Abstract
Context: The first successful molecule against herpes infections was Acyclovir, which competes with new generations in the market, with its potential activity. The major physicochemical constraints and pharmacokinetics of Acyclovir such as low solubility, poor permeability, less half-life, high dose has initiated many researchers to develop diverse modified release dosage forms.
Objective: The objective of this work was to design polymeric nanoparticles of Acyclovir and then incorporate the drug-loaded nanoparticles within an in situ gelling system to provide dual sustained release effect, whereby the duration of action and bioavailability through different routes of administration could be improved.
Materials and methods: The formulation was designed through 32 factorial design, first developing the nanoparticles using Polycaprolactone and Pluronic F127 by Solvent evaporation process, followed by dispersion of the suspended nanoparticles into thermosensitive in situ gelling system of Pluronic F127 with Carbopol.
Results and discussion: The characterization of the nanoparticles and its sol-gel system performed through zeta sizer, SEM, XRD, TG-DSC, FTIR and rheology helped to optimize the formulation. The drug release could be sustained to 60% and 30% at eight hours, for the nanoparticles and their in situ gel systems, respectively, with non-Fickian diffusion mechanism of drug release. The test for % cell viability with NIH3T3 cell line revealed low level of toxicity for the nanoparticles.
Conclusion: The statistical significance obtained for the trail formulations experimentally proved its suitability for this dosage form design to achieve desired level of drug release.
Introduction
Acyclovir (ACV), an analogue of purine nucleoside has been identified with potential antiviral mechanism through competitive inhibition of the viral DNA especially for the Herpes Simplex virus and in addition Varicella Zoster, Epstein-Barr and Cytomegalo virus infections. While various new antiviral molecules are emerging, yet Acyclovir stands in the market due to its significant suppression of recurrent infections, negligible drug interactions and the bioclinical safety data with carrying women and baby. Also, the price of molecule is comparatively cheaper and also the product exists in different dosage forms starting from conventional marketed dosage forms like single unit solid dosage form, liquid orals, topical semisolids and injections, and extending to various researches on novel drug delivery systems such as modified release tablets, microspheres, nanoparticles, sol-gel systems, implants, emulsified systems, transdermal films, vesicular dosage forms, etc., each having their specific purpose and targeting need in different routes.
Even though the molecule is more stable for its active application, certain parameters of this drug namely, slight water solubility (1.3 mg/mL at 25 °C), poor permeability (0.12 × 10−6 to 2.0 × 10−6 cm/s), high dose and dosing frequency (200 to 800 mg, 5 times a day), less half-life (2.5–3.3 h) and low oral bioavailability (10–20%) challenge the design of modified dosage forms to achieve 100% success in the therapy (Susantakumar et al., Citation2011).
Nanoparticles of the antiviral drugs could enhance their activity and therapy by improving the effective surface area which aid in prolonged release (Roberta et al., Citation2009). And likewise, in situ gelling system may improve the sustained release action of the dispersed drug, by sol to gel transition mechanism due to temperature, pH or ionization depending on the gelling agent involved in the formulation (Nirmal et al., 2010). The success of the twin delivery system by the combination of drug nanoparticles incorporated into in situ gels has been identified for drugs like Forskolin (Saurabh et al., Citation2010), Glibenclamide (Gohel et al., Citation2009) and Insulin (Song et al., Citation2011), whereby, an improvement in sustained release effect and bioavailability has been reported.
In this study, Acyclovir nanoparticulate in situ gelling system was prepared by developing polymeric nanoparticles of the drug first and then, dispersing the nanosuspension containing both polymer-entrapped and unentrapped drug into the thermoresponsive gelling system (). Pluronic is thermosensitive polymer which exists in the form of sol at below room temperature and undergoes gelation at body temperature (Jeong et al., Citation2002). The PEO-PPO-PEO tri-block copolymer chain present in Pluronic is amphiphilic in nature, which forms micelle like aggregates above its critical micelle concentration (Alain et al., Citation1997). The mechanism behind the sol-gel transition is the micelle packing in lattice undergoing liquid hard sphere crystallization, making a stiff and less fluid gel (Lee et al., Citation2010). The polymeric nanoparticles are entangled between the micelle aggregates of the gel to provide dual barrier so that more sustained release could be achieved, whereas the polymer unentrapped drug can provide the initial drug release. Because both polymer entrapped and the unentrapped drug nanoparticles are used in this system, there is no loss of drug during the formulation design. The addition of Carbopol with Pluronic can enhance the gelling capacity and bioadhesive property of the system, so prolonged action can be achieved. The mechanism of drug release involves combination of dissolution of the micelle and diffusion of drug through the polymeric nanoparticles (Ricci et al., Citation2005). The significance and novelty of this dual delivery system for Acyclovir over the other delivery system are its stability and possible administration of the same dosage form through many routes like oral, topical, nasal, ocular, rectal, vaginal, parenteral, etc.
Materials
Acyclovir was obtained as gift sample from Matrix India Pvt. Ltd., Hyderabad, India. Poly Capro Lactone (PCL) and Pluronic® F127 were purchased from Sigma Aldrich Chemicals. Carbopol® 940 was procured from SD fine Chem., Mumbai, India. The excipients, chemicals and reagents used in this formulation and evaluation studies were intended for analytical purpose.
Methodology
32 Factorial design for acyclovir-PCL nanoparticles and in situ gelling system
Response surface methodology was used to predict optimum level of polymer and surfactant and also the interactions among the factors using 32 factorial design as a statistical tool, which work based on the first degree of mathematical model (Devi et al., Citation2009).
As the first step, the low and high limits of the variables were selected from the data provided by literatures. When the possible low and high ranges were fed in the software (Design Expert Version 8, State Ease, Inc. Minneapolis, Minnesota 55413-2726, US), the composition of the nine trial formulations were fixed by the software by taking the medium range as one value for both the variables. The formulations were prepared as per the concentrations obtained from the software, and statistically optimized from the experimental work results.
With this tool, the design was developed for the nanoparticles selecting two independent variables as polymer PCL (X1) and surfactant Pluronic F127 (X2) levels (concentrations), for the dependant variable responses of size (Y1) and drug entrapment efficiency (Y2). The three levels of these independent variables were selected as low (−1), medium (0) and high (+1), and according to the design, the formulations were prepared and evaluated by changing the concentration as shown in the . The results obtained were subjected for statistical analysis using ANOVA. To obtain optimal formulation, surface response methodology and multiple regression analysis by quadratic polynomial equations was used. Similarly, the nanoparticles incorporated in situ gelling system was also optimized by taking two independent variables as percentage of Pluronic (X1) and Carbopol (X2) and the selected responses were gelling temperature (Y1) and percentage drug release (Y2).
Table 1. Factorial design of polymeric nanoparticles.
