Abstract
Elastin-like polypeptides (ELPs) are large molecular weight biopolymers. They have been widely studied as macromolecular carriers for targeted delivery of drugs. The aim of the present article is to review the available information on ELPs (including our recent investigations), their properties, drug delivery applications to tumor sites and future perspectives. This review also provides information on the use of short synthetic ELPs for making ELP-drug conjugates, for targeted delivery of anticancer drugs. In the present review we also focus on the point that short ELPs can also be used for targeting anticancer drugs to tumor sites as they behave similar to long ELPs regarding their capacity to undergo inverse temperature transition (ITT) behavior.
Introduction
Elastin, the elastomeric protein, is one of the main components of the extracellular matrix which provides structural integrity to the tissues and organs of the body. This highly cross-linked and insoluble protein is the essential element of elastic fibers, which induce elasticity to the tissues of lung, skin and arteries. These elastin fibers form the internal core, which is interspersed with non-elastin components, namely microfibrils that includes fibrillins, fibulins and microfibril associated glycoproteins (Vrhovski & Weiss, Citation1998). Nearly 90% of the elastic fibre consists of elastin protein, which is a polymer of the monomeric precursor, tropoelastin and is the dominant contributor to fibre elasticity (Muiznieks et al., Citation2010). Tropoelastin is the fundamental building component of all elastins. The most important feature of the primary tropoelastin sequence is the distinct arrangement of alternating hydrophobic and cross-linking domains which is highly conserved in elastins from all species (Chung et al., Citation2006; He et al., Citation2007). Further, tropoelastin has the ability to self-assemble under physiological conditions and exhibit an inverse temperature transition (ITT) behavior. This specific property has led to the development of a new class of polypeptides, namely elastin-like polypeptides (ELPs) that mimic elastin in its composition.
In recent years the use of biopolymer-based elastin and elastin-derived molecules have been increasingly investigated for their application in biomedical and drug delivery systems due to their remarkable properties such as elasticity, self-assembly, long-term stability and biological activity. Further, elastin can be applied for biomedical applications in various forms, including insoluble elastin fibres, hydrolysed soluble elastin, recombinant tropoelastin, repeats of synthetic peptide sequences, namely elastin-like polypeptides (ELPs) and as block copolymers of elastin (Daamen et al., Citation2007).
The origin and main properties of ELPs
Elastin-like polypeptides are genetically engineered pentapetide repeats of Val-Pro-Gly-Xaa-Gly amino acids, (VPGXaaG)n, where Xaa is a guest residue (except Proline) and n may vary from 10 to 200 (Meyer et al., Citation2001a; Raucher & Chilkoti, Citation2001). The repetitive ELP phenomenon was inspired from W4 domain of human tropoelastin that consists of repetitive VPGG, VPGVG and APGVGV peptides (Rapaka & Urry, Citation1978; Jensen et al., Citation2000; Toonkool et al., Citation2001).
Elastin-like polypeptides have several attractive features for biomedical applications. They are temperature sensitive biopolymers and undergo an ITT behavior in response to temperature change (Urry, Citation1992; Urry, Citation1997; Li et al., Citation2001). In other words, they are soluble in aqueous solutions at temperatures below their ITT but become insoluble and aggregate at temperatures above their ITT (Meyer & Chilkoti, Citation2002). The random disordered structure observed at aqueous solutions at low temperatures aggregates to ordered type II β turn structure as the temperature increases () (Li et al., Citation2001; Arkin & Bilsel, Citation2010). The ITT of ELPs can be precisely tuned between 0 °C and 100 °C with a precision of a few degrees Celsius and optimized for specific applications like drug delivery and protein purification. This phase transition behavior of ELPs is sensitive to numerous variables, including molecular weight, concentration, co-solute, presence of guest residue, hydrophobicity and mole fraction. For example, it has been observed that hydrophobic guest residues lower the ITT whereas hydrophilic residues increase the ITT. The distribution of the guest residues along the polypeptide chain and incorporation of residues with ionizable side chains at the fourth position of ELPs can also alter their ITT (Urry, Citation1997). The exact placement and nature of each amino acid is also important for its ITT behavior. Biocompatibility, biodegradability and non immunogenic properties of ELPs suggest their suitability for in vivo applications (Mackay & Chilkoti, Citation2008). ELPs have molecular weight greater than the renal threshold and can be retained for significantly long periods of time in blood circulation, thus increasing their plasma half-lives (Liu et al., Citation2006; McDaniel et al., Citation2010). They are degradable by proteolysis which reduces their adverse side effect due to long-term accumulation in the body (Mackay & Chilkoti, Citation2008). Shah et al. (Citation2012) have extensively studied the biodegradability of ELPs in different physiological microenvironments. They elaborated that the rate of biodegradation controls the accumulation of ELPs at the target site as well as clearance from the body and suggested a detailed mechanism for the degradation of ELPs. ELPs are internalized by cells pinocytosis, co-localized in low pH compartments, namely lysosomes and are eliminated over a period of 2–24 h after proteolytic cleavage by elastase and collagenase endopeptidases.
Figure 1. Structural transition behavior of the ELPs with respect to temperature change responsible for its ITT behavior.

Further, it has been shown that ELPs as drug carriers do not cause any inflammation at the site of injection. It is non toxic to mouse fibroblast, do not produce systemic toxicity, dermal irritation, systemic antigenicity and hemolysis (Urry et al., Citation1991; Cappello et al., Citation1998). ELPs can be used for facile attachment of chemotherapeutics at precise positions for preparing drug conjugates (Dreher et al., Citation2003).
