Abstract
In the present study, the enhancing effect of 2-isopropyl-5-methylcyclohexyl heptanoate (M-HEP) on the percutaneous absorption of indomethacin (IM) was evaluated by the in vitro penetration experiments using the rat abdominal skin as a barrier. Partition experiment, attenuated total reflection-Fourier transform infrared (ATR-FTIR) spectrum and transepidermal water loss (TEWL), was employed to investigate the possible mechanisms of the action of M-HEP. Furthermore, the reversible effect of M-HEP on excised rat skin was also evaluated through in vitro permeation as a preliminary indicator of safety. The result of in vitro permeation experiment indicated that, 10% (w/w) M-HEP in combination with isopropyl palmitate (IPP) significantly increased (p < 0.05), the cumulative amount of IM in comparison with the control group (IPP only). The partition coefficient of IM between the stratum corneum (SC) and enhancer solution was also greater than that between the SC and IPP. A blue shift in the ATR-FTIR spectra of SC after treatment with M-HEP solution was observed at the CH2 band, which indicating that M-HEP disrupted the intercellular lipid structure of the SC. In addition, both M-HEP/IPP and L-menthol (MT)/IPP improved the TEWL value of rat abdominal skin. After removal of M-HEP, the skin barrier function would be restored in 8 h. In conclusion, M-HEP could reversibly enhance the percutaneous absorption of IM by increasing the partitioning of IM into the SC from enhancer solution and disturbing the organized structure of SC lipids and the reversibility of M-HEP was better than MT.
Introduction
The unique composition of the stratum corneum (SC) is critical for regulating water loss from the body and preventing the ingress of potentially harmful substance and microorganisms from the skin surface (Marjukka Suhonen et al., Citation1999; Bouwstra & Ponec, Citation2006). This protective function also prevents the uptake of drugs and the systemic absorption of therapeutically relevant doses of compounds. Thus, many strategies have been developed to improve the permeation of drugs through the skin (Barry, Citation2001). Among them, chemical penetration enhancer is one of the most widely used approaches for improvement of transdermal absorption (Williams & Barry, Citation2004). Although many substances have been identified as drug penetration enhancers, the undefined mechanisms of action and safety remain the major concern, which limits their practical use in topical or transdermal formulations (Karande, Citation2005; Karande et al., Citation2005). Therefore, the development of a novel relatively safer and potent enhancer is an attractive option for researchers.
2-isopropyl-5-methylcyclohexyl heptanoate (M-HEP) was synthesized as a novel candidate for percutaneous permeation enhancer using L-menthol (MT) as a lead compound in our laboratory () (Zhao et al., Citation2008). Compared with MT, M-HEP has no volatile or odor characteristics, which might make it more suitable for transdermal drug delivery preparations. In previous studies, M-HEP showed promoting effects on the percutaneous absorption of several drugs (Zhao et al., Citation2008, Citation2013). However, the mechanisms of the action of M-HEP and its safety have not been clearly elucidated.
The present work was carried out to demonstrate the in vitro transdermal enhancement effect of M-HEP at different concentrations, using indomethacin (IM) as a model drug (). In addition, the mechanisms of penetration enhancing effect of M-HEP were investigated with partition experiment, attenuated total reflection-Fourier transform infrared (ATR-FTIR) spectroscopy and transepidermal water loss (TEWL). Partition experiment was employed to gain insight into the influence of enhancer on the partition of the drug. ATR-FTIR and TEWL provided information about the influence of enhancer on the skin barrier properties from a molecular level to a macroscopic level. Furthermore, the reversible effect of M-HEP on excised rat skin was evaluated through in vitro permeation as a preliminary indicator of safety.
Materials and methods
Materials
IM and propylparaben were supplied by Dahongyin Pharmaceutical Co., Ltd. (Ningbo, China) and Xingjin Chemical Plant (Beijing, China), respectively. Isopropyl palmitate (IPP) and L-menthol were purchased from China National Medicines Co., Ltd. (Shanghai, China). M-HEP was synthesized in our laboratory. Glacial acetic acid and triethylamine were purchased from Bodi Chemical Holding Co., Ltd. (Tianjin, China). Methanol of high-performance liquid chromatography (HPLC) grade was obtained from Hanbon Sci. & Tech. Co., Ltd. (Jiangsu, China). All other chemicals were of the highest reagent grade available.