Nanosuspension preparation
The nanosuspension containing polymeric nanoparticles of Acyclovir with PCL were prepared by the two steps in which a dispersion with organic solvent in water was first made followed by the vaporization of the volatile solvent (Devi et al., Citation2009). The polymer was dissolved in acetone and the drug was added in aqueous surfactant solution, after which the organic phase was dispersed in the aqueous media with the aid of probe sonication (P250 Vibronics, Mumbai, India) at 100 kV for 30 minutes. The colloidal dispersion of polymeric nanoparticles suspension was kept in magnetic stirrer maintained at 40 °C until acetone evaporates completely.
Freeze drying of the nanoparticles
The formulated nanosuspension was centrifuged at 4000 rpm for 10 min to eliminate the micron sized and unentrapped particles. The supernatant solution was again centrifuged using cooling ultracentrifuge (Sigma 3K30, Osterode Am Harz, Germany) at 16 000 rpm for 30 min at −10 °C where the nanoparticles were frozen and collected as pellet. This pellet was redispersed in distilled water using cyclone mixer (Remi CM101 DX, Mumbai, India) to get uniform nanoparticle dispersion which was freeze-dried (Christ Alpha 2-4 LD Plus, Osterode Am Harz, Germany) at −80 °C for 24 h (Abdelwahed et al., Citation2006).
Entrapment efficiency of nanoparticles
The supernatant solution obtained after the ultra centrifugation was suitably diluted and the drug content was estimated with UV-Visible spectrophotometer (Evolution 201, Thermo Scientific, Shanghai, Made in China, Deisgned in USA). The percentage of entrapment efficiency was calculated using the formula (Selvakumar et al., Citation2009),
Characterization of ACV-PCL nanoparticles
The measurement of particle size and its distribution was carried out using dynamic light scattering principle, and the surface charge in terms of zeta potential was measured using charge conductivity principle (Malverm Nano Series ZS, Worcestershire, UK). The exterior surface morphology of the freeze-dried samples were observed using Field Emission Scanning Electron Microscope (JSM 6701F, JEOL, Tokyo, Japan), after they were sputter coated with gold using auto fine coater (JFC 1600, JEOL, Tokyo, Japan). The changes in crystalline properties of the drug and its nanoparticles were evaluated by powder X-ray diffraction analysis. The diffraction patterns of freeze-dried nanoparticles and the pure drug were studied using the X-ray diffractometer (D8 FOCUS, BRUKER, Karlsruhe, USA) with Cu-Kα radiation at 1.5418 Å. The analysis was performed for 2θ values from 10° to 60° with a step size of 0.01°/min. The lyophilized dry samples were subjected to Fourier Transform - Infra Red (FTIR) spectroscopy (Perkin Elmer System 200, Shelton, Connecticut, USA). The spectrum was recorded in the wave number range of 4000–400 cm−1. The change in thermal property of Acyclovir nanoparticles and pure drug were studied using Thermo Gravimetric - Differential Thermal Analysis (TA instrument, Q100, Michigan, USA). Around 2–5 mg of the samples were placed in an aluminum pan and heated at the rate of 10 °C/min up to 1000 °C and the result was represented as weight loss and heat flow against temperature (Cristina et al., Citation2002; Kamel et al., Citation2007).
Formulation of in situ gelling system
Thermosensitive in situ gelling system of Acyclovir nanoparticles were formulated through cold processing technique. The optimized formulation with narrow size distribution and better entrapment of drug in the polymeric nanoparticles was selected for this formulation. In this process, the in situ gelling polymer Pluronic F127 was dissolved in water at 10 °C with continuous stirring and bath sonication and then kept overnight at 5 °C for polymer desolvation (Pravin et al., Citation2010). Carbopol 940 was added to this gel base to optimize the bioadhesive property and stability, followed by the addition of the Acyclovir polymeric nanoparticulate suspension. The colloidal solution was homogenously mixed and the pH was checked.
Evaluation of in situ gelling system
The in situ gelling system containing polymeric nanoparticles of Acyclovir was optimized for its quality, stability and suitability for drug release through certain evaluation parameters such as pH, gelling time, gelling temperature, viscosity, drug content and release rate (Grace Rathnam et al., Citation2012; Sonjoy et al., Citation2012).
The pH of the samples was evaluated using electronic pH meter (pHep, Hanna Instruments, Woonsocket, Rhode Island, USA). The drug content and its uniformity in the system was evaluated by taking 10 mg equivalent of sol containing polymeric nanoparticulate drug and dispersed in distilled water by bath sonication (Khera Instruments Pvt. Ltd., New Delhi, India) and then kept overnight. The solution was suitably diluted to estimate the concentration of drug by spectrophotometric method.
The temperature and time taken for the sol to gel transition (gelation temperature) was also noticed by observing the change in flow ability of the sol, taken in a test tube and heated at 2 °C/min rate. The viscosity of the sol systems was measured by the principle of cup and bob mechanism (Brookfield Viscometer DV-II + Pro Extra, Middleboro, Massachusetts, USA), using spindle no: 63, first at cold temperature followed by the conversion of the sol to gel by gradually increasing the temperature to the transition point and gel viscosity measurement at the respective gelation temperature for each system.
In vitro release studies
The percentage of drug release from the Acyclovir-loaded PCL nanoparticle suspension and the nanoparticle-loaded in situ gelling system were tested at periodic time intervals to estimate and compare their effects on sustained release of the drug. The nanosuspension/in situ gelling sol sample equivalent to 2 mg of the drug was loaded in a cylindrical open-ended tube of which one end was tied with the dialysis membrane (HiMedia, Mumbai, India; Pore size: up to 14 000 Daltons). The tube was placed into the basket of USP type I apparatus (LabIndia, Mumbai, India) and dipped into the dissolution chamber containing 200 mL of distilled water pH 7.0 as media with the bath temperature of 37 ± 0.5 °C. The study was carried at 100 rpm and the samples of 10 mL were withdrawn using a syringe at predetermined time intervals, and replaced with the same level of media to exhibit sink phenomenon (Dhanalekshmi et al., Citation2010; Abdel-Mottaleb et al., Citation2011). The collected samples were analyzed using UV-Visible spectrophotometer for the estimation of content of drug liberated from the polymer encapsulated nanoparticles/nanoparticles dispersed gels. The study was performed in triplicate and presented as the mean with standard deviation limits.
Kinetics of drug release
To understand the kinetics of drug release from Acyclovir-loaded PCL nanoparticles and the nanoparticles-loaded in situ gelling system, the dissolution data acquired from drug release studies were fitted to different mathematical kinetic models such as zero-order, first-order, Higuchi and Hixon models (Suvakanta et al., Citation2010). The Korsmeyer–Peppas model was also applied to analyze the mode of drug release.