Synthesis of ELPs
Elastin-like polypeptides can be synthesized in a precisely control manner with specific peptide sequence and molecular weight with low polydispersity using recursive directional ligation (RDL) recombinant DNA techniques (Meyer & Chilkoti, Citation2002). Kaufmann & Weberskirch (Citation2006) have described efficient synthetic methods for ELPs based on the solid-phase peptide synthesis using resin as solid support in the presence of HoBt/PyBob as activating agents. Chow et al. (Citation2008) have reviewed the various available methods for the synthesis of ELPs. They reported that ELPs can be synthesized via chemical methods, namely solid phase peptide synthesis as synthetic polypeptides and by recombinant DNA methodologies as genetically encoded polypeptides. They suggest that the recombinant DNA methodologies offer advantages like high specificity in sequence, stereochemistry and molecular weight with some limitations.
Anticancer drug targeting applications of ELPs
Cancer treatment is a multi-pronged strategy. Current cancer therapies include surgical resection of tumor, radiation and chemotherapy which varies depending on the nature of the tumor (Chabner & Longo, Citation2001). Among these three modalities, improvements to chemotherapy offer some of the most exciting opportunities to develop newer treatment approaches. The primary drawback of conventional chemotherapy using cytotoxic drugs is the by-stander toxicity on normal healthy cells. Various chemotherapeutic agents with anti-cancer properties such as Doxorubicin, Paclitaxel, Camptothecin and Melphalan are limited by their narrow therapeutic index due to unwanted toxicity to normal healthy cells. These cytotoxic drugs utilize the same processes in normal and cancerous cells for cell killing ability. In order to prevent unwanted effects to normal healthy dividing cells, it would be desirable to target these cytotoxic drugs to tumor cells in a site specific manner in addition maintaining the therapeutic concentration of drugs over long periods of time (Huang & Oliff, Citation2001; Atkins & Gershell, Citation2002; Moses et al., Citation2003; Duncan, Citation2006).
Several investigations have been carried out since 1960s on targeting applications. In particular, Lysosomotropic polymer-drug conjugates, block copolymer micelles and PEG-protein conjugates came into consideration due to the revolutionary work of De Duve et al. (Citation1974), Ringsdorf (Citation1975), Abuchowski et al. (Citation1977) and others. Further, since 1980s onwards Duncan and Kopecek have initiated research on the use of synthetic polymers like N-2-hydroxypropyl methacrylamide (HPMA), Poly ethyleneglycol (PEG) and Poly N-isopropylacrylamide (Poly-NIPAAm) for drug delivery purposes (Duncan & Kopecek, Citation1984; Duncan, Citation1992, Citation2009; Duncan et al., Citation1992). The first polymer-drug conjugate, HPMA-Doxorubicin conjugate, has now entered Phase II clinical trials. Several anticancer-drug conjugates have entered into clinical development, and a growing number of polymeric drugs and PEGylated proteins have since come into the market (Duncan & Vicent, Citation2013). These approaches have achieved varying degrees of success due to the complex barriers to drug transport within the tumor (Jain, Citation1989, Citation2001). Further, significant research efforts have been directed towards targeting anticancer drugs to tumors using specialized drug carriers. Biopolymers have become an important component of these targeting approaches (Dreher et al., Citation2003).
In this context, thermally responsive elastin-like polypeptides (ELPs) have also been examined. These polymers have several attractive features. In particular their ITT behavior has attracted the attention of researches. The tunable ITT of ELPs between 37 °C and 42 °C can be exploited for delivering drugs to solid tumors by the application of external hyperthermia. As temperature increases above ITT, ELPs undergo reversible phase transition and form insoluble aggregates and are internalized by cells via pinocytosis. Mild hyperthermia promotes high blood flow and high vascular permeability of therapeutic agents at tumor site (Fujiwara & Watanabe, Citation1990; Ponce et al., Citation2006). It has also been observed that hyperthermia can affect the biological function of cancer cells by inhibiting DNA synthesis and repair mechanism (Jain, Citation1987; Nakayama & Okano, Citation2011). Further, Matsumura & Maeda (Citation1986) have shown that macromolecular carriers like ELPs passively target tumors through the enhanced permeability and retention (EPR) effect. The EPR effect can be attributed to two factors, namely the disorganized pathology of angiogenic tumor vasculature with its discontinuous endothelium that provides hyperpermeability to circulating macromolecules. The lack of effective tumor lymphatic drainage leads to subsequent macromolecular accumulation. The leaky tumor vasculature developed by abnormal and poorly controlled angiogenesis results in vessel walls with pores ranging in size from 200 nm to 2 µm. This allows macromolecules to preferentially extravasate into the tumor through the EPR effect in contrast to healthy blood vessels, wherein the intact tight junctions between endothelial cells prevent transvascular migration of drug carriers and cargo into healthy tissues (Yuan et al., Citation1995; Maeda et al., Citation2009).
The ability to synthesize ELPs with a precise molecular weight with low polydispersity using genetically engineering techniques, favorable pharmacokinetics, the potential biocompatibility and their controlled degradation make them interesting delivery vehicles for systemic anticancer drug delivery (Duncan, Citation2006). Several investigations have been carried out in recent years towards this end. Meyer et al. (Citation2001a) have synthesized a thermally responsive ELP with an ITT of 41 °C and thermally unresponsive control ELP using genetic engineering techniques. In vivo fluorescence video microscopy and radiolabel distribution studies revealed that the hyperthermic targeting of the thermally responsive ELP shows a 2-fold increase in tumor localization compared to the unresponsive ELP in SKOV-3 ovarian carcinoma and D-54MG glioma implanted in nude mice. They observed aggregates of the thermally responsive ELP within the heated tumor and concluded that accumulation is caused due to its ITT behavior. Further, the results of window chamber studies also reveal the preferential accumulation of the thermally responsive ELPs in heated tumors due to the combined effect of EPR and hyperthermia.