HPLC determination of IM
The determination of IM was performed by HPLC, using an internal calibration method with propylparaben as internal standard. A Hitachi instrument (Pump L-2130, UV–VIS Detector L-2420, Autosampler L-2200) and a Diamonsil® C18 (5 μm, 200 × 4.6 mm) column were used. The mobile phase consisted of methanol and 0.5% acetic acid in distilled water (3:1, v/v), and the pH was adjusted to 6.0 with triethylamine. The column was maintained at 40 °C, and the flow rate was 1 mL/min while the detection wavelength was 266 nm. The retention times in this assay for IM and internal standard were 5.8 and 3.9 min, respectively. The peak of IM was well separated by this analytical method from those of the internal standard and other endogenous components in the skin.
Determination of drug solubility in the donor vehicles
Saturated solutions of IM were prepared by adding excess of drug in appropriate vehicles, with and without chemical enhancers. The mixture was vortexed for 3 min followed by sonication for 10 min to dissolve the drug and then sealed in glass vials, equilibrated in a water bath at 32 ± 0.5 °C for 48 h. Finally, the obtained suspension was passed through a membrane filter with a pore size of 0.45 μm to obtain clear solution. Then, the saturated solution was diluted appropriately before assaying by the HPLC method as described. The experiments were performed in triplicate.
Skin preparation
Male Wistar rats weighing 180–220 g used in all experiments were supplied by the Experimental Animal Center of Shenyang Pharmaceutical University (Shenyang, China). The experiments were performed in accordance with the NIH Guidelines for the Care and Use of Laboratory Animals and also in accordance with the guidelines for animal use published by the Life Science Research Center of Shenyang Pharmaceutical University. The rats were anesthetized with urethane (20%, w/v, i.p.) and the abdomen was carefully shaved with a razor after removal of hair by electric clippers. Full-thickness skin was excised from the shaved abdominal site. Any skin which had a disrupted barrier was rejected. After removal of the fat and subdermal tissue, the skin was kept frozen at −70 °C and used within one week. Prior to the experiments, the skin was allowed to thaw slowly to reach the room temperature.
Preparation of SC membranes
To isolate the SC membrane from the excised rat abdominal skin, the skin was incubated in a petri dish containing 5% (w/v) trypsin in physiological saline with SC facing up. The petri dish was covered and kept at 37 °C for 8 h. After epidermal digestion, the SC was lifted using a filter paper and then washed with distilled water at least three times where excess water was removed using filter paper. To ensure complete removal of the viable epidermis, the viable epidermis side was wiped gently using a cotton swab. Subsequently, the SC samples were allowed to dry at room temperature, and then stored in a vacuum desiccator until use.
Preparation of donor solutions
Donor solutions were obtained by equilibration of excess amounts of IM in IPP, with and without chemical enhancers, vortexed for 3 min followed by a 10 min ultrasound treatment to obtain a uniform system. An excess amount of drug was present throughout the experiments.
In vitro permeation experiment
The skin permeation experiment was carried out at 32 °C in two-chamber side-by-side diffusion cells (with an effective diffusional area of 0.95 cm2 and receiver volume of 3 mL). Rat abdominal skin was mounted between donor and receiver compartments, with the SC side of the skin facing the donor compartment and the dermis side facing the receiver compartment. Excess skin at the sides was trimmed off to minimize lateral diffusion. The donor compartments were filled with donor solutions and the receiver compartments were filled with pH 7.4 phosphate buffer solutions (PBS). During all permeation experiments, excess drug was maintained in the donor compartments in order to keep a constant drug concentration. Both donor and receiver compartments were stirred with a star-head bar driven by a constant speed synchronous motor at 600 rpm. At predetermined time intervals, 2.0 mL of receptor solution was withdrawn from each receiver compartment for analysis and replaced with the same volume of fresh PBS. All the experiments were repeated at least four times. The collected samples were centrifuged at 10 000 rpm for 5 min, and the supernatants were determined by reversed phase HPLC with reference to the calibration curve.