Ex-vivo diffusion study
The study was carried out using Franz diffusion cell to check the amount of drug diffused across the transdermal membrane (Guadalupe et al., 2011). The receptor was filled with distilled water as media, till the neck of the chamber (20 ml). Goat skin was collected from the slaughter house and the buccal skin was cut, wherein the hair present on the outer surface and the fatty tissue present on the inner surface were removed. The excised skin was mounted in between donor and the receptor cell. The entire apparatus was kept over magnetic stirrer with a magnetic teflon bead placed in the bottom of the receptor cell. The optimized formulation of the nanoparticle incorporated in situ gel (AIG-7) was placed on the upper surface of skin wherein the lower surface was in contact with the media present in the donor cell. Samples of 5 ml were collected from the receptor cell at periodic time intervals, and replaced with the same volume to maintain sink level. Absorbance of samples was measured at λmax of Acyclovir and the amount of drug diffused through the membrane at each time point was calculated to assess the cumulative drug diffusion.
Cytotoxicity study
The mouse embryonic fibroblasts cell line (NIH 3T3) was obtained from National Centre for Cell Science (NCCS), Pune, and grown in Dulbeccos Modified Eagles Medium containing 10% fetal bovine serum (FBS) and maintained at 37 °C, 5% CO2 and 100% relative humidity. Maintenance cultures were passaged weekly, and the culture medium was changed twice a week. The monolayer cells were detached with trypsin-ethylene diamine tetra acetic acid (EDTA) to make single cell suspensions and viable cells were counted using a hemocytometer and diluted with medium containing 5% FBS to give final density of 1 × 105 cells/mL. One hundred microliters per well of cell suspension were seeded into 96-well plates at plating density of 10 000 cells/well and incubated to allow for cell attachment at 37 °C, 5% CO2, 95% air and 100% relative humidity. After 24 h, the cells were treated with serial concentrations of the test samples. “Pure Drug” was solubilized in alcohol and was diluted in serum-free medium. The samples “Blank Nanoparticles” and “ACV-PCL Nanoparticles” were sterilized by syringe filtration using 0.45 µm filters. Because the formulation contained nanoparticles in the average size range of 100–400 nm, the usage of 0.25 µm filtration lead to separation of the nanoparticles from the dosage form during the process of sterilization, and so 0.45 µm membrane filter was used. As the optimized formulation was freshly prepared and used for the cytotoxicity study, the possibility of microbial contamination was very negligible. An aliquot of all the sample solutions were diluted to twice the desired final maximum test concentration with serum free medium. Additional four serial dilutions were made to provide a total of five sample concentrations. Aliquots of 100 µL of these different sample dilutions were added to the appropriate wells already containing 100 µL of medium, resulting in the required final sample concentrations as 12.5, 25, 50, 100 µg/mL. Following sample addition, the plates were incubated for an additional 48 h at 37 °C, 5% CO2 and 100% relative humidity. The medium containing without samples were served as control and triplicate was maintained for all concentrations (Rejinold et al., Citation2011).
After 48 h of incubation, 15 µL of 3-[4,5-dimethylthiazol-2-yl]2,5-diphenyltetrazolium bromide (MTT) (5 mg/mL) in phosphate buffered saline (PBS) was added to each well and incubated at 37 °C for 4 h. MTT, an yellow color water soluble salt containing tetrazolium ring can be cleaved by the mitochondrial enzyme (succinate-dehydrogenase) in living cells, converting them to an insoluble purple formazan. Therefore, the amount of formazan produced is directly proportional to the number of viable cells. The medium with MTT was then flicked off and the formed formazan crystals were solubilized in 100 µL of DMSO and then the absorbance measured at 570 nm using micro plate reader (Monks et al., Citation1991). The percentage cell viability was then calculated with respect to control as follows:
Results
Factorial design for nanoparticles and in situ gels
The independent variables selected for the nanoparticles were PCL (X1) and Pluronic F127 (X2), and for the in situ gelling system as Pluronic F127 (X1) and Carbopol 940 (X2). Their corresponding responses were particle size (Y1) and entrapment efficiency (Y2), and gelling temperature (Y1) and drug release (Y2) for drug-entrapped nanoparticle and nanoparticle incorporated in situ gel, respectively, the results obtained experimentally are shown in and . Based on the least PRESS (Predicted Residual Sum of Squares) value obtained by statistical method the quadratic model was selected as good fit, which was statistically significant, and the data were analyzed by ANOVA (analysis of variance) and results are shown in and . The suggested response surface model generated by the design formed a polynomial equation as follows,
Table 2. Factorial design of in situ gelling system.
Table 3. ANOVA results for ACV-PCL nanoparticles obtained using 32 factorial design.
Table 4. ANOVA results of nanoparticles incorporated in situ gels obtained using 32 factorial design.
Where β0 was intercept and β1 and β2 were coefficients of respective factors.
Response 1: Size of nanoparticles
The ANOVA results of lack of fit test showed that the probability value p < 0.05 indicates model terms such as X1, X2, X1X2 and X12 are significant. The final equation in terms of coded factors obtained was
Response 2: Entrapment efficiency of nanoparticles
The ANOVA results of lack of fit test showed that the probability value p < 0.05 indicates model terms such as X1, X2, X12 and X22 are significant. The final equation in terms of coded factors obtained was
When the same 32 factorial design was used to optimize the in situ gel formulations, the following response equation was obtained.
Response 1: Gelling temperature
The ANOVA results of lack of fit test showed that the probability value p < 0.05 indicates model terms such as X1, X2, X22 are significant. The final equation in terms of coded factors obtained was
Response 2: Drug release
The ANOVA results of lack of fit test showed that the probability value p < 0.05 indicates model terms such as X1, X2, X22 are significant. The final equation in terms of coded factors obtained was
The contour plot and 3D response surface images of the optimization scheme are shown in and , for the nanoparticles and the in situ gelling system, respectively.
Figure 2. Response surface images for size and entrapment efficiency for nanoparticles. (a) Contour plots for size optimization, (b) 3D response surface image for size optimization, (c) Contour plots for entrapment optimization, (d) 3D response surface image for entrapment optimization.
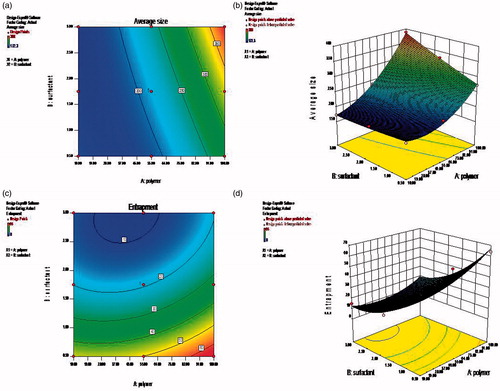
Figure 3. Response surface images for gelling temperature and drug release for in situ gels. (a) Contour plots for Gelling time optimization, (b) 3D response surface image Gelling time optimization, (c) Contour plots for Drug release optimization, (d) 3D surface response image drug release optimization.
