Abstract
Context: Tuberculosis (TB) is a chronic infectious disease with increasing incidence of drug resistance. Oral treatment for TB and multidrug resistance (MDR)-TB can have serious side effects. The causative agent of TB, Mycobacterium tuberculosis, resides in alveolar macrophages (AM). Pulmonary administration of anti-TB drugs can help in delivery of high concentration to AM. The ability of AM to phagocytose can also be utilized to generate mycobactericidal nitric oxide (NO) to improve efficacy of anti-TB drugs.
Objective: To compare the uptake of rifampicin (RIF) by AM post oral and pulmonary administration of RIF microparticles (RM) and to compare hepatotoxicity and phagocytosis activity.
Materials and Methods: RM were produced by spray drying process. RM were administered to rats through oral as well as intratracheal route. The uptake of RIF by AM and liver was measured. NO was measured in bronchoalveolar lavage (BAL) fluid. SGOT and SGPT levels were measured in serum.
Results: Significantly higher (p < 0.05) concentration of RIF was found in AM post intratracheal administration. NO production was also significantly higher but less than toxic level. SGOT and SGPT levels as well as uptake of RIF by liver were indicative of no hepatotoxicity post intratracheal administration.
Discussion: Phagocytosis of RM post intratracheal administration leads to significantly higher drug level in AM as well as production of significantly higher levels of NO.
Conclusion: The administration of RM as dry powder inhalation (DPI) formulation may reduce treatment time of TB and chances of drug resistance TB.
Introduction
Tuberculosis (TB) is a chronic infectious disease. Treatment of TB needs oral administration of isoniazid, rifampicin, pyrazinamide and ethambutol for 2 months followed by oral administration of isoniazid and rifampicin for another 4–6 months (Maartens & Wilkinson, Citation2007). The Global TB Report – 2012 published by World Health Organization (WHO) showed an increasing incidence of drug resistance. As per WHO guidelines, patients with drug resistance TB need to be treated for a period of 18–24 months using second-line anti-TB drugs. Poor patient compliance due to the longer treatment regimen is a major challenge in the treatment of TB (Suarez et al., Citation2001; Du Toit et al., Citation2006). Poor patient compliance also creates new challenge of drug resistance TB (Suarez et al., Citation2001; Du Toit et al., Citation2006; Sosnik et al., Citation2010). Another challenge associated with the treatment of TB is the toxicity of anti-TB drugs (Maartens & Wilkinson, Citation2007). Interest of researchers is directed toward inventing new drugs that are potent and less toxic (Duncan & Barry, Citation2004). However, it is evident that no new drug is approved for the treatment of TB since last 30 years (Duncan & Barry, Citation2004).
Drug targeting through pulmonary route of administration has remained successful to minimize toxicity in the treatment of cancer (Zhang et al., Citation2008; Aillon et al., Citation2009; Bailey & Berkland, Citation2009; Xie et al., Citation2010). This approach has also helped in improving patient compliance (Muttil et al., Citation2007; Sosnik et al., Citation2010; Roa et al., Citation2011). Drugs when administered through pulmonary route can provide higher concentration at local site. The causative agent of TB, Mycobacterium tuberculosis, resides in alveolar macrophages (AM) (Kleinnijenhuis et al., Citation2011; Dube et al., Citation2012). So, pulmonary delivery of anti-TB drugs may help in targeting of drugs to M. tuberculosis residing in AM (Du Toit et al., Citation2006; Misra et al., Citation2011). This in turn may help in reducing toxicity of anti-TB drugs and shorten the treatment period and may improve patient compliance (Suarez et al., Citation2001; Sen et al., Citation2003; Pandey & Khuller, Citation2005; Kaur et al., Citation2008).