Partition of IM between SC and enhancer solution
Ten milligrams of vacuum-dried SC pieces were hydrated for 24 h in physiological saline before use, and then incubated in 1 mL of sub-saturated control (IPP) or enhancer solution. The suspensions were vortexted for 24 h at ambient temperature to reach equilibrium. The supernatants, obtained at the end of 24 h by centrifugation, were analyzed for the drug content. The amount of IM partitioning into the SC was obtained by subtracting the drug concentration in the supernatants from the initial drug concentration.
Attenuated total reflection Fourier-transform infrared spectroscopy
The SC was cut into small circular disks with approximate diameter of 1 cm and hydrated for 24 h in physiological saline. After hydration, these disks were thoroughly blotted over filter paper. Then, SC pieces were incubated for 1.5 h in 2 ml of the respective formulations at room temperature, where the formulations were the same as the donor solutions of the in vitro permeation experiment. Thereafter, the SC pieces were cleaned carefully to remove all the residual solution on the surface. Then, the SC samples were vacuum-dried and subjected to ATR-FTIR spectroscopic study. An ATR-FTIR (Thermo Nicolet NEXUS 470, Madison, WI) system equipped with an ATR accessory was used to accomplish this study. The internal reflection crystal which was made of zinc selenide (ZnSe) had a 45 ° angle of incidence to the infrared (IR) beam. Spectra were acquired in the frequency range 4000–400 cm−1. All spectra analyzed represented an average of 32 scans with a resolution of 0.4 cm−1. All experiments were performed in triplicate at ambient temperature 23 ± 2 °C.
In vivo transepidermal water loss
TEWL measurements were performed on the abdomen of male rats (200 ± 10 g) in vivo according to the published standardized guidelines (Pinnagoda et al., Citation1990; Rogiers, Citation2001), with an open-chamber Tewameter® (TM 300, Courage + Khazaka electronic GmbH, Cologne, Germany). The test solutions were prepared as follows: drug solution was obtained by suspending excess amount of IM in IPP; enhancer solutions were acquired by adding 10% (w/w) M-HEP or MT in the drug solution. Prior to measurement, the rats were anaesthetized with 20% (w/v, i.p.) ethyl urethane, followed by the removal of the abdominal hair, and then remained physically inactive at least 15 min in a room of reasonably constant temperature (22 ± 2 °C, RH 50 ± 5%). Three test circle regions with a diameter of 2.5 cm were marked on the abdominal skin as shown in . The baseline values of TEWL were obtained by holding the Tewameter® probe over the skin until stable TEWL value reached (about 60 s after the placement of the probe). After topical application of 20 μL test solutions on marked sites for 1.5 h, the residual test solutions were gently removed with cotton swabs. The TEWL values of treated sites were determined and compared with the baseline TEWL values to obtain the ΔTEWL, i.e. ΔTEWL = TEWLtreated − TEWLbaseline. All experiments were performed in triplicate and the mean values were reported.
Reversibility study
Reversibility studies were modified from the three-step experiment of Bhatia & Singh (Citation1998). During step I, the skin was pretreated with enhancer solutions for 1.5 h. This step was similar with the in vitro permeation experiments except the donor solution. The donor compartment was filled with 10% enhancer solution which was prepared by dissolving enhancer in IPP. In step II, the receiver solution was withdrawn and replaced with fresh PBS. In order to eliminate the enhancer retention problem, the donor compartment was totally replaced by a new one and filled with IPP. Thereafter, the skin was allowed to recover for a predetermined time. In step III, the receiver and donor solutions were withdrawn leaving the epidermis intact in the diffusion cells. Then, the donor compartment was filled with drug solution which was obtained by adding excess amounts of IM in IPP, and the receiver compartment was filled with fresh PBS. The transport of IM was carried out for 8 h as explained in the in vitro permeation experiments. The samples were assayed by the HPLC method as above. Following the experiments, the cumulative amount of IM through enhancer exposed skin was compared with the control (only IPP exposed skin and recovered the same time as enhancer pretreated skin). All experiments were performed in quadruplicate, and the results were expressed as the mean ± SE of four experiments. The Recovery Index (RI) was calculated as
Data analysis
The cumulative amount of drug was calculated by the following formula:
where Q is the cumulative amount of drug in μg cm−2, Ci (μg mL−1) is the concentration in the receiver compartment at the time i, V is the volume of compartment in mL and A is the effective diffusion area in cm2. The cumulative amount of drug permeating through the skin was plotted as a function of time. The skin steady state flux, J (μg cm−2 h−1), was calculated from the linear region of the plot and expressed as
The lag time (Tlag) was determined by extrapolating the linear portion of the curve to the abscissa.