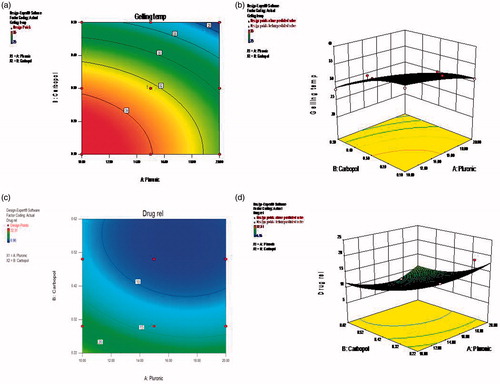
Particle size and zeta potential
The factorial design trial formulations of ACV-PCL nanosuspension had shown size distribution with wide range from 68 nm to 1281 nm. And the charge measurements expressed in terms of zeta potential was found to show negative values for all the formulations. The particle size distribution was found to be more narrow (105.7–396.05 nm) in formulation AF-3 with polydispersity index of 0.069. The zeta potential was higher in this formulation (−16.5 mV) compared to other trials, which indicated higher relative stability of the formulation.
Entrapment efficiency
The entrapment efficiency of the nanoparticles was found to be directly proportional to PCL and inversely proportional to Pluronic F127. As the amount of polymer was varied as 10 mg, 55 mg, 100 mg, and Pluronic F127 as 0.5%, 1.75%, 3%, the formulation AF-3 containing highest level of PCL (100 mg) and lowest concentration of Pluronic F127 (0.5%) showed maximum entrapment of 64% compared to the other trials. The total drug content evaluation of this nanosuspension showed 96% of drug, either encapsulated in polymer or remaining suspended in the supernatant. The physicochemical parameter results of the ACV-PCL nanoparticles are given in .
Table 5. Characterization of ACV-PCL nanoparticles.
Scanning electron microscopy
The SEM images () of pure Acyclovir particles showed crystalline flakes like structure. But the images of the lyophilized nanoparticles clearly indicate the uniform shaped spherical and smooth surface morphology. Even though the shape was homologous in the formulation, the size of the particles showed wide range distribution as confirmed by the results of particle size analyzer. The blank nanoparticles as observed by SEM to check whether nanodimension spheres can be formed using the respective polymer and surfactant, showed an evidence for the formation of nanospheres. Further optimization was done for the concentration of polymer and surfactant during the trial formulations along with drug.
X-ray diffraction studies
The XRD spectrum () of Acyclovir pure drug showed sharp peaks representative of crystalline material. And the spectrum of blank nanoparticles containing PCL showed distinct peaks of the polymer. The polymer-encapsulated ACV nanoparticles showed the identity points of the polymer with slight variation in its intensity and weakened peaks of the drug.
Fourier transform – infra red spectroscopy
The FTIR spectra () of Acyclovir pure drug, drug-encapsulated nanoparticles and blank polymeric nanoparticles without drug were obtained representing their specific functional groups. The wave numbers of the FTIR spectra of pure Acyclovir revealed the presence of similar functional groups as quoted by literature. The wave number of various functional groups identified was 3521.72 cm−1, 3440.86 cm−1 and 1215.8 cm−1 for alcoholic –OH group; 3182.89 cm−1 and 2854.29 cm−1 for –CH stretching; 1714.68 cm−1 for –C=O Ketone stretching; 1641.15 cm−1 and 1633.44 cm−1 for –C=C stretching; 1387.27 cm−1, 1347.48 cm−1 and 1308.70 cm−1 for amine group; 1105.78 cm−1, 1012.76 cm−1 and 1048.33 cm−1 for –C-N stretching; 902.00 cm−1 for –N-H stretching. The blank nanoparticles showed the characteristic peaks of the polymer. The PCL-ACV nanoparticles showed both polymer and drug peaks with mild shift in the wave numbers and also less intense peaks of Acyclvoir compared to pure drug. But the range of wave numbers lied within the standard region chart of Acyclovir as 3436.64 cm−1 for alcoholic –OH; 2941.50 cm−1 and 2870.45 cm−1 for alkyl –CH3 group; 1728.5 cm−1 for –C=O group; 1697.8 cm−1 for –C=C stretching; 1367.5 cm−1 for nitro group; 1242.5 cm−1, 1108.10 cm−1 and 1295.0 cm−1 for –C-N stretching.
Thermo gravimetry – differential scanning calorimetry
The DSC graph () of pure drug indicated a sharp endothermic peak at 252.77 °C corresponding to its melting point. At the same point, the weight reduced from 100% to 95% in the thermogravimetric curve. The blank nanoparticles containing PCL and Pluronic showed a sharp endothermic peak at 58.05 °C indicating the melting range of both polymer and surfactant followed by an exothermic curve and blunt endotherm region at 391.49 °C where they could be decomposed. Also, there was no weight loss till 350 °C, after which weight loss was observed in the TG curve, leading to a residual weight of 3.758%.The ACV-PCL nanoparticles showed the endothermic peaks at 235.80 °C and 56.52 °C, clearly corresponding to the melting of drug and polymer, respectively.
pH and drug content uniformity of in situ gelling system
The pH of the sol systems was maintained at neutral level and was confirmed by its results. As the optimized nanosuspension (AF-3) containing 10 mg equivalent of Acyclovir was dispersed into the colloidal gelling system, the uniformity in drug content for all the sol formulations were found to be within limits of 90–100%.
Sol-gel transition time and temperature
The sol-gel transition temperature was found to be in the range of 25 °C–35 °C for the variable combination of Pluronic F127 and Carbopol 940 gelling systems. Formulation containing highest concentration of Pluronic F127 (20%) showed gelation at lower temperature (25 °C) and shorter time (two minutes) compared to other formulations showing gelation temperature up to 35 °C and gelation time of five minutes. The physicochemical characterization results of the in situ gelling system are shown in .
Table 6. Characterization of in situ Gels.
Viscosity of sol – gel systems
The rheological variation in the sol and gel are shown in . The proportion of Pluronic F127 and Carbopol in each batch exhibited linear variation in their viscosity. Formulation AIG-1, AIG-3, AIG-7 and AIG-8 containing 15–20% of Pluronic and 0.3–0.5% of Carbopol was found to show highest viscosity (over 1000 cP) at 10 rpm in the sol state. Their corresponding viscosities at gel state elevated up to 5000 cP. The in vitro viscosity measurements taken under room temperature (20 °C) and in vivo body temperature (37 °C) clearly showed the sol to gel transition. Also, the presence of Carbopol as bioadhesive polymer had shown significant influence in the viscosity of gels.