Meyer et al. (Citation2001b) have also performed a study using a genetically engineered ELP and synthetic thermally responsive copolymer of N-isopropylacrylamide (NIPAAm) and showed a 2-fold increase in the accumulation of drug in ovarian tumors implanted in dorsal skin of nude mice with local hyperthermia. The results thus reveal that enhanced delivery of anticancer drugs to solid tumors can be achieved by conjugating drugs to thermally responsive natural as well as synthetic polymers with mild hyperthermia.
Raucher & Chilkoti (Citation2001) have examined the quantitative uptake of a fluorescence-labeled and thermally responsive ELP as a function of its concentration between 5 and 15 µM in solution in response to hyperthermia by three cultured cancer cell lines. Flow cytometry of the fluorescein-ELP conjugate showed that hyperthermia enhances the cellular uptake of the thermally responsive ELP in human ovarian carcinoma, SKOV-3 and HeLa cells at a concentration of 10 µM or higher as compared with the uptake of a thermally inactive ELP control. In FaDu cells, hyperthermia stimulated the uptake of the thermally responsive ELP at all solution concentrations of ELP between 5 and 15 µM. In particular, a >2-fold greater uptake of thermally responsive ELP compared to the thermally inactive control ELP was observed for FaDu cells at a solution concentration of 15 µM in heated cells. Further, confocal fluorescence microscopy of tumor cells incubated with a rhodamine conjugate of the thermally responsive ELP showed that the cytoplasm was uniformly stained below its ITT. Above its ITT, fluorescent particles were observed in the cytoplasm, suggesting that these particles are aggregates of the thermally responsive ELP.
Chilkoti et al. (Citation2002a) have reviewed the recombinant DNA methods for the design and synthesis of ELPs for targeted delivery of radionuclides, chemotherapeutics and biomolecular therapeutics to tumors and concluded that ELPs as drug carriers enhance the localization of ELP-drug conjugates within solid tumors when external hyperthermia is applied.
Chilkoti et al. (Citation2002b) have also reviewed the results on the development of macromolecular carriers for thermal targeting of therapeutics to solid tumors. The two thermally responsive polymers namely, poly(N-isopropylacrylamide-coacrylamide) [poly(NIPAAm)] and an artificial ELP designed by them exhibited ITT behavior at a temperature slightly >37 °C. In vivo fluorescent videomicroscopy and radiolabel distribution studies of ELP delivery to human tumors implanted in nude mice demonstrated that hyperthermic targeting of the thermally responsive ELP for 1 h provides a ∼2-fold increase in tumor localization compared to the same polypeptide without hyperthermia. Similar results were also obtained for poly(NIPAAm) though the extent of accumulation was somewhat lesser than observed for ELP.
In an effort to understand the behavior of ELPs within the tumor, Dreher et al. (Citation2003) have synthesized a thermally responsive ELP by genetic engineering technique and conjugated Doxorubicin through an acid labile hydrazone bond to enable its release in the acidic environment of the lysosomes. The results of confocal fluorescence microscopy revealed that the ELP-Doxorubicin conjugate was endocytosed by squamous cell carcinoma cells (FaDu) and trafficked into the lysosomes. Results also revealed that both the free drug and ELP-Doxorubicin conjugate exhibit near equivalent in vitro cytotoxicity, although their subcellular localization was significantly different. The free drug was largely concentrated in the nucleus whereas the ELP-Doxorubicin conjugate was dispersed throughout the cytoplasm with limited nuclear accumulation.
Bidwell & Raucher (Citation2005) have shown the application of a genetically engineered ELP as a delivery vehicle for a short peptide capable of inhibiting the transcriptional function of a specific oncogene. The sequence for the ELP was modified by the addition of the membrane translocating sequence, penetratin and a peptide derived from helix 1 of the helix-loop-helix region of c-Myc (H1S6A, F8A) known to inhibit c-Myc transcriptional function. The designed polypeptide, Pen-ELP-H1, was then expressed and purified from Escherichia coli. The results of the flow cytometry analysis revealed that the cellular uptake of Pen-ELP-H1 is enhanced by both the penetratin sequence and hyperthermia-induced phase transition. Further, the results of the immunofluorescence and reverse transcription-PCR studies revealed that the Pen-ELP-H1 is able to disrupt the nuclear localization of c-Myc and inhibit transcriptional activation by c-Myc. Cell proliferation studies showed that Pen-ELP-H1 inhibits growth of MCF-7 cells. Further, the use of hyperthermia increased the antiproliferative effect of the thermally responsive Pen-ELP-H1 by 2-fold when compared with a nonthermally responsive control polypeptide. The authors thus showed that genetically engineered ELP carriers can provide a new way to thermally target specific oncogene inhibitors to solid tumors.
Herrero-Vanrell et al. (2005) have studied the self-assembled Poly(VPAVG) micro-and nanoparticles as vehicles for the controlled release of Dexamethasone phosphate (DMP). Poly (VPAVG) was prepared as stable particles with a size <3 µm above its transition temperature (∼30 °C). These self-assembled particles were able to encapsulate significant amounts of the drug when self-assembling was carried out in a co-solution polymer-DMP. The results of their study revealed sustained DMP release and confirmed that ELPs have the potential to release drugs to specific sites.