Rifampicin (RIF) was selected as anti-TB drug in this investigation. RIF is administered at dose of 10 mg/kg body weight. The maximum dose is 600 mg per day as per WHO guidelines. The bioavailability of RIF post oral administration is 50% and it undergoes degradation in gastrointestinal tract in presence of isoniazid (Shishoo et al., Citation1999; Gohel & Sarvaiya, Citation2007). The major side effect associated with RIF is hepatotoxicity. So, it may be anticipated that pulmonary administration of RIF may improve patient compliance through reduction in dose and hepatotoxicity and shortening of treatment period.
Several studies have reported targeting of AM after administration of dry powder inhalation (DPI) formulation containing polymeric microparticles loaded with RIF (O'Hara & Hickey, Citation2000; Coowanitwong et al., Citation2008; Ohashi et al., Citation2009; Ober et al., Citation2012). Few reports are also available regarding respirable form of RIF (Son & Mcconville, Citation2011; Chan et al., Citation2013). These reports lack in the macrophage activation and toxicity status. In this investigation, Rifampicin microparticles (RMs) were prepared through spray drying process. The purpose of this work is to evaluate and compare the uptake of rifampicin by AM in rats post oral and pulmonary administration of RM. The purpose is also to evaluate the macrophage activation status for phagocytosis and to compare the hepatotoxicity.
Materials and methods
Chemicals
Rifampicin (RIF) was gifted by Microlabs, India. Trypan blue, Percoll™, N- (1-naphthyl)-ethylenediamine dihydrochloride (NED) were purchased from Sigma Aldrich, St Louis, MO. Sulfanilamide, phosphoric acid, methanol, dichloromethane (DCM), sodium taurocholate, lecithin, pepsin, sodium chloride, hydrochloric acid, sodium hydroxide, magnesium chloride, disodium hydrogen phosphate, sodium sulfate, calcium chloride dehydrate, sodium citrate dehydrate, citric acid, glycine, sodium tartrate dehydrate, sodium lactate, sodium pyruvate and phosphate buffer saline pH 7.4 (PBS) were purchased from Merck Chemicals, Bengaluru, India. Serum glutamate oxaloacetate transferase (SGOT) – serum glutamate pyruvate transferase (SGPT) kits were purchased from Crest Biosystems, Verna Goa, India. All the chemicals and ingredients utilized for study were of AR grade. Double distilled water was utilized throughout the study.
Reagents
Griess Reagent: Equal volumes of sulfanilamide (2%w/v) in phosphoric acid (5%v/v) and NED (0.2%w/v) in water were mixed just before use.
Percoll™ (80%v/v): Percoll™ (8 mL) was diluted to 10 mL using PBS.
Preparation of rifampicin microparticles (RM)
RM were prepared as per the described procedure with few modifications (Son & McConville, Citation2011). The solution of RIF in DCM (5%w/v) was spray dried using spray dryer (LU-222 advanced, Labultima, Mumbai, India) at inlet temperature 45 °C, aspiration 45 Nm3/hr and feed rate of 1 mL/min. The powder was collected from high-efficiency cyclone separator attached to the spray dryer. The particles were observed using high-resolution microscope (Axio Lab.A1, Carl Zeiss MicroImaging, GmbH and Gottingen) and images were taken. Particle size was measured through image analysis process performed using software [Biovis Image Plus (P+) V4.56 (Expert Vision Labs Pvt. Ltd., Mumbai, India)]. The mass median aerodynamic diameter (MMAD), respirable fine particle fraction (FPF<4 µm) and emitted dose (%ED) were determined by cascade impaction study using 8-Stage Anderson Cascade Impactor (ACI, Copley Scientific Ltd., UK).
