
Conclusion
A novel portable drug delivery system combining osmotic pump and subcutaneous infusion was developed. The compositions of CRO osmotic pump tablets were screened and optimized. The in vitro release profile of the optimum formulation achieved to the predetermined value. The pharmacokinetic profiles of this new drug delivery system were evaluated in Beagle dogs. In vivo results demonstrated that the osmotic pump subcutaneous infusion administration was equivalent to intravenous injection administration in terms of bioavailability. Moreover, constant drug plasma levels with minimized fluctuations could be achieved with this osmotic pump subcutaneous infusion system, compared with intravenous injection. In addition, the novel portable drug delivery system may be as a potential platform for the constant delivery of more drugs, such as analgesics, psychotropic agent or other therapeutic drugs.
Declaration of interest
The authors report no conflicts of interest. The authors alone are responsible for the content and writing of this article.
Financial support was provided by the Major State Basic Research Development Program of China (Grant No. 2009CB30305) and Important National Science & Technology Specific Projects (Grant No. 2012ZX09301003-001-009).
References
- De Conno F, Caraceni A, Zecca E, et al. (1991). Continuous subcutaneous infusion of hyoscine butylbromide reduces secretions in patients with gastrointestinal obstruction. J Pain Symptom Manage 6:484–6
- Fisher DM, Kellett N, Lenhardt R. (2003). Pharmacokinetics of an implanted osmotic pump delivering sufentanil for the treatment of chronic pain. Anesthesiology 99:929–37
- Fujikawa T, Imbe H, Date M, et al. (2012). Severe insulin allergy successfully treated with continuous subcutaneous insulin infusion. Diabetes Res Clin Pract 97:e31–3
- González-Michaca L, Ahumada M, Ponce-de-León S. (2002). Insulin subcutaneous application vs. continuous infusion for postoperative blood glucose control in patients with non-insulin-dependent diabetes mellitus. Arch Med Res 33:48–52
- Grattoni A, Merlo M, Ferrari M. (2007). Osmotic pressure beyond concentration restrictions. J Phys Chem B 111:11770–5
- Guo JH. (1993). Effects of plasticizers on water permeation and mechanical properties of cellulose-acetate-antiplasticization in slightly plasticized polymer film. Dr Dev Ind Pharm 19:1541–55
- Hansen S, Gustafson R, Smith CIE, et al. (2002). Express subcutaneous IgG infusions: decreased time of delivery with maintained safety. Clin Immunol 104:237–41
- Hernandez G, Haines E, Shizgal P. (2008). Potentiation of intracranial self-stimulation during prolonged subcutaneous infusion of cocaine. J Neurosci Methods 175:79–87
- Herrlich S, Spieth S, Messner S, et al. (2012). Osmotic micropumps for drug delivery. Adv Drug Deliv Rev 64:1617–27
- Herrmanna M, Henneicke H, Street J, et al. (2009). The challenge of continuous exogenous glucocorticoid administration in mice. Steroids 74:245–9
- Jiang H, Zhang HM, Frank MM. (2010). Subcutaneous infusion of human C1 inhibitor in swine. Clin Immunol 136:323–8
- Kumaravelrajan R, Narayanan N, Suba V, et al. (2010). Simultaneous delivery of nifedipine and metoprolol tartarate using sandwiched osmotic pump tablet system. Int J Pharm 399:60–70
- Leahy MG, Pitfield D, Popert S, et al. (1992). Phase I study comparing continuous infusion of recombinant interleukin-2 by subcutaneous or intravenous administration. Eur J Cancer 28:1049–51
- Lee H, Cima MJ. (2011). An intravesical device for the sustained delivery of lidocaine to the bladder. J Control Release 149:133–9
- Liu L, Ku J, Khang G, et al. (2000). Nifedipine controlled delivery by sandwiched osmotic tablet system. J Control Release 68:145–56
- Makhija SN, Vavia PR. (2003). Controlled porosity osmotic pump-based controlled release systems of pseudoephedrine I. Cellulose acetate as a semipermeable membrane. J Control Release 89:5–18
- Malaterre V, Metz H, Ogorka J, et al. (2009a). Benchtop-magnetic resonance imaging (BT-MRI) characterization on the water-insoluble drug release from push-pull osmotic systems. J Control Release 133:31–6
- Malaterre V, Ogorka J, Loggia N, et al. (2009b). Approach to design push-pull osmotic pumps. Int J Pharm 376:56–62
- Marchese G, Pittau B, Casu G, et al. (2010). A comparison of continuous subcutaneous paliperidone infusion and repeated subcutaneous injection of risperidone free-base in rats. Eur Psychiatry 25:92–100
- Mikkelsen Lynch P, Butler J, Huerta D, et al. (2000). A pharmacokinetic and tolerability evaluation of two continuous subcutaneous infusion systems compared to an oral controlled-release morphine. J Pain Symptom Manage 19:348–56
- Moulin DE, Kreeft JH, Murray-Parsons N, et al. (1991). Comparison of continuous subcutaneous and intravenous hydromorphone infusions for management of cancer pain. Lancet 337:465–8
- Ofori-Kwakye K, Fell JT. (2003). Biphasic drug release from film-coated tablets. Int J Pharm 250:431–40
- Pasero C. (2002). Subcutaneous opioid infusion. Am J Nurs 102:61–2
- Rani M, Mishra B. (2004). Comparative in vitro and in vivo evaluation of matrix, osmotic matrix, and osmotic pump tablets for controlled delivery of diclofenac sodium. AAPS PharmSciTech 5:153--9
- Rose S, Nelson JF. (1955). A continuous long-term injector. Aust J Exp Biol Med Sci 33:415–9
- Scholz OA, Wolff A, Schumacher A, et al. (2008). Drug delivery from the oral cavity: focus on a novel mechatronic delivery device. Drug Discov Today 13:247–53
- Shokri J, Ahmadi P, Rashidi P, et al. (2008). Swellable elementary osmotic pump (SEOP): an effective device for delivery of poorly water-soluble drugs. Eur J Pharm Biopharm 68:289–97
- Storey P, Hill HH Jr, St Louis RH, et al. (1990). Subcutaneous infusions for control of cancer symptoms. J Pain Symptom Manage 5:33–41
- Theeuwes F, Yum SI. (1976). Principles of the design and operation of generic osmotic pumps for the delivery of semisolid or liquid drug formulations. Ann Biomed Eng 4:343–53
- Wasserman RL, Melamed I, Stein MR, et al. (2012). Recombinant human hyaluronidase-facilitated subcutaneous infusion of human immunoglobulins for primary immunodeficiency. J Allergy Clin Immunol 130:951–7
- Wright JC, Leonard ST, Stevenson, CL, et al. (2001). An in vivo/in vitro comparison with a leuprolide osmotic implant for the treatment of prostate cancer. J Control Release 75:1–10
- Özdemir N, Sahin J. (1997). Design of a controlled release osmotic pump system of ibuprofen. Int J Pharm 158:91–7