Abstract
To evaluate the influence of diabetes on the permeation of dexamethasone acetate (DA) and dexamethansone sodium phosphate (DSP), the two major dexamethansone esters in clinical practice, when applied percutaneously, histochemical staining was used to determine the skin morphology; improved Franz diffusion cells and microdialysis were used to assess the percutaneous permeation of DA and DSP in normal and diabetic rats. Histopathological examination showed that the epidermal tissue of diabetic rat was much thinner, the epidermal cell layer was less clear and the stratified arrangement of epidemic cell had almost disappeared and progressive atrophy were developed on the subcutaneous fat. In vitro studies showed that the cumulative and the penetrated DSP amount in Group DM were higher. The mean flux value and the mean depositional amount of Group DM were increased significantly compared to those of Group CTL, whereas the amount of DA penetrating was of no difference. Microdialysis indicated that there was no significant difference between Group CTL and Group DM for all the pharmacokinetic parameters of DA. In contrast, the subcutaneous AUCall values and the Cmax of DSP were significantly increased compared to the control. In conclusion, diabetic rat skin significantly increased the percutaneous permeation of DSP but had no effect on that of DA. It suggests that patients with diabetes should consider the dose of administration when using DA, DSP or other glucocorticoids topically, as different liposolubilities may play some role in the permeability of these compounds via diabetic skin.
Introduction
Diabetes mellitus (DM) is the most common endocrine disorder, which is characterized by high blood glucose levels, disturbances of carbohydrate and lipid metabolisms, and resultant long-term systemic complications. The International Diabetes Federation estimated that about 285 million adults around the world suffered from DM in 2010 and at least one-third of them underwent some type of skin manifestation during the course of their disease (Behm et al., Citation2012). The cutaneous manifestations of DM can be classified into four categories: skin diseases associated with diabetes, bacterial and fungal infections, skin disorders due to diabetic complications and skin reactions to diabetic treatment (Romano et al., Citation1998). It seems that diabetes, similar to some other endocrine disorders, may cause some alterations in functional and mechanical properties of the skin, which derived from an impaired skin homeostasis caused by diabetes-induced abnormalities of the metabolism or by diabetic complications such as vasculopathy and neuropathy.
The skin is composed of three layers, the epidermis, dermis and subcutaneous tissue. The stratum corneum (SC), the outermost layers, is the key rate limitation to percutaneous delivery. It is widely accepted that the hydration state of the SC contributes largely to its barrier function. Sakai et al. reported that the SC hydration and the activity of sebaceous glands decreased obviously in diabetics (Sakai et al., Citation2005). There were also studies that indicated hyperglycemia and decreased insulin signal were involved in the impairment of skin function (Sakai et al., Citation2003; Seirafi et al., Citation2008). In the presence of high glucose (20 mmol/l), the keratinocytes was larger, flattened and had lost some of their orientation toward each other, and the keratinocytes proliferation was decreased. Moreover, the exposure of keratinocytes to high glucose conditions could lead to an inhibition of the proliferation and autophosphorylation of insulin-like growth factor. Therefore, the skin cells proliferation–differentiation balance could be altered by high glucose (Spravchikov et al., Citation2001), which might affect the functions of the SC (Seirafi et al., Citation2008). Besides, microcirculation and dysmetabolism are also involved in the diabetic skin damage. Pharmacokinetics parameters of many drugs were altered in diabetes due to gastrointestinal lesions, which caused changes in drug absorption (Kang et al., Citation2010); changes in liver enzymes which altered drug metabolic rate (Lee et al., Citation2009); and nephropathy which led to changes in drug transport, metabolism and elimination (Bae et al., Citation2005). Percutaneous absorption in some diseased skin was reported (Chiang et al., Citation2012). For example, the cutaneous penetration of a metronidazole cream formulation in the atopic dermatitis or irritant dermatitis was significantly improved in comparison with that in uninvolved skin (Ortiz et al., Citation2008,Citation2009). However, the percutaneous penetration of ketoconazole in gydrocortisone-induced rats group was highly decreased than that in control group (Bi-ya et al., Citation2012).