To evaluate the promoting activity of each enhancer, the enhancement ratio (ER) was calculated as
The permeability coefficient, vehicle/SC partition and diffusion coefficient were calculated as
where P is the permeability coefficient, Cs is the concentration of drug in donor solutions, K is the partition coefficient, D is the diffusion coefficient and L is the diffusion path length. Since the exact path length of the permeant is difficult to determine, the partition coefficient (K) and diffusivity (D) were normalized as apparent partition coefficient K′ (KL) and apparent diffusion coefficient D′ (D/L2), respectively (Nakamura et al., Citation1996), and these parameters can be calculated using the following equations:
All data were expressed as the mean value ± SE. Student’s t-test was used to compare two group data with the level of significance set at p < 0.05.
Results and discussion
In vitro permeation experiments
The choice of vehicle plays an important role in the evaluation of the activity of the penetration enhancers because the vehicle can affect both drug release and percutaneous absorption. In our study, IPP was employed as a vehicle for its low toxicity and desirable compatibility with the skin.
The permeation profiles of IM with different enhancer concentrations through rat abdominal skin were shown in and . From the profiles, we can see that M-HEP had an enhancing effect on IM permeation. At the concentration of 10% (w/w), M-HEP exhibited the most remarkable enhancing effect on the cumulative transport amount of IM than that of 5% (w/w) and 20% (w/w) (p < 0.05), with the cumulative amount being 38.50 ± 4.52 μg cm−2. For MT, the cumulative transport amount of IM was enhanced only at a high concentration (20%, w/w), with the cumulative amount of 29.52 ± 2.93 μg cm−2. At the concentration of 5% (w/w) and 10% (w/w), MT did not significantly increase the cumulative amount of IM compared with the control (without enhancer) (p > 0.05), with the cumulative amount of 21.66 ± 3.37 μg cm−2 and 24.25 ± 1.48 μg cm−2, respectively. In order to elucidate the results of in vitro permeation experiment, the solubility of IM in the donor solution was determined (). The addition of M-HEP slightly increased the solubility of IM in the donor solution, but the solubility of IM did not increase with the increasing concentration of M-HEP. In the case of MT, the solubility of IM increased with the increasing concentration of MT. However, the high solubility of IM in the presence of MT did not contribute significantly to the permeation of IM.
Table 1. Permeation parameters of IM with different enhancer concentrations through rat abdominal skin in vitro (mean ± SE, n = 4).
Penetration enhancement is a consequence of either an increase in the diffusion coefficient (D) or the partition coefficient (K) of the drug, or both (Dias et al., Citation2001). shows the permeability coefficient (P), apparent diffusion parameter (D/L2) and apparent partition parameter (KL) of IM in the absence and presence of the enhancers. It was revealed that M-HEP exerted an enhancing effect by increasing both diffusion and partition while MT significantly reduced the apparent partition parameter of IM in the IPP system.
Table 2. Permeability coefficient (P), apparent diffusion parameter (D/L2) and apparent partition parameter (KL) of IM in the absence and presence of the enhancers.
Generally, enhancers act by more than one mechanism and the enhancing activities depend on the physicochemical properties of both drug and enhancer (Barry, Citation2001; Zhao et al., Citation2013). In the molecular structure of IM, there are four hydrogen bond receptors. The hydroxyl group contained in the MT structure might form hydrogen bonds with the hydrogen bond receptors in IM (Du Plessis et al., Citation2001). The extensive interactions between IM and MT might increase the drug solubility in the donor solutions compared with the control group, as shown in , at the same time, it could create an obstacle to the permeation of IM into the skin SC which might be responsible for the reduction of apparent partition parameters. It also reminds us that drug properties should be taken into consideration while investigating the enhancing effect and mechanisms of enhancers. However, further investigation is required to prove this hypothesis, and the mechanisms of M-HEP will be detailed in the following investigations.