In vitro drug dissolution study
USP Type II paddle apparatus (TDT-08 L, Electrolab, Mumbai, India) was used at a stirring speed of 150 rpm for in vitro drug release study in simulated phagosomal fluid pH 4.5 (Muttil et al., Citation2007; Marques et al., Citation2011) and in simulated gastric fluid pH 1.2 (Marques et al., Citation2011). Temperature of dissolution media was maintained at 37 ± 0.5 °C throughout the study. A dialysis membrane (Himedia, molecular weight cutoff >900 kDa) was cut into equal pieces, approximate size 6 cm × 2.5 cm, and pretreated as suggested by the manufacturer. RM equivalent to 5 mg of RIF were dispersed in 1 mL of each media. The dispersion was filled in the pouch prepared using the pretreated dialysis membrane and the pouch was sealed with clips. The pouch was attached to the paddle of the apparatus using rubber bands wound over the clips. The sample of dissolution medium (5 mL) was drawn at an interval of 5, 10, 15, 30, 45, 60, 90 and 120 min for analysis. At each time point, 5 mL of dissolution medium was replenished in the bath. The sample was filtered through 0.22-μm cellulose acetate membrane filter. Rifampicin was estimated by UV spectrophotometric method after measuring the absorbance at 333 nm of a suitably diluted filtrate. Each sample was tested in triplicate.
In vivo animal study
The Institutional Animal Ethics Committee (IAEC), registered with the Government of India approved the protocol (RPCP/IAEC/2012-2013/R-12) for animal experiments. Albino rats (Wistar) were taken for the study. They were divided in two groups; each group was consisted of six rats weighing between 300 and 340 g. Each rat in group I and group II was subjected to following treatment:
Group I: From the tail vein of each rat, 0.5 mL of blood was collected, centrifuged at 3000 rpm and 4 °C for 10 min and serum was separated and stored at −80 °C till further analysis. Each rat in group was administered RM at a RIF dose of 10 mg per kg body weight via oral route, as a dispersion (1.8 mg/mL) prepared in 10%w/v carboxymethyl cellulose in water. The rats were anesthetized by intraperitoneal administration of phenobarbital at dose of 50 mg per kg body weight and trachea was cannulated using butterfly cannula. Bronchoalveolar lavage (BAL) fluid was collected from each animal at an interval of 15 min, 30 min, 60 min, 90 min up to 240 min. For collection of BAL fluid at each time point, 2 mL of PBS was first introduced through cannula and then BAL fluid was sampled gradually at very slow rate to ensure that no injury occurs to the lungs.
Each rat was sacrificed after the last sample of BAL fluid was collected. Immediately, 1 mL of blood was collected, centrifuged at 3000 rpm and 4 °C for 10 min and serum was separated and stored at −80 °C till further analysis. The liver was isolated. The total weight of liver was measured.
Group II: From the tail vein of each rat, 0.5 mL of blood was collected, centrifuged at 3000 rpm and 4 °C for 10 min and serum was separated and stored at −80 °C till further analysis. Each rat in the group was anesthetized by intraperitoneal administration of pentobarbital at dose of 50 mg per kg body weight. The trachea was cannulated using butterfly cannula and 1 mL dispersion of RM in PBS was administered to deliver a dose of 5 mg of RIF per kg of body weight (Muttil et al., Citation2007). For collection of BAL fluid, blood and isolation of liver, procedure as outlined above was followed.
RIF estimation in AM post pulmonary administration
The AM containing RM was separated from BAL fluid as per the described method (Ohashi et al., Citation2009). The BAL fluid was centrifuged at 20 000 rpm and 4 °C for 10 min to obtain a cell pellet. The supernatant was utilized for nitric oxide (NO) determination in the form of nitrite as per procedure outlined in the section “Measurement of NO as nitrite”. The pellet was dispersed in 1 mL of PBS. The cell suspension (500 µL) was layered over 500 µL of 80% Percoll™ present in 1.5 mL capacity eppendorf tube. The centrifugation was done at 20 000 rpm and 4 °C for 10 min. The RM that have not been internalized in AM migrate to lower Percoll™ layer whereas AM in which drug is not internalized remain as upper layer and AM containing RM form middle layer (Vyas et al., Citation2004; Coowanitwong et al., Citation2008; Verma et al., Citation2008; Zhang & Kawakami, Citation2010). The middle layer was collected and mixed with methanol (1 mL), centrifuged at 20 000 rpm and 4 °C for 10 min. Supernatant was filtered through a 0.22-μm cellulose acetate membrane filter. The absorbance of filtrate was measured at 333 nm using UV spectrophotometer (UV-1800 240 V, Shimadzu, Japan).