Drug release from nanoparticles
The percentage of drug release found at every one hour time interval for up to eight hours () showed slow and sustained outcome of the drug from the nanoparticulate system. The release was 15–40% till 1st hour followed by increase in drug concentration up to the range of 60–100% till 8th hour, in the designed nanoparticles. The release was more sustained in formulation AF-3 showing 15% till 1st hour and 60% till 8th hour (as per USP specification limits for sustained release), which contain 100 mg of PCL and 0.5% of Pluronic F127, compared to other composition. Even though a similar release profile was observed with the formulation AF-7, the entrapment level of this trail was only 8%, due to low concentration of polymer (10 mg) and high concentration of surfactant (3%). When the amount of PCL was modified as 10 mg or 55 mg with respect to increase in the surfactant concentration as 1.75–3%, the drug release was not linear and found to be less sustained.
Drug release from in situ gelling system
The percentage of drug release till 1st hour was 2.75–4.22% extending to the maximum level of 6.96–32.31% at the end of eight hours. When the sol was placed in the media at 37 °C, it converted into gel immediately followed by sustained drug release. Formulation (AIG-1) with 20% Pluronic and 0.5% Carbopol was found to show 3% drug release at 1st hour elevating gradually up to 8% only at eight hours which provided very slow drug release than the required level. Whereas, the formulation (AIG-7) containing 15% Pluronic and 0.3% Carbopol provided linear drug release as 4% till 1st hour leading to 14% at 8th hour, which was identified to be optimum. Because a proper design of sustained release dosage form required for the drugs with low elimination half-life to avoid the frequent dosing, the formulation which provide optimum release was selected as the suitable trial. The polymer and micelles encapsulated drug acts like a reservoir from which free drug will be released in a sustained manner (Barichello et al., Citation1999).
Mechanism of drug release from nanoparticles and in situ gels
As evidenced by the kinetic study for the in vitro drug release data, the R2 values of all the trials of ACV-PCL nanoparticles and the nanoparticles incorporated in situ gels are shown in . The nanoparticles were found to follow Korsmeyer–Peppas kinetics except AF-2 and AF-6 where Hixson Crowell kinetics was predominant. Similarly, the drug release from the in situ gelling systems showed the Korsmeyer–Peppas kinetics for all the trials. The n-value for both the nanoparticles formulation and in situ gelling system was found to be in the range between 0.45 and 0.89.
Table 7. Drug release kinetics from nanoparticles and in situ gels.
Diffusion of drug through ex-vivo skin membrane
The diffusion of Acyclovir from the optimized formulation (AIG-7) through the excised buccal skin membrane showed linear pattern of release. The profile was comparable to the in-vitro dissolution study of the same formulation. In the ex-vivo study, around 3% was released in the 1st hour cumulatively increasing to 12.5% at the end of 8 hours, whereas the in-vitro profile showed 4 to 14% from 1st to 8th hour, respectively ().
Cytotoxicity studies
The in vitro cytotoxicity of ACV-PCL nanoparticles evaluated by MTT assay by varying the concentration from 6.25 to 100 µg/mL using the mouse embryonic fibroblasts cell line (NIH 3T3) was compared with the pure drug. The cell viability of the 100 µg/mL concentrated solution of the nanoparticles and the pure drug after 48 h of incubation was found to be 66% and 86%, respectively. The comparative results of the study are represented in .
Discussion
Factorial design of ACV-PCL nanoparticles and its in situ gelling system
The mathematical equations (Equations 1–5) shown in the results for factorial design of nanoparticles and in situ gelling systems revealed the second-order model with twisted plane curvature arising from the quadratic terms (Anthony & Kenneth, Citation1996). The contour plots were obtained plotting two independent variables against the responses with its 3D response surface image. With the above analysis, the formulation was optimized for higher entrapment efficiency (100%) and suitable size (<200 nm) for nanoparticles, and low gelling time (<35 °C) and more sustained drug release (>24 hrs) for in situ gels through the graphical method. Based on their desirability value, the optimized formulation with its respective independent variable showing expected response was found to be AF-3 (containing 100 mg of PCL and 0.5% of Pluronic F127) which showed average particle size of 201 nm and maximum entrapment efficiency of 64% for the designed nanoparticles, and AIG-7 (containing of 15% of Pluronic F127 and 0.3% Carbopol 940) with gelling temperature of 32 °C and sustained drug release of 13% at 8th hour for the nanoparticle incorporated in situ gels.
Effect of polymer and surfactant ratio on particle size distribution and surface charge
As highlighted in the results, the formulation AF-3 showing narrow size distribution range (105.7–396.05 nm) also approved by its pdI value of 0.069, confirmed the monodispersity (0.05–0.08) of the nanosized particle in this suspension, compared to the other suspension where a mid range of polydispersity (0.08–0.7) was observed (Philipus & Barbara, Citation2006). The negative charge shown by the nanoparticles was due to surface modification of Acyclovir with negatively charged PCL polymer in aqueous media in presence of the nonionic surfactant (Seok et al., Citation2010). Also, the zeta potential was higher in formulation containing higher amount of PCL (100 mg) and lower amount of Pluronic F127 (0.5%), indicating the better stability of the nanosuspension with less agglomeration or settling of particles. In further trials, when the surfactant concentration was increased further with respect to change in the polymer ratio, the zeta potential was lesser, which might be due to less surface modification effects on the freely dissolved or nonencapsulated drug in the media.
The size of the nanoparticles was influenced by the concentration of polymer used, wherein a slight increase in size with respect to increase in the polymer level was observed (Prasad Rao et al., Citation2011). But, when the size range of formulations AF2 (105.7 nm–1106.43 nm) and AF3 (105.7 nm–396.05 nm) were compared (), it was observed that AF2 containing less polymer content showed larger average size than AF3. Because the former showed wide size distribution, the average size calculated by the analyzer was higher than the latter, which had narrow size range. Also, it was proved by the PDI value that AF2 is polydisperse and AF3 is monodisperse in nature, one of the main reasons to select AF3 as the optimized formulation.
Encapsulation efficiency at variable polymer level
The formulation containing highest concentration of polymer and lowest amount of surfactant provided more entrapment, because at higher concentrations of the surfactant the solubility of the drug was enhanced and hence the drug remained freely solubilized, unentrapped and more concentrated in the supernatant (Zohra et al., Citation2005). Also, when the total drug content of the nanosuspension was evaluated, the percentage of drug concentration was higher than the entrapped concentration, which clearly indicated the presence of unentrapped nanosized drug particles in the supernatant solution. And the reduction of total drug content showing less than 98% indicated the loss of unentrapped drug removed by centrifugation at 5000 rpm.