Partition of IM between SC and enhancer solutions
Improved partition of drugs into the SC has been reported following enhancer treatment by several investigators (Gao & Singh, Citation1998; Zhao & Singh, Citation1999), and it is considered as an important mechanism of penetration enhancers. The results of in vitro permeation experiment revealed that M-HEP exerted an enhancing effect might by increasing the partition of IM to the skin, and the partition experiment was presented here to confirm this hypothesis.
The 10% (w/w) concentration of enhancers was used in the partition experiment and the following investigation, since M-HEP exhibited the most remarkable enhancing effect on the in vitro permeation of IM at this concentration. Partition of IM into the SC from enhancer solution was shown in . The partition of IM from M-HEP solution to the SC sheets was significantly higher than that of the control whereas MT reduced the partition of IM into SC. The tendency was consistent with the results of in vitro permeation experiment that M-HEP increased the apparent partition parameter and MT decreased the apparent partition parameter. The higher partition of IM from the M-HEP solution to SC was probably due to the increased solubility of IM in SC lipids, and the higher solubility of IM in SC promoted its permeation. A lower partition in the presence of MT compared with that of the control could be attributed to the lower chemical potential of the drug in MT solution than that in the control solution as the same (sub-saturated) drug concentration was used. From the results of solubility determination (), the solubility of IM in MT solution was higher than that of the control. The chemical potential of the IM was lower in MT solution at the same drug concentration compared with control. Therefore, the lower leaving tendency of IM from the MT solution to SC resulted in a lower partition coefficient value.
Table 3. Partitioning of IM between SC and enhancer solution system (mean ± SE, n = 4).
SC organization alteration study using ATR-FTIR
ATR-FTIR studies were performed to gain further insight into the effect of enhancer on the biophysical properties of the SC. The ATR-FTIR spectra of the rat abdominal skin SC treated with 10% enhancer solutions for 1.5 h are presented in . The prominent peaks near 2850 and 2920 cm−1 result from the asymmetric CH2 and symmetric CH2 vibrations of long-chain hydrocarbons of lipids. The higher wavenumber shifts of the CH2 asymmetric stretching vibration are associated with an increase in the number of gauche conformers along the lipid hydrocarbon chains. ATR-FTIR results showed that the treatment of SC with 10% test enhancer solution produced a blue shift in the asymmetric and symmetric CH2 stretching peak positions in comparison with the control. This indicated that both M-HEP and MT strongly induced disorder of lipid lamellar structures.
The bands at 1650 and 1550 cm−1 are due to the amide I and amide II stretching vibrations of SC keratin (Tori & Tasumi, Citation1996). The amide I band arises from C = O stretching vibration and the amide II band from C–N stretching and N–H bending vibration. The frequencies of these two bands, especially amide I band, are sensitive and shift to higher or lower frequencies according to the change in the secondary structure of keratin in SC. In the spectra of , the position of amide II band after treatment with M-HEP or MT was similar with control. The variability of amide peak mainly existed among amide I bands. Based on the standard spectra of the amide I (Byler & Susi, Citation1986; Vaddi et al., Citation2002), the amide I band was analyzed to elucidate the changes of secondary structure of keratin in SC under the effects of enhancer. The ranges are assigned as: β-sheeting of 1620–1640 cm−1, random coiling of 1640–1650 cm−1, α-helix of 1650–1660 cm−1, and β-turning of 1660–1695 cm−1. Under the effect of the drug solution without enhancer (control), the main configuration of amide I was β-sheeting (1637.3 cm−1). M-HEP solution did not remarkably alter the configuration of keratin from that of the control. Upon treatment with MT, the wavenumber of amide I band increased to 1646.9 cm−1, revealing that the main secondary configuration of keratin was changed to random coiling from β-sheeting, and the decreased crosslinking density of keratin matrix would result in the increased enhancing effect of drug. However, this was only a secondary effect for the permeation of IM.
The percutaneous absorption of drug is a complex procedure. Although in the experiment of ATR-FTIR, both M-HEP and MT produced a blue shift in the asymmetric and symmetric CH2 stretching, for MT, prevention the partition of IM might played a main role in the permeation procedure of drug. Consequently, M-HEP showed a better enhance effect on the in vitro percutaneous absorption of IM than MT.