RIF estimation in AM post oral administration
The BAL fluid was centrifuged at 20 000 rpm and 4 °C for 10 min to obtain a cell pellet. The supernatant was utilized for NO determination in the form of nitrite as per the procedure outlined in the section “Measurement of NO as nitrite”. The pellet was collected and mixed with methanol (1 mL), centrifuged at 20 000 rpm and 4 °C for 10 min. Supernatant was filtered through a 0.22 -μm cellulose acetate membrane filter. The absorbance of filtrate was measured at 333 nm using UV spectrophotometer (UV-1800 240 V, Shimadzu, Japan).
RIF estimation in liver homogenate
The bigger lob of liver was used to prepare liver homogenate (20%w/v) in PBS (Sung et al., Citation2009). The homogenate was prepared using tissue homogenizer (RQ-127-A, Remi Elektrotechnik Ltd., Goregaon, India). The liver homogenate was centrifuged at 20 000 rpm and 4 °C for 10 min. The supernatant was treated with equal volume of methanol to precipitate the cell metrics. Centrifugation was again carried out at 20 000 rpm and 4 °C for 10 min. The absorbance of RIF in the supernatant was measured at 333 nm using UV spectrophotometer (UV-1800 240 V, Shimadzu, Japan).
Study of liver function test
The SGOT and SGPT levels were determined for liver function test. The serum samples were evaluated for SGOT and SGPT levels using SGOT kit and SGPT kit.
Measurement of NO as nitrite
NO produced in AM is instantly oxidized to nitrite (Ohashi et al., Citation2009). Hence, the NO was determined in terms of nitrite using Griess reagent (Rojanarat et al., Citation2011). The supernatants collected as per procedure outlined in section the “RIF estimation in AM post pulmonary administration” and “RIF estimation in AM post oral administration” were utilized for nitrite measurement. Equal volume of supernatant and Griess reagent were mixed and kept aside for 10 min. The absorbance was measured at 540 nm using UV-visible spectrophotometer (UV-1800 240 V, Shimadzu, Japan).
Statistical analysis
Data are presented as mean ± SD. T-test was employed to determine the differences between treated groups of animals. A significance level of p < 0.05 was considered statistically significant.
Results and discussion
Validation of analytical method for estimation of RIF in AM and liver
The UV spectrophotometric method used for estimation of RIF was validated with exclusion of interferences and with following details:
Limit of Detection (LOD): 0.741 mcg
Limit of Quantification (LOQ): 2.26 mcg
Accuracy [(Relative Mean Error (RME)(%)]: 0.05–0.3
Precision [Relative Standard Deviation (RSD)(%)]: 0.16–1.1
Linear Range: 2–25 mcg/mL
Correlation coefficient (r2): 0.997
Particle size and mass median aerodynamic diameter
RM with the median particle size of 3.6 ± 0.77 µm (560 particles) were obtained through spray drying process. Through the cascade impaction study, following parameters were found: mass median aerodynamic diameter (MMAD): 2.5 ± 0.061 µm; respirable fine particle fraction (FPF<4 µm): 77.85 ± 1.05%; and emitted dose (%ED): 58.68 ± 0.84%. These characteristics indicate that the RM were suitable for administration as a dry powder inhalation as well as for deep lung deposition (Chow et al., Citation2007; Muttil et al., Citation2007).
In vitro drug dissolution study
The results for in vitro drug release study are shown in . It is evident that 98% of RIF gets dissolved in simulated gastric fluid pH 1.2 within 120 min; however, only 53.35% of RIF gets dissolved in the same time period in simulated phagosomal fluid pH 4.5. Although rate of dissolution of RIF in simulated gastric fluid pH 1.2 is higher, uptake of RIF by AM is lower post oral administration (). This may be due to significant uptake of RIF by liver post oral administration ().