It has been well known that the skin lesions were found in a large, unselected diabetic population. In type I diabetes patients, vitiligo, posoriasis and eczema are the most common skin disease, whose high frequency can be explained by the common immunological background with this type of diabetes (Romano et al., Citation1998). In the chronic disease, the symptomatic treatment of diabetic skin complications thus requires long-term local application of glucocorticoids. Among the numerous existing artificial glucocorticoids, dexamethasone is most commonly used, mainly in esterified forms such as acetate (DA) or phosphate (DSP) made as external preparation in the market. However, glucocorticoids could increase blood glucose level and inhibit immunoreactions, when administered inappropriately in diabetic patients. They may aggravate complications, increase toxic and side effects, and attenuate therapeutic effects. It was confirmed that insulin secretion could also be inhibited by glucocorticoid (Lambillotte et al., Citation1997; Ullrich et al., Citation2005). In this regard, people suffering from diabetes should be avoided using glucocorticoids theoretically. Nevertheless, many diabetic patients still use glucocorticoids frequently, especially the local application of glucocorticoids due to the high incidence (25∼30%) of dermatoses, one major diabetes complication (Ahmed & Goldstein, Citation2006). Therefore, how to make the local application of glucocorticoids more reasonably to diabetics was elicited from the above-mentioned situations.
Streptozotocin (STZ) is well known to destroy the insulin-secreting pancreatic β cells and STZ-treated animals are widely used as a model of type I diabetes that exhibits many of the features seen in human patients with uncontrolled DM, including hyperglycemia, polydipsia and polyuria, and is characterized by severe loss in body weight (Cheta, Citation1998, Sakai et al., Citation2003, Tunali & Yanardag, Citation2013). Hence, the STZ-induced animals can be used to evaluate the skin functional properties of the diabetes patients to some extent.
In this study, the cutaneous penetration differences of DA and DSP between STZ-induced diabetic rats and healthy rats were evaluated, and the histopathological changes of diabetic skin were determined to elucidate its effect on the drugs permeation and absorption. This study is for the first time in the field of diabetes research, which will provide basis for the rational use of glucocorticoids on diabetics in clinic and the development of percutaneous drugs for diabetics.
Materials and methods
Materials
DA and DSP raw medicine were purchased from Tianjin Tianyao Drug Industry Co. Ltd. (Tianjin, China). DA, DSP and STZ were purchased from Sigma (St. Louis, MO). All other chemicals and solvents were analytical grade.
Animals
All experiments were carried out using adult male Wistar rats (200–250 g), purchased from the Center of Experimental Animals, Southern Medical University (Guangzhou, China). Animals were caged in groups with free access to water and food and kept at 24 °C on a 12-h light/dark cycle during the study period. All animal experiments were performed according to the guidelines of the Experimental Animal Ethics Committee of Southern Medical University.
Induction of diabetes
Adult male Wistar rats were randomly assigned into two groups: control group (Group CTL) and diabetes mellitus group (Group DM). DM models were prepared by a single intraperitoneal injection of 55 mg/kg STZ dissolved in ice cold citrate buffer, containing 100 mM citric acid and 100 mM sodium citrate at pH 4.5. In the following 24 h, to avoid hypoglycemia induced by massive destruction of beta cells and release of intracellular insulin associated with STZ treatment, glycosylated serum was fed to the animals. Rats in the control group were injected intraperitoneally with the same amount of the solvent. All the animals were kept for 30 days before the experiments. During this period, fasting blood glucose levels were measured from the tail vein, using a ONE TOUCH glucometer (Johnson & Johnson Company, New Brunswick, NJ) to confirm diabetes. Animals with blood glucose >250 mg/dl were considered diabetics; animals with blood glucose ≤250 mg/dl were excluded from the study (Arun et al., Citation2005; Chandak et al., Citation2009; Wodarski et al., Citation2009).
Preparation and quality examination of DA and DSP creams
Formulations for two creams
The formulations used for the preparation of Dexamethasone Acetate Cream (DA Cream) and Dexamethansone Sodium Phosphate Cream (DSP Cream) are shown in .
Table 1. Formulations of two creams.
Preparation
Creams were prepared using a standard reverse-emulsification method, using an oil phase and an aqueous phase. Briefly, the oil phases contained Dexamethasone Acetate (added only in the preparation of DA Cream), stearic acid, glyceryl monostearate, liquid paraffin and white vaseline, while the water phase was consisted of Dexamethansone Sodium Phosphate (added only in the preparation of DSP Cream), glycerol, sodium dodecylsulfate, triethanolamine and distilled water. Both phases were heated to 80 °C in a water bath, respectively. The water phase was then infunded into the oily phase expeditiously, while the mixture was being stirred, cooling to room temperature, and thus formed a homogenous cream.
Quality examination
To measure the content of DA (or DSP), 0.2000 g Cream was exactly weighed and dissolved in 50 ml methanol (or distilled water), the DA (or DSP) was extracted by homogenization for 60 s using a D-79219 homogenizer (GMBH&CO.KG, Staufen, Germany) and then the solution was placed into ice bath for 1 h. After that, the solution was filtered twice and analyzed by HPLC.