Transepidermal water loss
TEWL is an non-invasive bioengineering method which frequently used for assessing the effects of enhancers on the skin barrier (Green et al., Citation1988; Tanojo et al., Citation1997) or skin irritation (Fang et al., Citation2003). The skin barrier plays an important role in regulating water loss from the body, once damaged, more water can evaporate on the skin than in the case of an intact barrier. TEWL is actually the diffusion rate of water/water vapor across the skin based on the Fick’s law (Priot et al., Citation1997). Several investigators suggested that the high resistance of the SC to water flux is due to the extended multilamellar lipid domains present intercellular in the SC (Wertz & Downing, Citation1982; Elias, Citation1983). Potts & Francoeur (Citation1990) also concluded that water loss depends on conformational disorder in the lipid hydrocarbon chains. According to these hypotheses, if enhancers could interact with the SC and disturb the barrier function of SC, just as described in the ATR-FTIR study, an increasing TEWL value would be determined.
The measurements of TEWL after the topical applications with the test solutions on the rats’ abdominal skin are shown in . It was found that the interindividual variation of baseline TEWL values was high either by sites or by time which was also reported by many researchers (Blichmann & Serup, Citation1987; Boddé et al., Citation1991; Oestmann et al., Citation1993). Therefore, it is advisable to use baseline of each site itself to calculate the value of ΔTEWL. As can be seen from the results (), the influence of drug solution (control) which was obtained by suspending excess amount of IM in IPP on the control group was small and negligible. However, the treatment of M-HEP solution for 1.5 h caused a significant increase in TEWL on the sites in comparison with the sites treated with control (p < 0.05), yet the ΔTEWL was very consistently observed in all subjects. MT solution also increased the TEWL value of the skin by a ΔTEWL of 1.89 ± 0.11 g m−2 h−1. The increased TEWL value induced by M-HEP and MT solution revealed the macroscopic barrier perturbation of skin, and this result is consistent with the ATR-FTIR study. Compared with M-HEP group, the ability of MT solutions in increasing TEWL value was lower, which is also consistent with the results of in vitro permeation experiment.
Table 4. Enhancement value of TEWL after the application of drug solution with/without enhancer for 1.5 h (mean ± SE, n = 4, g m−2 h−1).
Reversibility study
In general, an ideal enhancer should be a safe compound, which can induce a temporary and reversible increase in skin permeability. Theoretically, because of the dynamics of the SC replacement mechanisms, almost any damage to the SC can be repaired, it is only a matter of time. However, some lipid solvents, such as hexane and high concentration of DMSO, would increase permeability irreversibly, some even caused permanent damage that can only be repaired by SC regeneration (Scheuplein & Ross, Citation1970). Therefore, the reversible effect of the enhancers was evaluated as a preliminary indicator of safety.
illustrated the reversible enhancement effect of M-HEP and MT on the in vitro permeation of IM through rat abdominal skin. At the recovery time of 0 h, i.e. the in vitro permeation of IM was started immediately after the enhancer was removed, the ER of MT was higher than the result of the in vitro permeation experiment mentioned above.
Figure 5. The reversible enhancement effect of M-HEP and MT on the in vitro permeation of IM through rat abdominal skin. Each point represented the mean ± SE (n = 4).
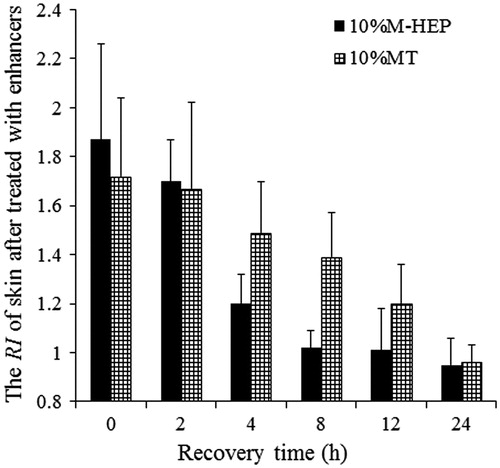
Two protocols are possible to show the potential activity of an enhancer (Duracher et al., Citation2009): (1) a skin pre-treatment is made with the selected enhancer; (2) without pre-treatment, the chosen enhancer is incorporated in the vehicle which is then applied with “in-use” conditions. Our research coincidentally used the two protocols, and the results showed that the potential activity of enhancer may be different through the two methods, and the key agent might be the interaction (hydrogen bond) between IM and MT.