Figure 1. RIF dissolution in simulated gastric fluid pH 1.2 and in simulated phagosomal fluid pH 4.5.
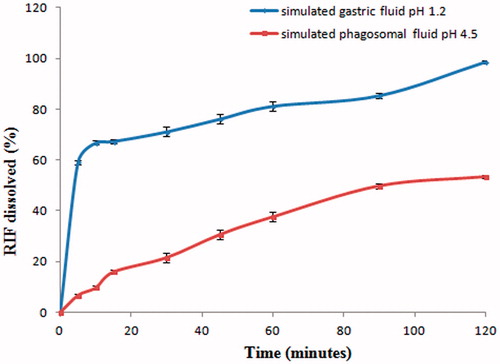
Table 1. RIF uptake in liver after oral administration and intratracheal administration.
Uptake of RIF by AM
The uptake of RIF by AM is shown in . RIF uptake by AM was found significantly higher (p < 0.05 at every sampling point) after intratracheal administration. The deep lung deposition of RM brings them in close proximity with AM and this in turn may lead to internalization of RIF through phagocytosis (Sharma et al., Citation2007).
Significantly lower uptake of RIF by AM post oral administration may be attributed to the fact that uptake of RIF by liver is very high (). Hence, only a small fraction of the administered dose is available for distribution to AM through diffusion process after oral administration of RM.
Phagocytosis activity and generation of NO
The levels of NO in BAL fluid are shown in . The presence of NO in BAL fluid is an indication of uptake of RM by AM through phagocytosis and activation of phagolysosome fusion process (Sharma et al., Citation2007). For the treatment of TB, it is important to stimulate the phagocytes for favorable therapeutic outcome (Young, Citation2001). Phagocytosis is accompanied with activation of key macrophage enzymes such as the inducible nitric oxide synthase (iNOS) and phagocyte oxidase (phox) or NADPH oxidase complex. Such activation leads to accumulation of reactive nitrogen intermediates (RNIs) in forming phagosome (Marcinkiewicz, Citation1997; Ricciardolo et al., Citation2004; Kolodziej & Kiderlen, Citation2007). These intracellular RNIs are directly mycobactericidal (Nathan & Hibbs, Citation1991, Ricciardolo et al., Citation2004; Hirota et al., Citation2008).
NO in concentration of 4–5 µM is toxic to lung epithelial cells (Murphy, Citation1999). In this investigation, the maximum concentration of NO found was 0.0069 µM after 30 min of post intratracheal administration. Thus, it can be inferred that levels of NO generated are not toxic.
Significant uptake of RIF by AM as well as generation of mycobactericidal NO is an indication to infer that efficacy of RIF may significantly improve post intratracheal administration of RM.
Liver toxicity
Increased levels of SGOT and SGPT are observed in conditions such as hepatitis, cirrhosis, obstructive jaundice and other hepatic diseases (Lal et al., Citation1972; Carlisle & Galambos, Citation1979). Significant increase in SGOT levels and SGPT levels were found post oral administration of RM ( and , respectively). In addition, the uptake of RIF by liver is 99.67% of the dose after 4 h of oral administration (). Thus, it can be inferred that hepatotoxicity may occur post oral administration of RM.
Table 2. SGOT levels.
Table 3. SGPT levels.
RIF uptake by liver post intratracheal administration is significantly low compared to oral administration. No significant difference in level of SGOT and SGPT were found when levels were measured before and after intratracheal administration of RM. Thus, it can also be inferred that post intratracheal administration of RM, the hepatotoxicity may not occur.
Conclusion
RM suitable for deep lung deposition post pulmonary administration may provide improved efficacy over oral administration through significantly higher uptake as well as activation of AM to produce mycobactericidal NO. The liver toxicity of this product may be almost negligible as indicated by lower liver uptake and nonsignificant levels of SGOT and SGPT. RM formulated as dry powder inhalation may improve patient compliance through improved efficacy and reduced hepatotoxicity which in turn may reduce treatment period as well as chances of drug-resistant tuberculosis.