Morphology changes of acyclovir in nanoparticles
The modification of crystalline orthorhombic structure of Acyclovir pure drug into smooth surfaced spherical nanospheres, (Hellen et al., Citation2009; Selvaraj et al., Citation2010) due to polymer (PCL) encapsulation was confirmed by the comparison of the respective scanning electron microscopic images. Also, the blank nanoparticles of PCL formulated without drug, have shown the spherical particles, which also accounted for the formation of uniform-shaped ACV-PCL nanoparticles. This also revealed that the polymer and the drug possess properties of forming stable nanodimension particles.
Crystallinity behavior of acyclovir in nanoparticles
The ACV-PCL nanoparticles in which the drug was encapsulated within the PCL polymer matrix showed intense peaks of the polymer and blurred peaks of the drug, due to diminished crystal character of the drug. The unprocessed original drug is in pure state and completely crystalline nature, whereas in the formulation, the drug exists in the nanosize and not necessarily observed in its original crystalline nature. There is solid state transition in crystallinity of Acyclovir to semicrystalline or amorphous form due to polymer/excipient interactions, whereby the structure of the drug is less likely to construct its crystal lattice (Yu et al., Citation2011). This could be attributed to the improvement in its solubility and other physical properties (Takaaki et al., Citation2012). The XRD spectra of the PCL (blank) nanoparticles and the ACV-PCL nanoparticles are nearly super imposable, where the intensity of the peaks of PCL was slightly lower in the formulation compared to the blank, due to its minor crystallinity changes during entrapment of the drug. In case of ACV-PCL nanoparticles, the drug is encapsulated by the polymer matrix, especially at higher proportion whereby, more amount of polymer will be on the surface, so the peaks of polymer is more prominent and are found to be similar to blank.
Interaction of drug–polymer
The wave numbers of the functional groups for drug and polymer were shifted from its normal range which revealed that there was mild interaction of drug with polymers due to hydrogen bonding (Yu et al., Citation2011), yet the existence of drug molecule with its characteristic functional groups could be confirmed from its relevant peaks in the nanoparticles (Alicia & Ching, Citation2013). It was obvious that the intensity of polymer peaks was dominant than the drug peaks due to its encapsulation and its higher ratio (10:1) in the formulation. The presence of drug without significant modifications was also confirmed by drug content analysis and in vitro drug release studies.
Modification of thermal stability of drug in nanoparticles
The changes in the weight loss and melting range of drug, polymer and their nanoparticles were depicted by the TG-DSC thermograms of Acyclovir pure, blank nanoparticles and the ACV-PCL nanoparticles, respectively. The weight loss from 100% to 95% observed in the range of 30–260 °C at heat flow of −30 to −40 mW in pure Acyclovir sample could be attributed to either desorption of physically adsorbed water or removal of chemisorbed water (Ioannis et al., Citation2010). A further increase in temperature from 300 °to 1000 °caused complete removal of moisture from its crystal to give yield of 9.767% residue weight. Whereas the TG curve of blank nanoparticles and ACV-PCL nanoparticles could be super imposed which indicated the weight loss after 350 °C only. The melting range of both drug and polymer in the nanoparticles was slightly shifted from 252.77 °C to 235.80 °C and 50.05 °C to 56.52 °C, respectively, as compared to its pure sample, the significant reason was the entrapment of drug into polymer and their weak H-bonding interactions as well as solid state modification.
Effect of polymer composition on sol-gel transition
Because temperature was the main trigger for in vivo gelation of the Pluronic sol, the temperature and time needed for gel transformation was considered. Apart from the formulation containing highest content of 20% Pluronic showing less gelation time and temperature, certain formulations having even lower Pluronic concentration of 10–15% but higher Carbopol content of 0.5% could provide gelation time within three minutes at temperature of 28 °C. This specifically indicated that even though Carbopol was intended for its bioadhesive property, its concentration could influence the gelation time (Rathore, Citation2010).
Influence of gelling and bioadhesive polymer on rheology
The viscosity measurements of the sol (cold condition) and gel (28–35 °C at gelation point) depicted the change in flow behavior with respect to shear rate, which indicated the Non-Newtonian type of system (Tirnaksiz et al., Citation2005). When the speed of rotation of the spindle was increased from 10 to 200 rpm, viscosity was found to decrease for all formulations representing pseudoplastic nature. The sol-gel transition with respect to time and temperature, represented over time at constant shear proved the flow as thixotrophic behavior. The combination of Pluronic and Carbopol sol-gel system exhibited shear thinning property and thermoreversible transitions (Ibrahim et al., Citation2010). Even though Carbopol was meant as pH-sensitive polymer, it was found to influence the rheology of thermosensitive Pluronic sol-gel system, despite the neutral pH maintained in the sol. This could be attributed to its high viscosity and adhesive nature that can play considerable role for in vivo bioadhesion also.
Role of polymer encapsulation and surfactant level on drug release from nanoparticles
The sustained and linear drug release observed in the nanoformulation can be related to the entrapment of the drug within the polymer and its concentration in the supernatant of the nanosuspension (Thirumala et al., Citation1999; Vanessa et al., Citation2011). The initial release at 1st hour could be due to the unentrapped drug nanoparticles in the suspension, followed by the gradual elevation in drug concentration up to eight hours due to drug liberated from the entrapped polymeric system. This could be clearly evidenced in the formulations containing high polymer and low surfactant concentration with higher drug entrapment could provide less initial release and more sustained release (Ibrahim et al., Citation2010). Alternatively, an immediate initial release followed by less sustained release was exhibited by other formulations due to poor entrapment of drug by the lower ratio of polymer and also because of more solubilization of drug in supernatant by the higher levels of surfactant. It was evident that the increase in polymer concentration and decrease in surfactant quantity to an optimum level could provide better polymeric encapsulated Acyclovir nanoparticles and in supplementary effective controlled release of the drug.
Control of gelling polymer on drug release
As the nanosuspension which showed more entrapment, optimum particle size and effective controlled release (AF-3) was dispersed into the in situ gelling polymers, still more sustained drug release was achieved by the system, because even the PCL-unentrapped nanosized drug present in the nanosuspension could be entrapped into the sol-gelling dispersion media. Even though Pluronic was intended as the gelling polymer, presence of Carbopol at different percentage also modified the drug release because of its gluey, tacky and bioadhesive nature. This was evident from the formulations having 20% Pluronic as fixed but Carbopol varied as 0.1%, 0.3% and 0.5%, out of which the former two showed more and the later provided less drug release. Also the reverse was observed in the cases where only 10% or 15% Pluronic was present as gelling base, but when Carbopol was at higher concentration (0.5%), the liberation of drug was more controlled. Eventually the formulation with top level of both polymers exhibited very slow drug release and the reverse observed with least level of Pluronic and Carbopol showing higher drug release (Patel et al., Citation2012; Shital, Citation2012). The intermediate concentration of Pluronic (15%) and Carbopol (0.3%) was found to provide linear and optimum drug release, which was also authenticated by the results shown through the factorial design optimization.