The actual content of each drug was calculated using the following equation: C = Ct × Vt/Ms, where Ct is the drug concentration in the solution determined by HPLC, Vt is the volume of the drug solution, Ms is the weight of the cream.
In vitro skin permeation studies
Preparation of rat skin
Eighty male Wistar rats (Group CTL (DA) = 20, Group DM (DA) = 20; Group CTL (DSP) = 20, Group DM (DSP) = 20) were used in this study. After the animals were anesthetized with ether and euthanized, the abdominal hair was removed and a circular piece of abdominal skin was excised from the animal immediately, then the subcutaneous fat was carefully removed and the skin integrity was examined after being washed.
Transdermal permeability experiment
In vitro release studies were carried out using TK-12B improved Franz diffusion cells with a receptor compartment volume of 7 ml and an effective area of 2.92 cm2 (Shanghai Kai Kai Technology Co., Ltd.). The freshly excised full-thickness rat skins, which were used as the permeation membrane, were clamped between donor and receiver chambers of the Franz diffusion cell with the SC side facing to the donor chamber and the dermal side facing to the receptor chamber. Isotonic phosphate buffer (pH 7.2) with 0.5% w/v of polyoxyethylene 20 cetyl ether and 0.01% w/v of thiomersal (Lopez et al., Citation2000) was poured into the receptor chamber used as the receptor medium of DA or DSP. The solution in the receptor chambers was stirred continuously at 300 rpm and the assembled apparatus was set at 37 ± 1 °C in the water bath. The respective formulation (DA Cream 2 g, DSP Cream 2 g) was gently placed on the skin surface in the donor compartment. After 1 ml samples were withdrawn from the receiver compartment at regular intervals (0.5, 1, 2, 4, 6, 8, 12 and 24 h), an equal volume of fresh buffer was immediately added. The collected samples were then filtered through 0.45 membrane filters and analyzed by HPLC.
Extraction of drug from the whole skin
To determine the amount of DA or DSP deposited in the skin, after the transdermal permeability experiment, the whole skin was carefully removed from the cells and washed with 5 ml distilled water three times. The treated skin was then clipped into fragments and placed in a 5 ml vial containing 5 ml of methanol. The drug in the skin was then extracted by homogenization and vortexing at 5 min intervals. Finally, the tissue samples were centrifuged at 10 000 rpm for 30 min. The supernatants were collected and analyzed by HPLC.
Microdialysis experiment
In vivo studies
Twenty-four male Wistar rats weighting about 200 g [Group CTL (DA) = 6, Group DM (DA) = 6; Group CTL (DSP) = 6, Group DM (DSP) = 6] were anesthetized with pentobarbital sodium (35 mg/kg), supplementary intraperitoneal injections were given as half the dose every 90 min if needed. For intra-dermal microdialysis, the abdominal fur of the rats was carefully shaved, and then the skin was incised over the dermis. The linear probe (CMA 30 Liner Microdialysis Probe, OD 240 µm, the length of active dialyzer was 10 mm, the cut-off molecular weight was 6 kD) was then implanted into the rat abdominal dermis, introduced through guide cannulae which was subsequently withdrawn. After placement, the probe was perfused with physiological saline using a syringe pump (CMA 100, Stockholm, Sweden) at a constant flow rate of 0.5 μl/min for about 2 h, which was found to be the optimal time period to minimize the needle-induced trauma for a postsurgical stabilization of the dialysate levels (Simonsen et al., Citation2004), DA or DSP cream (10 g/kg) was applied to the abdominal skin of the rat where the linear probe was placed with an area of 2.92 cm2. The intra-dermal dialysate of 30 µl was collected every hour for LC-MS or HPLC analysis. A total of 12 samples were collected from each rat. Throughout the whole experiment, body temperature of the animal was maintained at 37 °C by a heating pad.
In vivo recovery of microdialysis
To obtain absolute tissue concentrations from dialysate concentration, in vivo was carried out using retrodialysis prior to the microdialysis studies. The principle relies on diffusion being quantitatively equal in both directions through the membrane (Stahle et al., Citation1991). The microdialysis probes were implanted into the rat abdominal dermis as described above, and perfused with 100 ng/ml DA normal saline or 100 μg/ml DSP normal saline at a constant flow rate of 0.5 µl/min. The respective dialysates were collected every one hour for seven hours, and the initial two samples were discarded as the equilibration for probe. The inlet (Cin) and outlet (Cout) concentrations of DA were determined by LC-MS, while that of DSP was determined by HPLC. The in vivo recovery (Rin vivo) was then calculated by the following equation: Rin vivo = 1− (Cout/Cin) (Hsiao et al., Citation1990; Huang et al., Citation1999; Tsai & Liang, Citation2001). Rin vivo of each drug was determined from 10 rats of Group CTR (n = 5) and Group DM (n = 5) all over the in vivo experiment.