Furgeson et al. (Citation2006) have synthesized a thermoresponsive and genetically engineered ELP containing a C-terminal cysteine residue and conjugated Doxorubicin to the ELP through different pH-sensitive and maleimide activated hydrazone linkers. The results revealed that the ELP-Doxorubicin conjugates with longer linkers exhibit slower drug release kinetics compared to the ELP-Doxorubicin conjugates with shorter linkers.
Kaufmann & Weberskirch (Citation2006) have investigated an elastin mimetic polypeptide-drug conjugate for targeted delivery of Doxorubicin and demonstrated that elastin mimetic polypeptide, GRDPSS(VPGVG VPGKG VPGVG VPGVG VPGEG VPGIG)7 can be used as potential drug carriers for cancer chemotherapy. They used an efficient synthetic method for conjugating to Doxorubicin to the polypeptide thus avoiding the generally used multi-step synthesis of drug conjugation with higher cost and lower efficiency.
Liu et al. (Citation2006) have synthesized a genetically engineered ELP and examined its preferential accumulation in solid tumors upon mild hyperthermia. They investigated the biodegradability, pharmacokinetics, tumor localization and tumor spatial distribution of a C14-labeled ELP. The tumor accumulation and spatial distribution of the intravenously administered C14-labeled ELP and a control ELP (that was designed to remain soluble in heated tumors) were examined in both heated (41.5 °C) and unheated tumors. The C14-labeled ELP was found to accumulate in significantly higher concentrations in heated tumors than in unheated tumors. Quantitative autoradiography of tumor sections provided similar tumor accumulation results as the whole tumor analysis. In addition, the C14-labeled ELP had a more homogeneous distribution in heated tumors and a greater concentration in the tumor center than the control treatment.
Bidwell et al. (Citation2007) have investigated the multidrug resistance problems in cancer cells, a major obstacle for successful anticancer therapy. The major mechanism of resistance involves cellular drug efflux by the expression of P-glycoprotein (P-gp), a membrane transporter, with a wide variety of substrates. In this context, they pointed out that anthracyclines, a class of antitumor drugs, are especially prone to induction of resistance by the P-gp mechanism. To overcome the problem associated with multidrug resistance, they synthesized novel analogs of drugs conjugated to ELPs in order to circumvent the multidrug resistance. The results obtained thus suggest that ELP-Doxorubicin conjugates may provide a means to thermally target Doxorubicin to solid tumors and overcome multidrug resistance in cancer cells.
Dreher et al. (Citation2007) have studied a new methodology to target a temperature responsive ELP to solid tumors. They used a dorsal skin fold window chamber model and intravital laser scanning confocal microscopy for their study and showed that ELP forms micron-sized aggregates that adhere to the tumor vasculature when hyperthermia is applied. Their results further revealed that thermal cycling of tumor increases the exposure of tumor cells to the ELP drug carrier. The study thus highlights a new approach, namely tumor thermal cycling, to exploit stimuli-responsive polymers in vivo to target to tumor vasculature with high specificity.
Tumor cell metastasis is a complex multi-step process which is a major cause of death and morbidity amongst cancer patients. Cell adhesion plays a critical role in the development of metastatic cancer. It is mediated by interactions between receptors on the cell surface and ligands of the extracellular matrix or other surfaces. Inhibition of the cell adhesion process appears to be an effective method of preventing metastasis. In this context, Massodi et al. (Citation2009a) have investigated antitumor application of ELPs by fusing a cell penetrating peptide to ELPs. The study results revealed that the cell penetrating peptide Tat, (YGRKKRRQRRR), fused with an ELP inhibits adhesion, spreading, invasion and migration of human SKOV-3 ovarian cancer cells in cell culture. In addition, they also confirmed that Tat-ELP has significant anti-metastatic potential in an experimental ovarian cancer metastasis model in vivo, causing ∼80% reduction in the tumor burden. Since cell attachment is an important step in tumor cell invasion and metastasis, these results suggest a novel role of Tat-ELP as a therapeutic intervention in cancer metastasis.
Massodi et al. (Citation2009b) have also investigated a thermally responsive ELP fused to a Lactoferrin-derived peptide for the treatment of pancreatic cancer. They showed that the well-characterized peptide derivative of bovine lactoferrin L12 possesses anticancer properties in multiple cell lines with some limitations. However, adverse side effects in normal tissues and poor plasma kinetics of this peptide hinders its clinical effectiveness. To overcome these limitations, they developed Tat-ELP-L12 drug conjugate for targeted delivery of the L12 peptide and found that the conjugate is soluble in aqueous solution at 37 °C but aggregates near 41 °C, which makes this conjugate ideal for targeting to solid tumors. The results of their study revealed that under hyperthermic conditions the designed Tat-ELP-L12 drug conjugate possesses 30-fold increased cytotoxic properties in MIA PaCa-2 pancreatic adenocarcinoma cells.
Mackay et al. (Citation2009) have developed a recombinant chimeric polypeptide (CP)-Doxorubicin conjugate nanoparticle formulation and evaluated the same for its in vivo efficacy in a murine tumor model. The results revealed that the ELP spontaneously self assemble into sub-100 nm size upon conjugation with Doxorubicin. They concluded that the ELP-Doxorubicin conjugate has a 4-fold higher accumulation in solid tumor when compared to the free drug and the nanoparticle-based formulation of CP can be used as a multifunctional nanomedicine.