Impact of delivery system on drug release mechanism
The mechanism of the drug release predicted from Korsmeyer–Peppas model could be attributed to diffusion of media into the system followed by drug diffusion, for both the nanoparticle and in situ gelling systems. In case of formulation AF-2 and AF-6, the kinetics following Hixson Crowell model indicated that the drug release from the nanoparticulate system could be correlated to the change in surface area and diameter of particles. Moreover, the higher R2 value observed in first-order and Higuchi model kinetics for most of the formulations indicated that the drug release process was both concentration and time dependent. In both cases, the type of diffusion mechanism was found to be Anomalous non-Fickian transport, as indicated by the n value between 0.45 and 0.89 (Rajendran et al., Citation2010; Gautam et al., Citation2011).
Comparison of in-vitro dissolution and ex-vivo diffusion
The diffusion behavior of Acyclovir from the optimized in situ gelling system containing polymeric nanoparticles showed a gradual increase in the percentage drug release over a period of 8 hours. The release profile of the ex-vivo diffusion study showed similar pattern as in-vitro dissolution study, wherein the percentage of release was found to be slightly lesser in the ex-vivo study (Darshana et al., 2011). The reason was due to the difference in thickness of pores of the skin membrane containing many layers, compared to the thin dialysis membrane with uniform pores used in the in-vitro dissolution study.
Cytotoxicity effect of nanoparticles
The slight increase in toxicity was found in drug incorporated PCL nanoparticle compared to pure drug. It may be due to negative surface charge of the nanoparticles, which caused an increase in its uptake by proteins present on the cell surface leading to slight increase in the toxicity. The cytotoxicity of ACV from previous studies indicated the similar level of toxicity at different concentrations (Zhengwei et al., Citation2007; Haetrakul et al., Citation2010).
Conclusion
The stability and efficiency of the nanosuspension to provide slow release of Acyclovir from PCL polymer was evidenced by their characterization and release studies. Also, the morphology, interaction study, thermal analysis and cytotoxicity evaluation contributed for the supportive confirmation for the acceptability of the optimized polymeric nanoparticle formulation. The sol-gel transition, pH, temperature, rheology and drug content encouraged the development of thermosensitive in situ gelling system. Moreover, the nanoparticle incorporated into in situ gelling systems could provide more sustained drug release than the polymeric nanoparticles alone, which also authenticated that the dual sustained effect achieved by the system. The novelty of the formulation can be assessed by in vivo bioavailability estimation studies through various routes. Also, suitable in vivo and ex vivo techniques can be applied to understand the mechanism of absorption and biodistribution process and also study the influence of Carbopol in rheology and bioadhesion force, etc. Because the in situ gelling systems have been proved to be suitable for administration through many routes like ocular, nasal, rectal, vaginal, etc., by various researchers, Acyclovir nanoparticle incorporated gel for treatment of viral infections at specific sites is also a unique and promising system for site-specific sustained action.
Declaration of interests
The authors report no declarations of interest.
Acknowledgements
The authors are thankful to the management of SASTRA University for funding this research work under Prof. T.R.R. grant scheme, and KMCH college of Pharmacy for performing the cell lines studies. Also, the authors extend heartily thanks to Dr. K.S. Rajan, Associate Dean – Research, SASTRA University, and Mr. B.N. Vedha Hari, Assistant Professor, SASTRA University, for their valid guidance and support during the entire study.
References
- Abdel-Mottaleb MM, Lamprecht A. (2011). Standardized in vitro drug release test for colloidal drug carriers using modified USP dissolution apparatus I. Drug Dev Ind Pharm 37:178–84
- Abdelwahed W, Degobert G, Stainmesse S, Fessi H. (2006). Freeze-drying of nanoparticles: formulation, process and storage considerations. Adv Drug Delivery Rev 58:1688–713
- Alain C, Abdellatif AK, Julianna J. (1997). Study of the gelation process of polyethylene oxide –polypropylene oxide – polyethylene oxide copolymer (Poloxamer 407) aqueous solutions. J colloid interface sci 190:307–12
- Alicia JS, Ching AP. (2013). Guanosine-based antiviral Acyclovir incorporated in ring-opening polymerization of ε-caprolactone. Macromol Res 21:1--4
- Anthony AN, Kenneth CJ. (1996). Pharmaceutical experimental design and interpretation. USA: Taylor & Francis, 169–192
- Barichello JM, Morishita M, Takayama K, Nagai T. (1999). Absorption of insulin from Pluronic F-127 gels following subcutaneous administration in rats. Int J Pharm 184:189–98
- Cristina F, Sergio S, Rogerio G. (2002). Paclitaxel-loaded PLGA nanoparticles: preparation, physicochemical characterization and in vitro anti-tumoral activity. J Control Release 83:273–86
- Darshana SJ, Amrita NB, Nimisha T. (2011). In-vitro--in-vivo assessment and comparison of intranasally administered microemulsion formulations of essential oils for migraine. Int J Curr Pharm Res 3:4751
- Devi KV, Bhosale UV. (2009). Formulation and optimization of polymeric nano drug delivery system of Acyclovir using 32 full factorial design. Int J PharmTech Res 1:644–53
- Dhanalekshmi UM, Poovi G, Kishore N, Reddy NP. (2010). In vitro characterization and in vivo toxicity study of Repaglinide loaded poly (methyl methacrylate) nanoparticles. Int J Pharm 396:194–203
- Gautam S, Mahaveer S. (2011). Review: in vitro drug release characterization models. International J Pharm Stud Res 2:1–8
- Gohel MC, Par RK, Patel KD. (2009). Formulation and development of novel in situ nasal gel containing Glibenclamide nanoparticles. Nanotech Conference and Expo; 2009; Houston, Texas
- Grace Rathnam, Periyanayagi GK. (2012). Sustained ophthalmic delivery of Atenolol for Glaucoma by in situ gelling system. Int J Pharm Res Develop 4:291–301