Analytical methods
HPLC
The quantification of DA and DSP in the transdermal permeability experiment and DSP in the microdialysis experiment was determined by a Shimadzu LC-20 A (Japan) reverse-phase high-performance liquid chromatography (HPLC) (Shimadzu, Kyoto, Japan), using a Waters XTerra MS C18 column (150 mm × 4.6 mm, 5 μm) (Waters, Milford, MA). A mixture of methanol and distilled water (65:35; v/v) was used as the mobile phase for DA. The filtered mobile phase was pumped at a flow rate of 1.0 ml/min, and the wavelength was set at 243 nm. On the other side, the mobile phase for DSP assay was a mixture of potassium dihydrogen phosphate buffer (0.34%, pH = 3.0) and methanol (40:60, v/v). The filtered mobile phase was pumped at a flow rate of 1.0 ml/min, and the wavelength was set at 245 nm. All the measurements were performed at 30 °C.
LC-MS
The quantity of DA was too little to reach the detectability of HPLC, thus the electrospray ionization-liquid chromatography/mass spectrometry (ESI-LC/MS) was introduced to determine the DA content in the dialysates of the microdialysis experiment. The system was equipped with an Agilent 6410A triple quadrupole LC–MS system (Agilent Corporation, MA), and DA was separated by an Agilent ZORBAX SB- C18 column (4.6 mm × 50 mm, 1.8 μm) with an isocratic mobile phase mixture of methanol–distilled water containing 0.1% formic acid (80:20, v/v) at a flow rate of 0.4 ml/min. All the determinations were performed at 25 °C.
Histopathology
Certain sections obtained from healthy rats and DM rats were stained with hematoxylin for cutaneous histomorphological observation. OLYMPUS BX51M light microscopy was used to determine possible anatomical changes in DM rats.
Octanol/water partition coefficient
The octanol/water partition coefficient was an import factor of drug affecting its skin penetration. The Nernst distribution law was applicable in this experiment, a small amount of drug was dissolved in one phase (DA was dissolved in n-octyl alcohol; DSP was dissolved in water) saturated by the other phase in the system prior to the preparation of DA or DSP solution. And then 0.5 ml drug solution was put into a centrifuge tube with 5 ml of the other phase saturated by the solvent. Equilibrium partition was obtained by pendulating for 24 h in the SPX-100B-D shake cultivation device (Shanghai Bo Xun Industrial Co., Ltd. Medical Equipment Factory) where the temperature was maintained at 37 ± 1 °C. After that the two phases were separated and analyzed by HPLC, respectively.
Statistical analysis
All data were presented as mean ± standard deviation (mean ± SD) where mean was from at least three independent measurements. The statistical differences were tested by one independent samples t test. p < 0.05 was considered as significant.
Results
General features of the diabetes rats
In this study, type Ι insulin-dependent DM was induced in rats by STZ according to the method mentioned above. Thirty days after STZ treatment, rats showed a significant higher level of plasma glucose concentration and a significant lower level of body weight (), indicating that the DM animal model was induced successfully.
Table 2. General features of STZ-induced diabetic rats.
The content of two creams
The content of dexamethasone acetate in DA cream and dexamethasone sodium phosphate in DSP cream were detected to be 2.0600% ± 2.65% (n = 3) and 2.0633% ± 2.52% (n = 3), respectively, which were 103.00% (n = 3) and 103.17% (n = 3) of their labeled amount. These results were consistent with the prescript of Pharmacopoeia of People’s Republic of China (PPRC).
In vitro permeation study
Transdermal permeability experiment
The cumulative amount of each drug was calculated as the equation: Q = [Cn × V + ΣCi × 1]/A, where Q is the cumulative drug amount, Cn and Ci are the drug concentration in the receiver compartment at n and i (i ≤ n-1) sampling point, respectively, V is the capacity of receiver compartment, A is the actual surface diffusion area. The cumulative permeation amount of DA in DM rat was almost the same as the control, but for DSP, the cumulative amount penetrated the skin of DM rats was increased compared with that of the control (). To have a better comparison between the permeation measurements of different skin, the slopes (flux) of the linear portion of the in vitro permeation profiles were calculated, as was the steady state flux (Jss), based on the cumulative amount of drug permeated per unit area plotted against time (). The permeation rate of DA (0.7801 ± 0.4480 μg/h/cm2) was almost not changed in DM rats compared to that of the control (0.7947 ± 0.3630 μg/h/cm2), while for DSP, the permeation rate in DM rats (68.3435 ± 15.1866 μg/h/cm2) was significantly higher than that of the control (42.6156 ± 10.6145 μg/h/cm2) (p < 0.01) ().