As we can see in , the permeability of the skin barrier was restored in 8 h after M-HEP was removed, whereas pre-treated with MT for 1.5 h, the skin barrier was fully recover in 24 h. The mechanism for reversible enhancement of enhancer has been discussed in several researches (Zhao et al., Citation2001; Kang et al., Citation2007), but it is still not well explained. Kang et al. (Citation2007) reported that the mechanism for the reversible enhancement of enhancer could be attributed to the insertion of these enhancers within the SC intercellular lipid lamella, once the enhancers were removed, the packing of the lipids would revert back to its original alignment. However, the author did not mention about the detail procedure of enhancer removing. Zhao et al. (Citation2001) pointed out that actively metabolizing skin repairs itself within the given time period or is capable of removing the enhancer through some enzymatic or other neutralizing pathway. Under the conditions of our in vitro experiments, the skin lacked of circulation and was metabolically compromised. Diffusion might be one factor to account for the barrier recovery. After removal of enhancer, the enhancer concentration in the skin was higher than that in donor or receiver solution. The concentration gradient between solution and SC was driving the enhancer to leave the SC lipids and allow the lipids to revert back to its original alignment. As MT could also interact with the keratin in the SC, which was found in the ATR-FTIR study, the recovery time of MT-treated skin was longer.
Conclusion
In the present work, the mechanisms and reversible enhancement effect of M-HEP on the transdermal permeation of IM was investigated. It was found that M-HEP could enhance the IM permeation by increasing the drug partitioning into the SC from enhancer solution and disturbing the organized structure of SC lipids which caused the perturbation of the macroscopic barrier properties of the skin. And the reversibility of M-HEP was better than MT. M-HEP could be a potential candidate for enhancing the transdermal absorption of lipophilic drug. These results might provide important foundation information for further studies of the transdermal enhancement and mechanisms of M-HEP.
Declaration of interest
The authors report no conflicts of interest. The authors alone are responsible for the content and writing of the paper.
References
- Barry BW. (2001). Novel mechanisms and devices to enable successful transdermal drug delivery. Eur J Pharm Sci 14:101–14
- Bhatia KS, Singh J. (1998). Mechanism of transport enhancement of LHRH through porcine epidermis by terpenes and iontophoresis: permeability and lipid extraction studies. Pharm Res 15:1857–62
- Blichmann CW, Serup J. (1987). Reproducibility and variability of transepidermal water loss measurement. Studies on the servo med evaporimeter. Acta Derm Venereol 67:206–10
- Boddé H, Pechthold L, De Haan F. (1991). A large scale, non-invasive in vivo screening of the human skin barrier recording ATR-FTIR-spectra, TEWL, skin impedance and temperature. Proc Int Symp Control Rel Bioact Mater 18:311–2
- Bouwstra JA, Ponec M. (2006). The skin barrier in healthy and diseased state. Biochim Biophys Acta (BBA) – Biomemb 1758:2080–95
- Byler DM, Susi H. (1986). Examination of the secondary structure of proteins by deconvolved FTIR spectra. Biopolymers 25:469–87
- Dias M, Raghavan SL, Hadgraft J. (2001). ATR-FTIR spectroscopic investigations on the effect of solvents on the permeation of benzoic acid and salicylic acid through silicone membranes. Int J Pharm 216:51–9
- Du Plessis J, Pugh WJ, Judefeind A, et al. (2001). The effect of hydrogen bonding on diffusion across model membranes: consideration of the number of H-bonding groups. Eur J Pharm Sci 13:135–41
- Duracher L, Blasco L, Hubaud JC, et al. (2009). The influence of alcohol, propylene glycol and 1,2-pentanediol on the permeability of hydrophilic model drug through excised pig skin. Int J Pharm 374:39–45