Wu et al. (Citation2009) have described a novel application of electrospraying to construct ELP nanoparticles for drug delivery purposes. Electrospraying is an emerging method for the rapid and high throughput production of nanomeso scale particles of same morphology for controlled drug delivery applications. They studied the encapsulation and triggered release of a model drug, Doxorubicin, from ELP nanoparticles. The study results demonstrate that electrospraying is an efficient and flexible method for generating stimuli-responsive drug particles that have potential drug delivery applications.
Bidwell & Raucher (Citation2010) have developed ELP fused therapeutic peptides for thermally targeted delivery of cell penetrating peptides (CPPs) to enhance their intracellular delivery in various cancers. The study results revealed that the ELPs are a promising carrier for delivery of therapeutic peptides, namely cell penetrating peptides.
Liu et al. (Citation2010) have synthesized and evaluated ELPs of molecular weight ∼49 kDa using genetic engineering techniques. An ELP1 radio-conjugate was designed to spontaneously undergo a soluble-insoluble phase transition between room temperature and body temperature upon intratumoral injection and on ELP2 radio-conjugate was designed to remain soluble upon injection as a control. The study results revealed that after intratumoral administration, ELP1 radio-conjugate retained in the tumor for significantly longer periods when compared to the ELP2 radio-conjugate. The study also revealed that ELP1 radio-conjugate has low systemic toxicity even at high radionuclide doses, delay tumor growth and improve survival when compared to ELP2 radio-conjugate.
MacEwan et al. (Citation2010) have shown that ELPs as carriers enhance the efficacy of antitumor therapeutics when compared to the administration of free drugs by three mechanisms, namely increasing the overall accumulation within solid tumors, providing a homogeneous spatial distribution in tumor tissues and increasing the intracellular localization of anticancer therapeutics. The study results highlight recent developments in “smart” stimulus responsive peptides and lipid drug carriers designed to enhance the localization and efficacy of therapeutic payloads as compared to free drugs.
Massodi et al. (Citation2010) have studied the inhibition of ovarian cancer cell proliferation by a cell cycle inhibitory peptide fused to a thermally responsive ELP. The coding sequence of the ELP was modified by the addition of the cell penetrating peptide, Bac-7, at the N-terminus and a 23 amino acid peptide derived from p21 at the C-terminus (Bac-ELP1-p21). Bac-ELP1-p21 is soluble in aqueous solutions below physiological temperature (37 °C) but aggregates when the temperature is raised >39 °C, making it a promising thermally responsive therapeutic carrier that may be actively targeted to solid tumors by the application of hyperthermia. The study results revealed that Bac-ELP1-p21 at 37 °C do not have any effect on SKOV-3 cell proliferation and the use of hyperthermia increases the antiproliferative effect of Bac-ELP1-p21 compared to the thermally unresponsive control polypeptide. Confocal microscopy study revealed that Bac-ELP1-p21 displays both a cytoplasmic and nuclear distribution in the SKOV-3 cells, with nuclear-localized polypeptide enriched in the heated cells. Further, western blotting study results revealed that Bac-ELP1-p21 causes a decrease in Rb phosphorylation levels in cells treated at 42 °C. The polypeptide also induced caspase activation, PARP (Poly ADP ribose polymerase) cleavage and cell cycle arrest in S-phase and G2/M-phase. These studies indicate that ELP is a promising macromolecular carrier for the delivery of cell cycle inhibitory peptides to solid tumors.
Chen et al. (Citation2011) have presented a novel thermosensitive ELP-based diblock copolymer – geldanamycin conjugate for thermo-targeted drug delivery of geldanamycin. In their studies, they chose geldanamycin as a chemotherapeutic agent because it has low IC50 ∼1 nM and inhibition activities for pro-oncogenic pathways. The study results demonstrated that the ELP-based diblock copolymer-geldanamycin conjugate forms stable nanoconstructs and displays tunable, acute phase transitions behavior at high temperatures. The study results also demonstrated that the ELP-based diblock copolymer-geldanamycin conjugate has great potential for thermo targeted chemotherapy of the tumor margins and inhibition of pro-oncogenic pathways.
Simnick et al. (Citation2011) have studied the novel nanoscale in vivo antitumor application of Asn-Gly-Arg (NGR) tripeptide coupled to the recombinant diblock copolypeptide of ELP. Their study results revealed that NGR tripeptide specifically targets the neovasculature via interaction with the aminopeptidase N (APN/CD13) receptor which is highly up-regulated in the membranes of endothelial tumor cells.
Walker et al. (Citation2012) have demonstrated that ELPs passively accumulate in solid tumors and aggregate in tumor tissues when external hyperthermia is applied. They conjugated Doxorubicin, and three different cell penetrating peptides (CPP) to an ELP. Fluorescence microscopy studies in MCF-7 breast carcinoma cells demonstrated that the three different CPP-ELP-Doxorubicin conjugates deliver Doxorubicin to the cell nucleus. Under hyperthermic conditions, tumor inhibition with CPP-ELP-Doxorubicin was found to be 2-fold higher than under therapy with free Doxorubicin at the equivalent dose.
Moktan et al. (Citation2012) have investigated the thermal targeting of an acid-sensitive ELP-Doxorubicin conjugate and evaluated its therapeutic efficacy in vivo against the free drug. The results revealed that the ELP aggregates in response to mild hyperthermia and accumulate in solid tumors but remain soluble under normal physiological conditions. They evaluated the therapeutic potential of ELPs in delivering Doxorubicin in the E0771 syngeneic mouse breast cancer model.