Figure 1. Transdermal permeability experiment of the two drugs in DM group and control group. A) Data of DA, B) Data of DSP.
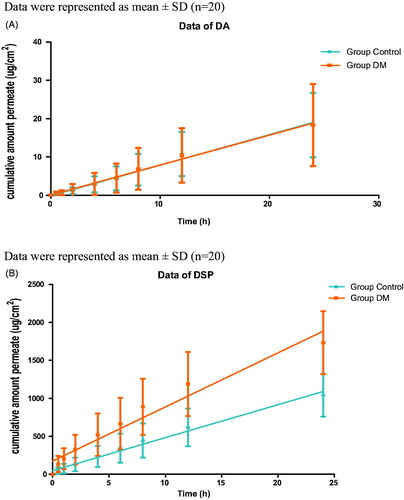
Table 3. Flus of DA and DSP through the rats’ skin.
Extraction of each drug from whole skin
As shown in , there was a significant difference of depositional amounts of DSP at 24 h between DM rats and the control (83.0609 ± 30.0689 μg/g versus 36.0671 ± 13.3097 μg/g). But those of DA at 24 h have no difference between DM rats and the control (2.5133 ± 1.7766 μg/g versus 2.5451 ± 1.4717 μg/g).
Table 4. Depositional amounts of DA and DSP remained in whole skin.
Microdialysis experiment
In vivo recovery
In vivo recovery of microdialysis probe for DA and DSP in DM rats and control was presented in . The real concentration of drugs in the probe-implanted location (abdominal dermis) was calculated as the equation: C = C0/R, where C0 was the drug concentration of the collected samples, C was the real concentration of drug in the subcutaneous interstitial fluid, and R was the in vivo recovery of the probe.
Table 5. In vivo microdialysis recovery (%) in different position of rats.
In vivo studies
The corrected concentrations of DA and DSP in subcutaneous liquid after their topical applications (10 g/kg) on DM or healthy rats over 12 hours were shown in . The pharmacokinetic parameters of DA and DSP in Group CTL and Group DM were calculated by Phoenix WinNonlin 2.0 (). Statistical analysis indicated that there was no significant difference between Group CTL and Group DM for all the pharmacokinetic parameters of DA. Furthermore, the tmax (time to maximum concentration) of DSP between the two groups was also identical. However, the area under the curve (AUCall) and the Cmax (maximum concentration) of DSP in DM group were significantly increased in comparison with those in CTL group.
Figure 2. DA and DSP concentration in subcutaneous liquid by in vivo microdialysis for DA cream (2%) and DSP cream (2%). A) Data of DA, B) Data of DSP.
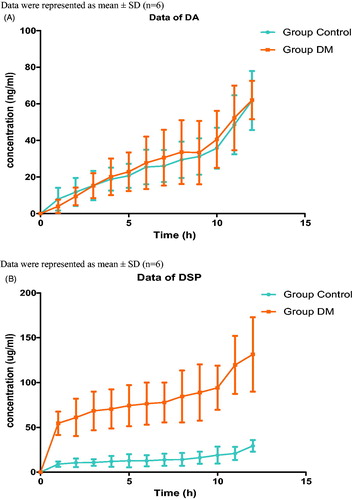
Table 6. Pharmacokinetic parameters of drugs.
Changes of the abdominal skin
The epidermal tissue of DM diabetes was much thinner, and the epidermal cell layer was less clear than those of the control group. Furthermore, the stratified arrangement of epidermal cells almost disappeared and progressive atrophy was developed on the subcutaneous fat compared to those of the control group ().
Evaluation of octanol/water partition coefficient
The octanol/water partition coefficients of DA and DSP were calculated according to the following equation: Papp = (ρv–ρwvw)/ρwvw, where Papp was the octanol/water partition coefficients, ρ was the original concentration of drugs in one phase, v was the volume of one phase, ρw was the drug concentration detected in the other phase, vw was the volume of the other phase. The octanol/water partition coefficients of DA and DSP were found to be 391.44 ± 61.38 (n = 5) and 0.76 ± 0.19 (n = 5), respectively.