- Elias PM. (1983). Epidermal lipids, barrier function, and desquamation. J Invest Dermatol 80:44s–49s
- Fang JY, Hwang TL, Fang CL, et al. (2003). In vitro and in vivo evaluations of the efficacy and safety of skin permeation enhancers using flurbiprofen as a model drug. Int J Pharm 255:153–66
- Gao S, Singh J. (1998). In vitro percutaneous absorption enhancement of a lipophilic drug tamoxifen by terpenes. J Control Release 51:193–9
- Green PG, Guy RH, Hadgraft J. (1988). In vitro and in vivo enhancement of skin permeation with oleic and lauric acids. Int J Pharm 48:103–11
- Kang L, Poh AL, Fan SK, et al. (2007). Reversible effects of permeation enhancers on human skin. Eur J Pharm Biopharm 67:149–155
- Karande P. (2005). Principles of penetration. Nat Rev Drug Discov 4:372–3
- Karande P, Jain A, Ergun K, et al. (2005). Design principles of chemical penetration enhancers for transdermal drug delivery. Proc Natl Acad Sci USA 102:4688–93
- Marjukka Suhonen T, Bouwstra JA, Urtti A. (1999). Chemical enhancement of percutaneous absorption in relation to stratum corneum structural alterations. J Control Release 59:149–61
- Nakamura Y, Takayama K, Higashiyama K, et al. (1996). Promoting effect of O-ethylmenthol on the percutaneous absorption of ketoprofen. Int J Pharm 145:29–36
- Oestmann E, Lavrijsen APM, Hermans J, et al. (1993). Skin barrier function in healthy volunteers as assessed by transepidermal water loss and vascular response to hexyl nicotinate: intra- and inter-individual variability. Br J Dermatol 128:130–6
- Pinnagoda J, Tupker RA, Agner T, et al. (1990). Guidelines of the transepidermal water loss (TEWL) measurement: a report from the standardization group of the European society of contact dermatitis. Contact Dermatitis 22:164–78
- Potts RO, Francoeur ML. (1990). Lipid biophysics of water loss through the skin. Proc Natl Acad Sci USA 87:3871–3
- Priot F, Berardesca E, Kalia YN, et al. (1997). Stratum corneum thickness and apparent water diffusivity facile and noninvasive quantitation in vivo. Pharm Res 15:492–4
- Rogiers V. (2001). EEMCO guidance for the assessment of transepidermal water loss in cosmetic sciences. Skin Pharmacol Appl Skin Physiol 14:117–28
- Scheuplein R, Ross L. (1970). Effects of surfactants and solvents on the permeability of epidermis. J Soc Cosmet Chem 21:853–73
- Tanojo H, Jungiger HE, Bodde HE. (1997). In vivo human skin permeability enhancement by oleic acid transepidermal water loss and fourier-transform infrared spectroscopy. J Controlled Release 47:31–9
- Tori H, Tasumi M. (1996). Theoretical analyses of the amide I infrared bands of globular proteins. In: Mantsch HH, Chapman D, eds. Infrared spectroscopy of biomolecules. New York: Wiley-Liss, 1–18
- Vaddi HK, Ho PC, Chan SY. (2002). Terpenes in propylene glycol as skin-penetration enhancers permeation and partition of haloperidol: fourier transform infrared spectroscopy, and differential scanning calorimetry. J Pharm Sci 91:1639–51
- Wertz P, Downing D. (1982). Glycolipids in mammalian epidermis: structure and function in the water barrier. Science 217:1261–2
- Williams AC, Barry BW. (2004). Penetration enhancers. Adv Drug Deliv Rev 56:603–18
- Zhao K, Singh J. (1999). In vitro percutaneous absorption enhancement of propranolol hydrochloride through porcine epidermis by terpenes ethanol. J Control Release 62:359–66
- Zhao K, Singh S, Singh J. (2001). Effect of menthone on the in vitro percutaneous absorption of tamoxifen and skin reversibility. Int J Pharm 219:177–81
- Zhao L, Fang L, Xu Y, et al. (2008). Transdermal delivery of penetrants with differing lipophilicities using O-acylmenthol derivatives as penetration enhancers. Eur J Pharm Biopharm 69:199–213
- Zhao N, Cun D, Li W, et al. (2013). In vitro percutaneous absorption enhancement of granisetron by chemical penetration enhancers. Drug Dev Ind Pharm 39:561–8