McDaniel et al. (Citation2012) have reported on the design, physicochemical characterization and in vitro cytotoxicity studies of a thermally responsive chimeric polypeptide (CP) derived from an ELP. They found that CP self-assembles into ∼40 nm diameter nanoparticles upon conjugation with an anticancer drug, Doxorubicin and displays a phase transition behavior between 39 °C and 42 °C. They showed CP-Doxorubicin nanoparticle is stable upon dilution to low micromolar concentrations and exhibit cytotoxic activity at 37 °C and 42 °C. The study results revealed that thermally responsive nanoparticle developed may be broadly useful in hyperthermia mediated targeted chemotherapy for a variety of solid tumors.
Na et al. (Citation2012) have synthesized the ELP-liposome-drug conjugate and studied its application for enhanced cellular uptake of drug in tumor cells. They investigated temperature-triggered drug release properties of the conjugate over a range of temperatures and evaluated its cellular uptake efficiency under normothermic and hyperthermic conditions. Flow cytometry and confocal microscopy studies were also carried out to investigate drug accumulation inside tumor cells. The study results revealed that higher ELP-liposome-drug conjugate internalization occurs in the ELP-modified liposomes when compared to ELP-unmodified liposomes. The study results also suggest that dehydration of ELP molecules on the liposomal surface can induce efficient cellular uptake and improve chemotherapeutic efficacy.
MacEwan & Chilkoti (Citation2012) have described a newer digital technique for specific cellular uptake application of a diblock copolymer of ELP and its genetically encoded self-assembled temperature triggered CPP-arginine analog. They developed a nanoparticle micelle by modulation of diblock copolymer ELPs with the CPP-arginine. The study results revealed that temperature triggered arginine analog of ELP has increases cellular uptake when compared to the same diblock copolymer of ELPs without arginine.
Callahan et al. (Citation2012) have reported the first pH-responsive polypeptide micelle that dissociates at the low extracellular pH of the solid tumors. They developed a histidine-rich ELP block copolymer, which self-assembles at 37 °C into spherical micelles for better in vivo penetration and distribution of ELPs in tumors.
Rapamycin, an extremely potent in cancer treatment, has a number of drawbacks such as severe cytotoxicity, low bioavailability and rapid clearance limit its wider use. Shi et al. (Citation2013) have investigated the application of a biosynthesized ELP as a nanocarrier. They developed an encapsulation strategy in which Rapamycin associates tightly to an ELP. The study results revealed that the ELP carrier delivers more Rapamycin to the tumor sites via the EPR effect.
Nitta & Numata (Citation2013) have reviewed ELP-based nanoparticles for drug/gene delivery and tissue engineering applications. They observed a well-defined unique phase transition behavior of ELPs that promotes recombinant expression, protein purification and self-assembly of nanostructures. They concluded that the multi-functional biopolymer-based and biopolymer-synthetic polymer conjugated nanoparticles, precisely targets drug/gene to specific site in cancer chemotherapy.
The treatment of glioblastoma is complicated due to high resistance to chemotherapy, poor penetration of drugs across the blood brain barrier and damaging effects of chemotherapy to normal neural tissue. To overcome these limitations, Bidwell et al. (Citation2013) have used an ELP as a peptide carrier that forms aggregates above a characteristic transition temperature. They modified the ELP with cell-penetrating peptides (CPPs) to enhance delivery to brain tumors and mediate uptake across the tumor cells using hyperthermia in in vivo models. The results indicate that ELP-mediated drug targeting enhances 5-fold drug accumulation at tumor site when external hyperthermia is applied.
Patel et al. (Citation2013) have studied drug delivery and tissue engineering applications of ELP-collagen composite microstructure. They prepared an ELP-collagen-Doxycycline composite formulation for in vitro drug release profile study. The study results demonstrate that fabrication is an efficient method for generating stimuli-responsive microstructure drug particles. Their study results open possibilities to understand the effect of temperature on the morphology and drug release characteristics of ELP-collagen-Doxycycline composites that have potential for drug delivery applications.
A recent study on the use of a short synthetic ELP
Several investigations have thus been carried out using ELPs for drug delivery purposes. ELP-based drug conjugates, no doubt, have several possible advantages over the parent free drug like improved drug pharmacokinetics, passive tumor targeting by the EPR effect, decreased toxicity, increased solubility in biological fluids, site-specific drug targeting and prolonged biological half-life, thus leading to less frequent administration to the patient and a programmed profile of drug release (Raucher & Chilkoti, Citation2001).
In all the investigations discussed above, genetic engineering techniques have been generally used for synthesizing ELPs (). This synthetic technique is, however, a cumbersome and expensive process (Chow et al., Citation2008). The presence of reactive side chain in these ELPs also makes them unstable over long periods of time and at higher temperatures (Nicolini et al., Citation2004). Conjugating drugs to these ELPs are also cumbersome. There is a need, therefore, to develop more versatile chemical approaches that require fewer synthetic steps. In this context, it is our hypothesis that short synthetic ELPs can be used as drug carriers. They also offer the possibility of higher amount of drug conjugation. A detailed literature survey revealed that a synthetic short ELP, GVG(VPGVG)n where n = 1, has been extensively studied for its ITT, structural and physical transition behavior (Castiglione-Morelli et al., Citation1990; Reiersen et al., Citation1998; Rousseau et al., Citation2004). The results of this study reveal that this short ELP also behaves similar to long ELPs regarding its capability to undergo ITT behavior. In other words, the very important polymer behavior of ELPs, namely formation of type II β turn structure can be reproduced by a single VPGVG unit and a pentameric repeat unit is not always necessary (Reiersen et al., Citation1998). The literature survey, however, reveal that such a short synthetic ELP has not so far been investigated for targeting anticancer drugs to solid tumors.