Discussion
Almost all the diabetics suffer from skin damage if the microcirculation dysmetabolism and its effect on skin collagen protein are considered together. In rats with diabetes, continuous hyperglycemia may induce the alteration of spatial structure, and change the microenvironment properties which are important to cell function and survival. The alteration of microenvironment has been characterized by infiltration of inflammatory cell to different extent in the dermis of rats, demonstrating that the diabetic skin tissue is in a pathological state even in the absence of exogenous trauma, which suggests that after local application of glucocorticoids, there might be some possible differences between the diabetic skin and normal skin in their transdermal permeability and absorption. Thus, this study was undertaken to investigate if pathological changes of diabetes skin would affect percutaneous penetration and absorption of glucocorticoids compared to that of normal skin, with the expectation to provide important preclinical data for clinical tests or rational drug administration.
It is well established that the quantity of a compound recovered by microdialysis is only a fraction of that present in the tissue (Leveque et al., Citation2004). The ratio of the concentration of analyte in microdialysate samples relative to the medium surrounding the probe is defined as recovery (Elmquist & Sawchuk, Citation1997; Sasongko et al., Citation2000). The actual concentration of drug in the probe-implanted location is demanded to calculate the recovery. Both in vivo and in vitro methods could be adopted to estimate probe efficiency, but in vivo recovery is more credible (Weiss & Lunte, Citation2000), because probe-implanted location may more accurately reflect the real physiological conditions. Thus, in vivo retrodialysis was used in this study.
DA and DSP creams made in the laboratory were provided as the model drugs in two groups (control group and DM group). Those two creams were prepared in parallel, and the blank matrix materials and preparative methods of the cream were selected according to the regulation of PPRC.
In vitro permeation experiments showed that the cumulative amount, the permeation rate and depositional amount of DSP in DM group were much higher compared to those in the control group, which may be due to the reduced thickness of the diabetic abdominal dermis with morphological characteristics of obscured multilayer epithelium and shortened, thinned and disorganized collagen fibrils (Niu et al., Citation2012). However, there was no difference for DA in the skin of DM rats and the control. That may be because of the different solubility and oil-water partition coefficient of DA and DSP. The high lipophilicity of DA prevented drug diffusion from the skin into the receiver fluid.
The deposition amount of DSP was more than two much larger in DM group compared to that in the control, which may be related to the abnormal proliferation and differentiation of keratinocytes in the epidermis (Seirafi et al., Citation2008) and the altered hydration state of patients with diabetes. The increased proliferation of keratinocytes may accumulate the drug detained in corneum, preventing it to be absorbed further. However, there was no difference of DA deposition amount in DM group and the control, because the hydration state did not affect the highly lipophilic drug.
The results of microdialysis experiment were highly in accordance with the in vitro permeation experiment. Corrected dialysate concentrations at each time point after topical application of DA in two groups (Group CTL and Group DM) were consistent with each other; however, those of DSP in DM group were obviously higher than those in the control group ().
In recent years, with the development and application of microdialysis, implanted linear probes into the dermis were widely used to determine the percutaneous permeation of drugs (Graumlich et al., Citation2000; Simonsen et al., Citation2004). In this study, both of the classical and the new-type method were applied to evaluate the percutaneous permeability and absorption of dexamethasone with different liposolubilities. Those two methods showed a good correlation. The experiments demonstrated that the changes of diabetic skin had no effect on the transdermal penetration of DA but increased the amount of DSP penetrating through the skin significantly both in vitro and in vivo, thus the consequences were more comprehensive and more factual.
Histopathological examination showed that the structure of the control rat skin had normal skin layers, while the thickness of the dermal layers was reduced and presented an abnormal histomorphological structure in diabetic rats. In addition, the epidermal cell layer was less clear, the stratified arrangement of epidermal cell had almost disappeared and progressive atrophy was developed in the subcutaneous fat. A possible explanation is that the accumulation of advanced glycosylation end products (AGEs) in diabetic skin tissues induced an oxidative damage of the fibroblasts and acted as an important contributor to the disorganized collagen fibers in the thinner diabetic abdominal dermis (Niu et al., Citation2012). Furthermore, the increased level of malondialdehyde (MDA) in diabetic rats promoted proteolytic degradation of damaged tissue. This was consistent with the collagen feature in the diabetic dermis, which manifested as shortened, thinned and disorganized fibers (Niu et al., Citation2012).