Declaration of interest
The authors report no declaration of interest.
Acknowledgements
Authors are grateful to Microlabs, India for providing gratis sample of rifampicin. Sonali Dalwadi is grateful to Charotar University of Science and Technology for providing research fellowship.
References
- Aillon K L, Xie Y, El-Gendy N, et al. (2009). Effects of nanomaterial physicochemical properties on in vivo toxicity. Adv Drug Deliv Rev 61:457–66
- Bailey MM, Berkland CJ. (2009). Nanoparticle formulations in pulmonary drug delivery. Med Res Rev 29:196–212
- Carlisle R, Galambos JT. (1979). The relationship between conventional liver tests, quantitative function tests, and histopathology in cirrhosis. Dig Dis Sci 24:358–62
- Chan JGY, Chan H-K, Prestidge CA, et al. (2013). A novel dry powder inhalable formulation incorporating three first-line anti-tubercular antibiotics. Eur J Pharm Biopharm 83:285–92
- Chow AL, Tong HY, Chattopadhyay P, Shekunov B. (2007). Particle engineering for pulmonary drug delivery. Pharm Res 24:411–37
- Coowanitwong I, Arya V, Kulvanich P, Hochhaus GN. (2008). Slow release formulations of inhaled rifampin. AAPS J 10:342–8
- Du Toit LC, Pillay V, Danckwerts MP. (2006). Tuberculosis chemotherapy: current drug delivery approaches. Respir Res 7:118--35
- Dube D, Agrawal GP, Vyas SP. (2012). Tuberculosis: from molecular pathogenesis to effective drug carrier design. Drug Discov Today 17:760–73
- Duncan K, Barry III CE. (2004). Prospects for new antitubercular drugs. Curr Opin Microbiol 7:460–5
- Gohel MC, Sarvaiya KG. (2007). A novel solid dosage form of rifampicin and isoniazid with improved functionality. AAPS PharmSciTech 8:E133–9
- Hirota K, Tomoda K, Inagawa H, et al. (2008). Stimulation of phagocytic activity of alveolar macrophages toward artificial microspheres by infection with mycobacteria. Pharm Res 25:1420–30
- Kaur J, Muttil P, Verma RK, et al. (2008). A hand-held apparatus for nose-only exposure of mice to inhalable microparticles as a dry powder inhalation targeting lung and airway macrophages. Eur J Pharm Sci 34:56–65
- Kleinnijenhuis J, Oosting M, Joosten LA, et al. (2011). Innate immune recognition of Mycobacterium tuberculosis. Clin Dev Immunol 11:1--12
- Kolodziej H, Kiderlen AF. (2007). In vitro evaluation of antibacterial and immunomodulatory activities of Pelargonium reniforme, Pelargonium sidoides and the related herbal drug preparation EPs 7630. Phytomedicine 14:18--26
- Lal S, Singhal S, Burley D, Crossley G. (1972). Effect of rifampicin and isoniazid on liver function. BMJ 1:148--50
- Maartens G, Wilkinson RJ. (2007). Tuberculosis. Lancet 370:2030–43
- Marcinkiewicz J. (1997). Nitric oxide and antimicrobial activity of reactive oxygen intermediates. Immunopharmacology 37:35–41
- Marques MR, Loebenberg R, Almukainzi M. (2011). Simulated biological fluids with possible application in dissolution testing. Dissolution Technol 18:15–28
- Misra A, Hickey AJ, Rossi C, et al. (2011). Inhaled drug therapy for treatment of tuberculosis. Tuberculosis 91:71–81
- Murphy MP. (1999). Nitric oxide and cell death. Biochim Biophys Acta 1411:401–14
- Muttil P, Kaur J, Kumar K, et al. (2007). Inhalable microparticles containing large payload of anti-tuberculosis drugs. Eur J Pharm Sci 32:140–50
- Nathan CF, Hibbs Jr JB. (1991). Role of nitric oxide synthesis in macrophage antimicrobial activity. Curr Opin Immunol 3:65–70