- Guadalupe N, Elizabeth P, Luis M, Nestor M, David Q, Adriana G. (2011). Formulation and in-vitro, ex-vivo and in-vivo evaluation of elastic liposomes for transdermal delivery of Ketorolac Tromethamine. Pharmaceutics 3:954--970
- Haetrakul T, Tangtrongpiros J, Suthamnajpong N, Chansue N. (2010). Cytotoxicity concentration of Acyclovir and Clinacanthus nutans (Burm. f.) Lindau extract to Koi Fin cell line. Thai J Vet Med 40:108
- Hellen KS, Monika PT, Alexandre LP, et al. (2009). Evaluation of cross-linked chitosan microparticles containing Acyclovir obtained by spray-drying. Mater Sci Eng C 29:387–92
- Ibrahim MEB, Mohsen AB, Gamal MM, et al. (2010). Effect of gamma irradiation on pluronic gels for ocular delivery of Ciprofloxacin: in vitro evaluation. Aust J Basic Appl Sci 4:4490–8
- Ioannis AK, George K. (2010). Synthesis and characterization of cerium molybdate nanocontainers and their inhibitor complexes. J Am Ceram Soc 93:65–73
- Jeong B, Gutowska A. (2002). Lessons from nature: stimuli responsive polymers and their biomedical applications. Trends Biotechnol 20:305–11
- Kamel AO, Awad GAS, Geneidi AS, Mortada ND. (2007). Preparation and characterization of Acyclovir nanoparticles by double emulsion technique. Egypt J Biomed Sci 23:218–36
- Lee DS, He C. (2010). In situ gelling stimuli-sensitive PEG-based amphiphilic copolymer hydrogels. In: Ottenbrite RM, et al., eds. Biomedical applications of hydrogels handbook. New York, Dordrecht, Heidelberg, London: Springer, 123--46
- Monks A, Scudiero D, Skehan P, et al. (1991). Feasibility of high flux anticancer drug screen using a diverse panel of cultured human tumour cell lines. J Natl Cancer Inst 83:757–66
- Nirmal HB, Bakliwal SR, Pawar SP. (2010). In situ gel: new trends in controlled and sustained drug delivery system. Int J PharmTech Res 2:1398–408
- Patel DB, Patel SR, Patel NK, Patel MM. (2012). Temperature and pH triggered in situ hydrogel of Doxycycline for the treatment of chlamydial conjunctivitis. Int J Pharm Res Sch 1:1–7
- Philipus JP, Barbara JF. (2006). Direct determination of the number-weighted mean radius and polydispersity from dynamic light-scattering data. Appl Opt 45:2209–16. Available from: http://dx.doi.org/10.1364/AO.45.002209 [last accessed 9 Nov 2012]
- Prasad Rao J, Geckeler KE. (2011). Polymer nanoparticles: preparation techniques and size-control Parameters. Prog Polym Sci 36:887–913
- Pravin K, Rajendra A, Puneet KR, et al. (2010). Mucoadhesive in situ gels of local anaesthetic for periodontia. Der Pharmacia Lettre 2:28–39
- Rajendran NN, Natrajan R, Siva Kumar R, Selvaraj S. (2010). Acyclovir-loaded chitosan nanoparticles for ocular delivery. Asian J Pharm 4:220–6
- Rathore KS. (2010). In situ gelling ophthalmic drug delivery system: an overview. Int J pharm Pharm Sci 2:30–4
- Rejinold SN, Muthunarayanan M, Muthuchelian K, et al. (2011). Saponin-loaded chitosan nanoparticles and their cytotoxicity to cancer cell lines in vitro. Carbohyd Polym 84:407–16
- Ricci EJ, Lunardi LO, Nanclares DMA, Marchetti JM. (2005). Sustained release of lidocaine from Poloxamer 407 gels. Int J Pharm 288:235–44
- Roberta C, Manuela D, Andrea C, et al. (2009). Enhanced antiviral activity of Acyclovir loaded into β-cyclodextrin-poly(4-acryloyl morpholine) conjugate nanoparticles. J Control Release 137:116–22
- Saurabh G, Malay KS, Ashok MR. (2010). Dual-drug delivery system based on in situ gel-forming nanosuspension of Forskolin to enhance antiglaucoma efficacy. AAPS Pharm Sci Tech 11:322–35
- Selvakumar K, Yadav AV. (2009). Formulation and evaluation of Carvedilol loaded Eudragit E100 nanoparticles. Int J Pharm Tech Res 1:179–83
- Selvaraj S, Saravanakumar N, Karthikeyan J, et al. (2010). Acyclovir loaded chitosan nanoparticles for ocular delivery. Der Pharmacia Lettre 2:420–31
- Seok WP, Kristin NW, Sundararajan VM. (2010). In vitro characterization of polycaprolactone matrices generated in aqueous media. Acta Biomater 6:1061–8
- Shital U. (2012). Formulation and development of in situ gelling system for nasal administration for an antiemetic drug Ondansetron hydrochloride by using pluronic 127P and pluronic F68. Int J Res Pharm Biomed Sci 3:1103–18
- Song YQ, Zhang SX, Yin YZ, Deng SH. (2011). Preparation of the Insulin chitosan nanoparticle thermosensitive in situ gel and its drug releasing performance in vitro. Chin J Hosp Pharm 2:116--120
- Sonjoy M, Manjunath KMJT, Prabhushankar GL, Geetha MS. (2012). Formulation and evaluation of an in situ gel-forming ophthalmic formulation of Moxifloxacin hydrochloride. Int J Pharma Investig 2:78–82
- Susantakumar P, Ajay G, Piush S. (2011). Comparative pharmacokinetics, safety and tolerability evaluation of Acyclovir IR 800 mg tablet in healthy Indian adult volunteers under fasting and non-fasting conditions. J Bioequiv Availab 3:128–38
- Suvakanta D, Padala NM, Lilakanta N, Prasanta C. (2010). Kinetic modeling on drug release from controlled drug delivery systems. Acta Pol Pharm Drug Res 67:217–23
- Takaaki M, Yasuo Y, Etsuo Y, et al. (2012). Cocrystallization and amorphization induced by drug–excipient interaction improves the physical properties of Acyclovir. Int J Pharm 422:160–9
- Thirumala G, Snjezana S, Martin CG, et al. (1999). PLGA nanoparticles prepared by nanoprecipitation: drug loading and release studies of a water soluble drug. J Control Release 57:171–85
- Tirnaksiz F, Robinson JR. (2005). Rheological, mucoadhesive and release properties of pluronic F-127 gel and pluronic F-127/polycarbophil mixed gel systems. Pharmazie 60:518–23
- Vanessa AM, Eduardo RJ. (2011). Encapsulation of Naproxen in nanostructured system: structural characterization and in vitro release studies. Quim Nova 34:933–9
- Yu DG, Zhu LM, Branford-White CJ, et al. (2011). Solid dispersions in the form of electrospun core-sheath nanofibers. Int J Nanomedicine 6:3271–80
- Zhengwei M, Wang B, Lie M, et al. (2007). The influence of polycaprolactone coating on the internalization and cytotoxicity of gold nanoparticles. Nanomed - Nanotechnol 3:215–23
- Zohra Z, Souad S, Hatem F. (2005). Preparation and characterization of poly-ε-caprolactone nanoparticles containing Griseofulvin. Int J Pharm 294:261–7