As it is well known, the solubility and oil-water partition coefficient of a drug would directly affect its percutaneous absorption. Generally speaking, oil-soluble drugs have higher ability to penetrate the skin than water-soluble drugs, but actually, the drugs which can dissolve in both oil and water have even higher penetrability. The drugs with highest penetrability has almost the same solubility in both oil and water solution. The high oil-soluble drugs may be accumulated and detained in corneum, so it is hard for them to be absorbed further. Thus, one of the reasons causing above results might be related to the different solubilities and oil-water partition coefficient of DA and DSP. The results indicated that the oil-water partition coefficients of DA and DSP were 391.3079 ± 38.3824 (n = 5) and 0.7563 ± 0.2606 (n = 5), respectively. With great lipid solubility, DA may be accumulated and detained in corneum, so it is hard for it to be absorbed further and the changes of diabetic skin could not increase the amount of DA penetrating though the diabetic skin.
It is known that the transfer of drugs contained in transdermal drug delivery systems through the skin includes two main steps: 1) the diffusion of drugs from matrix to skin surface; 2) the permeation of drugs through the skin. It is speculated that the main limiting step of DA is the drug diffusion from matrix to skin surface, while the main limiting step of DSP is its permeation through the skin. So the pathological changes in horny layer and cuticular layer of the diabetic skin only had a significant effect on the permeation process of DSP but not DA. Thus, a similar situation could be expected when diabetic patients use DA and DSP creams. Therefore, it may be necessary for patients with diabetes to consider the dose of administration when they need to use DA, DSP or other glucocorticoids topically.
In conclusion, the changes of diabetic skin had no effect on the transdermal penetration of DA, a highly liposoluble glucocorticoid, but significantly increased the permeation amount of DSP, a water-soluble glucocorticoid. As a result, when these two drugs are applied to diabetics, the dose of DSP cream should be decreased whereas that of DA cream should be the same as normal. The results from this study provided a reference for the topical application of glucocorticoids to diabetic patients, and an experimental evidence for medical pharmacist’s consultation aiming at using glucocorticoids more reasonably.
Declaration of interest
The authors declare no conflicts of interest.
This work was supported by the Grants from NSFC (No. 81173014 for Li GF) and Science and Technology Planning Project of Guangdong Province(No. 2010B0370700014 for Li GF) as well as Natural Science Foundation of Guangdong Province, China (Grant No.:9151008901000223).
Acknowledgements
The authors would like to thank Professor Zeng Kang, the chief of dermatology in Nanfang Hospital, Guangzhou, China, for his suggestion of the application of glucocorticoids on the diabetics and the technicians of the Endocrinology Department in Nanfang Hospital, Guangzhou, China, for their assistance with the animal model and surgeries.
References
- Ahmed I, Goldstein B. (2006). Diabetes mellitus. Clin Dermatol 24:237–46
- Arun KHS, Kaul CL, Ramarao P. (2005). AT1 receptors and L-type calcium channels: functional coupling in supersensitivity to angiotensin II in diabetic rats. Cardiovasc Research 65:374–86
- Bae SK, Yang SH, Lee SJ, et al. (2005). Pharmacokinetic changes of DA-7867, a new oxazolidinone, after intravenous and oral administration to rats with short-term and long-term diabetes mellitus induced by streptozotocin. Eur J Pharm Sci 25:337–45
- Behm B, Schreml S, Landthaler M, Babilas P. (2012). Skin signs in diabetes mellitus. J Eur Acad Dermatol Venereol 26:1203–11
- Bi-Ya S, Guo-Feng L, Lu-Qi X, Bo·Xin Z. (2012). Efect of hydr0c0rtis0ne on the percutaneous penetration of ketoconazole via rat skin. Chinese Pharmacological Bullet 28:709–15
- Chandak PG, Gaikwad AB, Tikoo K. (2009). Gallotannin ameliorates the development of streptozotocin-induced diabetic nephropathy by preventing the activation of PARP. Phytother Res 23:72–7
- Cheta D. (1998). Animal models of type I (insulin-dependent) diabetes mellitus. J Pediatr Endocrinol Metab 11:11–19
- Chiang A, Tudela E, Maibach HI. (2012). Percutaneous absorption in diseased skin: an overview. J Appl Toxicol 32:537–63
- Elmquist WF, Sawchuk RJ. (1997). Application of microdialysis in pharmacokinetic studies. Pharmaceut Res 14:267–88