The authors of this review, therefore, synthesized, characterized and evaluated such a short ELP-drug conjugate (). The ELP, namely GVG(VPGVG)n where n = 1, was coupled to Doxorubicin at its C-13 position through a hydrolysable hydrazone linkage to obtain the ELP-Doxorubicin conjugate (). The ESI-MS, FTIR, NMR spectral studies carried out on the ELP-Doxorubicin conjugate indicate that the drug conjugate synthesized is in agreement with the predicted structure. The results of the temperature induced turbidity and Differential Scanning Calorimetry (DSC) studies show that the short ELP exhibits ITT behavior similar to genetically engineered large ELPs. The results reveal that the ELP is soluble in aqueous solution at 37 °C but as the temperature increases it starts aggregating >40 °C, namely, ITT (). This temperature, namely ∼40 °C, is in between the body temperature, 37 °C and 42 °C, a temperature approved for clinical hyperthermia thus confirming that the short ELP can also be used for thermal targeting to solid tumors. Further, DSC results also indicate a clear visualization of the physical transition of the ELP. An exothermic peak near 40 °C observed denotes the peak temperature corresponding to a phase transition. The thermogram also shows a glass transition for the ELP at 97 °C, corresponding to the degeneration of ELP at higher temperatures ().
Figure 4. ELP-Doxorubicin conjugate. The ELP was coupled to Doxorubicin at its C-13 position through a hydrolysable hydrazone linkage.
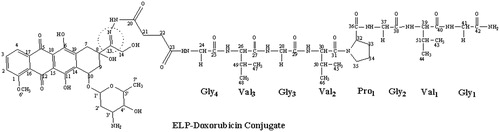
The results obtained by us from cytotoxicity assay show a similar percentage growth inhibition (cell killing ability) for both the free drug, Doxorubicin and the synthesized short ELP-Doxorubicin conjugate though the drug and the conjugate show different intracellular distribution pattern as found from confocal fluorescence microscopy study (). Cell viability results also confirm that the short ELP does not show any cytotoxicity and also does not alter the functional activity of the free drug, Doxorubicin, on BMG-1 cerebral glioma cell lines.
Figure 7. Cytotoxicity effect of ELP, Doxorubicin and ELP-Doxorubicin conjugate on BMG-1 cell lines.
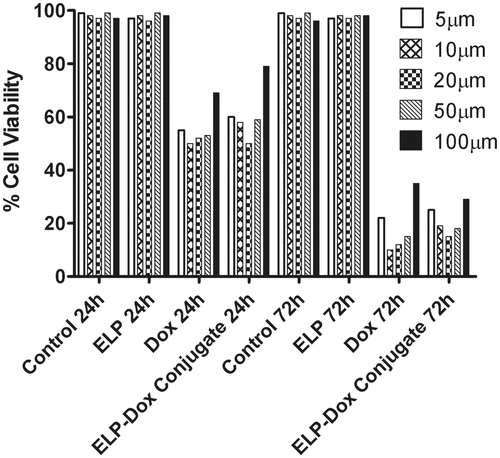
Intracellular distribution of the free drug, Doxorubicin and the synthesized short ELP-Doxorubicin conjugate were examined by confocal fluorescence microscopy. The data reveal that fluorescence and hence cellular uptake is weak after 4 h of incubation. However, fluorescence emerges at 24 h and gradually increases with longer incubation. These results are consistent with in vitro data which reveal that at 24 h the free drug and synthesized short ELP-Doxorubicin conjugate show ∼42% growth inhibition and at higher incubation time, namely, 72 h they show ∼78% growth inhibition. The microscopic images reveal that the free drug targets the nuclei whereas the short ELP-Doxorubicin conjugate targets mostly the perinuclear region of the cytoplasm. The free drug and the short ELP-Doxorubicin conjugate thus show different intracellular distribution and hence exhibit different mechanism of cellular uptake (). The fluorescence intensity results also confirm that the drug uptake from the ELP-Doxorubicin conjugate is higher when compared to the free drug (). This is due to the fact that the conjugate involves a different mechanism of drug uptake and release. The ELP-Doxorubicin conjugate is taken in by the cells by endocytosis and trafficked via endosomal compartments to the lysosomal compartments. The lysosomal enzymes at acidic pH hydrolyze the acid sensitive hydrazone bond of the ELP-Doxorubicin conjugate and release the free drug. The free drug then diffuses from lysosome into the cytoplasm and causes cell lysis.
Figure 8. Confocal fluorescence images showing intracellular localization of free drug, Doxorubicin and ELP-Doxorubicin conjugate.
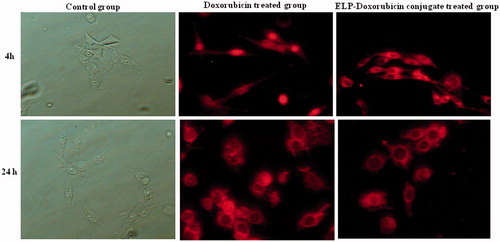
Table 1. Cellular uptake measured by flow cytometry.