- O'hara P, Hickey AJ. (2000). Respirable PLGA microspheres containing rifampicin for the treatment of tuberculosis: manufacture and characterization. Pharm Res 17:955–61
- Ober CA, Kalombo L, Swai H, Gupta RB. (2012). Preparation of rifampicin/lactose microparticle composites by a supercritical antisolvent-drug excipient mixing technique for inhalation delivery. Powder Technol 236:132–8
- Ohashi K, Kabasawa T, Ozeki T, Okada H. (2009). One-step preparation of rifampicin/poly (lactic-co-glycolic acid) nanoparticle-containing mannitol microspheres using a four-fluid nozzle spray drier for inhalation therapy of tuberculosis. J Control Release 135:19–24
- Pandey R, Khuller GK. (2005). Solid lipid particle-based inhalable sustained drug delivery system against experimental tuberculosis. Tuberculosis 85:227–34
- Ricciardolo FL, Sterk PJ, Gaston B, Folkerts G. (2004). Nitric oxide in health and disease of the respiratory system. Physiol Rev 84:731–65
- Roa WH, Azarmi S, Al-Hallak MHDK, et al. (2011). Inhalable nanoparticles, a non-invasive approach to treat lung cancer in a mouse model. J Control Release 150:49–55
- Rojanarat W, Changsan N, Tawithong E, et al. (2011). Isoniazid proliposome powders for inhalation preparation, characterization and cell culture studies. Int J Mol Sci 12:4414–34
- Sen H, Jayanthi S, Sinha R, et al. (2003). Inhalable biodegradable microparticles for target-specific drug delivery in tuberculosis and a process thereof. U.S. Patent Application 10/685,567
- Sharma R, Muttil P, Yadav AB, et al. (2007). Uptake of inhalable microparticles affects defence responses of macrophages infected with Mycobacterium tuberculosis H37Ra. J Antimicrob Chemother 59:499–506
- Shishoo CJ, Shah SA, Rathod IS, et al. (1999). Stability of rifampicin in dissolution medium in presence of isoniazid. Int J Pharm 190:109–23
- Son Y-J, Mcconville JT. (2011). A new respirable form of rifampicin. Eur J Pharma Biopharm 78:366–76
- Sosnik A, Carcaboso ÃNM, Glisoni RJ, et al. (2010). New old challenges in tuberculosis: potentially effective nanotechnologies in drug delivery. Adv Drug Deliv Rev 62:547–59
- Suarez S, O'hara P, Kazantseva M, et al. (2001). Respirable PLGA microspheres containing rifampicin for the treatment of tuberculosis: screening in an infectious disease model. Pharm Res 18:1315–19
- Sung JC, Padilla DJ, Garcia-Contreras L, et al. (2009). Formulation and pharmacokinetics of self-assembled rifampicin nanoparticle systems for pulmonary delivery. Pharm Res 26:1847–55
- Verma RK, Kaur J, Kumar K, et al. (2008). Intracellular time course, pharmacokinetics, and biodistribution of isoniazid and rifabutin following pulmonary delivery of inhalable microparticles to mice. Antimicrob Agents Chemother 52:3195–201
- Vyas S, Kannan M, Jain S, et al. (2004). Design of liposomal aerosols for improved delivery of rifampicin to alveolar macrophages. Int J Pharm 269:37–49
- Xie Y, Aillon KL, Cai S, et al. (2010). Pulmonary delivery of cisplatin-hyaluronan conjugates via endotracheal instillation for the treatment of lung cancer. Int J Pharm 392:156--63
- Young DB. (2001). Stimulate the phagocytes. Tuberculosis (Edinb) 81:257–8
- Zhang S, Kawakami K. (2010). One-step preparation of chitosan solid nanoparticles by electrospray deposition. Int J Pharm 397:211–17
- Zhang Y, Li A, Wang Z, et al. (2008). Antimetastatic activities of pegylated liposomal doxorubicin in a murine metastatic lung cancer model. J Drug Target 16:679–87