- Graumlich JF, Mclaughlin RG, Birkhahn D, et al. (2000). Subcutaneous microdialysis in rats correlates with carbamazepine concentrations in plasma and brain. Epilepsy Research 40:25–32
- Hsiao JK, Ball BA, Morrison PF, et al. (1990). Effects of different semipermeable membranes on in vitro and in vivo performance of microdialysis probes. J Neurochem 54:1449–52
- Huang C-T, Chen C-F, Tsai T-H. (1999). Measurement and pharmacokinetic study of unbound tropisetron in rat blood by microdialysis with high-performance liquid chromatography. Int J Pharm 182:237–42
- Kang HE, Sohn SI, Baek SR, et al. (2010). Liquiritigenin pharmacokinetics in a rat model of diabetes mellitus induced by streptozotocin: greater formation of glucuronides in the liver, especially M2, due to increased hepatic uridine 5'-diphosphoglucuronic acid level. Metab Clin Exp 59:1472–80
- Lambillotte C, Gilon P, Henquin J-C. (1997). Direct glucocorticoid inhibition of insulin secretion. An in vitro study of dexamethasone effects in mouse islets. J Clin Invest 99:414–23
- Lee DY, Chung HJ, Choi YH, et al. (2009). Pharmacokinetics of ipriflavone and its two metabolites, M1 and M5, after the intravenous and oral administration of ipriflavone to rat model of diabetes mellitus induced by streptozotocin. Eur J Pharm Sci 38:465–71
- Leveque N, Makki S, Hadgraft J, Humbert P. (2004). Comparison of Franz cells and microdialysis for assessing salicylic acid penetration through human skin. Int J Pharm 269:323–8
- Lopez RFV, Collett JH, Bentley MVRLB. (2000). Influence of cyclodextrin complexation on the in vitro permeation and skin metabolism of dexamethasone. Int J Pharm 200:127–32
- Niu Y, Cao X, Song F, et al. (2012). Reduced dermis thickness and AGE accumulation in diabetic abdominal skin. Int J Low Extrem Wounds 11:224–30
- Ortiz PG, Hansen SH, Shah VP, et al. (2008). The effect of irritant dermatitis on cutaneous bioavailability of a metronidazole formulation, investigated by microdialysis and dermatopharmacokinetic method. Contact Dermatitis 59:23–30
- Ortiz PG, Hansen SH, Shah VP, et al. (2009). Impact of adult atopic dermatitis on topical drug penetration: assessment by cutaneous microdialysis and tape stripping. Acta Derm Venereol 89:33–8
- Romano G, Moretti G, Benedetto AD, et al. (1998). Skin lesions in diabetes mellitus prevalence and clinical correlations. Diabetes Res Clin Pr 39:101–6
- Sakai S, Endo Y, Ozawa N, et al. (2003). Characteristics of the epidermis and stratum corneum of hairless mice with experimentally induced diabetes mellitus. J Invest Dermatol 120:79–85
- Sakai S, Kikuchi K, Satoh J, et al. (2005). Functional properties of the stratum corneum in patients with diabetes mellitus: similarities to senile xerosis. Br J Dermatol 153:319–23
- Sasongko L, Williams KM, Ramzan I, Mclachlan AJ. (2000). Assessment of in vitro and in vivo recovery of gallamine using microdialysis. J Pharmacol Toxicol Methods 44:519–25
- Seirafi H, Farsinejad K, Firooz A, et al. (2008). Biophysical characteristics of skin in diabetes: a controlled study. J Eur Acad Dermatol Venereol 23:146–9
- Simonsen L, Jorgensen A, Benfeldt E, Groth L. (2004). Differentiated in vivo skin penetration of salicylic compounds in hairless rats measured by cutaneous microdialysis. Eur J Pharm Sci 21:379–88
- Spravchikov N, Sizyakov G, Gartsbein M, et al. (2001). Glucose effects on skin keratinocytes_ implications for diabetes skin complications. Diabetes 50:1627–35
- Stahle L, Segersvard S, Ungerstedt U. (1991). A comparison between three methods for estimation of extracellular concentrations of exogenous and endogenous compounds by microdialysis. J Pharmacol Methods 25:41–52
- Tsai T-H, Liang C-C. (2001). Pharmacokinetics of tetramethylpyrazine in rat blood and brain using microdialysis. Int J Pharm 216:61–6
- Tunali S, Yanardag R. (2013). Protective effect of vanadyl sulfate on skin injury in streptozotocin-induced diabetic rats. Hum Exp Toxicol (In Press). doi: 10.1177/0960327112478445
- Ullrich S, Berchtold S, Ranta F, et al. (2005). Serum- and glucocorticoid-inducible kinase 1 (SGK1) mediates glucocorticoid-induced inhibition of insulin secretion. Diabetes 54:1090–9
- Weiss DJ, Lunte CE. (2000). In vivo microdialysis as a tool for monitoring pharmacokinetics. TRAC-Trend Anal Chem 19:606–16
- Wodarski R, Clark AK, Grist J, et al. (2009). Gabapentin reverses microglial activation in the spinal cord of streptozotocin-induced diabetic rats. Eur J Pain 13:807–11