Our investigations, however, reveal that the free drug, Doxorubicin and the short ELP-Doxorubicin conjugate show different cellular distribution but surprisingly have similar cytotoxicity. Further, in vivo studies are necessary to unequivocally prove that the drug molecules accumulate at solid tumor site when external hyperthermia is applied. Studies are in progress to investigate these aspects.
Conclusions
Effective cancer chemotherapy can be achieved if appropriate delivery systems are available and novel approaches are used in appropriate combinations for targeting purposes. This review highlights the various aspects of ELPs as polymeric carriers for drug delivery applications especially cancer chemotherapy by exploiting their unique property, namely ITT, in addition to targeting tumors passively through their EPR effect. ELPs can be used for not only for conjugating anticancer agents but also other therapeutic peptides, like cell penetrating peptides, lactoferrin peptides, radionuclide conjugate, antiproliferating peptides, penetratin for targeted delivery and multidrug resistance nanomedicine applications. This review also brings out that a short synthetic and thermally responsive octamer, GVG(VPGVG)n, where n = 1, can be used as a carrier for targeted delivery. In conclusion, the present review focuses on ELPs for drug delivery applications to improve the therapeutic efficacy of pharmacological agents particularly anticancer drugs. The review also opens possibilities for the synthesis of newer ELPs.
Future perspectives
The present review brings out the application of ELPs as carriers for targeting chemotherapeutic agents, particularly, anticancer agents to the intracellular space. In future, it is possible that an increased understanding of drug delivery aspects and tailoring of ELPs to optimize their thermal control for pharmacokinetic clearance and also how the concentration of ELPs affect their ITT behavior may contribute newer dimensions to tumor targeting. Nanoparticle-based ELP-drug conjugates can also be investigated to enhance drug accumulation at tumor sites. These nanoparticle-based drug delivery systems may open up new frontiers for targeting to cancer by increasing tumor accumulation of anticancer drugs with reduced systemic toxicity. ELPs can also be possibly exploited for targeting in the case of metastasis. Current and future developments in polymeric-based drug delivery applications will no doubt open new frontiers in the next generation cancer chemotherapy.
Acknowledgements
One of the authors (RS) acknowledges Prof. S. Chandrasekaran, Prof. Erode N. Prabhakaran, Prof. A. K. Mishra, Prof. B. Dwarakanath and Dr. Raunak Varshney for helpful discussions.
Declaration of interest
Rubha Saxena acknowledges the Department of Science and Technology, Government of India for financial support under the Women scientist Scheme. The authors report no conflicts of interest. The authors alone are responsible for the content and writing of this article.
References
- Abuchowski A, McCoy JR, Palczuk NC, et al. (1977). Effect of covalent attachment of polyethylene glycol on immunogenicity and circulating life of bovine liver catalase. J Biol Chem 252:3582–6
- Arkin H, Bilsel M. (2010). How conformational transition depends on hydrophobicity of elastin-like polypeptides. Eur Phys J E Soft Matter 31:327–32
- Atkins JH, Gershell LJ. (2002). Selective anticancer drugs. Nat Rev Drug Discov 1:491–2
- Bidwell GL III, Davis AN, Fokt I, et al. (2007). A thermally targeted elastin like polypeptide doxorubicin conjugate overcomes drug resistance. Invest New Drugs 25:313–26
- Bidwell III GL, Perkins E, Hughes J, et al. (2013). Thermally targeted delivery of a c-Myc inhibitory polypeptide inhibits tumor progression and extends survival in a rat glioma model. PLoS One 8:e55104
- Bidwell GL III, Raucher D. (2005). Application of thermally responsive polypeptide directed against c-Myc transcriptional function for cancer therapy. Mol Cancer Ther 4:1076–85
- Bidwell GL III, Raucher D. (2010). Cell penetrating elastin-like polypeptides for therapeutic peptide delivery. Adv Drug Deliv Rev 62:1486–96
- Callahan DJ, Liu W, Li X, et al. (2012). Triple stimulus responsive polypeptide nanoparticles that enhance intratumoral spatial distribution. Nano Lett 12:2165–70
- Cappello J, Crissman JW, Crissman M, et al. (1998). In-situ self assembling protein polymer gel systems for administration, delivery and release of drugs. J Control Release 53:105–17
- Castiglione-Morelli A, Scopa A, Tamburro AM, Guantieri V. (1990). Spectroscopic studies on elastin like synthetic polypeptides. Int J Biol Macromol 12:363–8
- Chabner BA, Longo DL. (2001). Cancer chemotherapy and biotherapy: principles and practice. 3rd ed. Philadelphia, New York: Lippincott Williams & Wilkins
- Chen Y, Youn P, Furgeson DY. (2011). Thermo-targeted drug delivery of geldanamycin to hyperthermic tumor margins with diblock elastin-based biopolymers. J Control Release 155:175–83
- Chilkoti A, Dreher MR, Meyer DE. (2002a). Design of thermally responsive, recombinant polypeptide carriers for targeted drug delivery. Adv Drug Deliv Rev 54:1093–111
- Chilkoti A, Dreher MR, Meyer DE, Raucher D. (2002b). Targeted drug delivery by thermally responsive polymers. Adv Drug Deliv Rev 54:613–30
- Chow D, Nunalee ML, Lim DW, et al. (2008). Peptide-based Biopolymers in Biomedicine and Biotechnology. Mater Sci Eng R Rep 62:125–55
- Chung MI, Miao M, Stahl RJ, et al. (2006). Sequences and domain structure of mammalian, avian, amphibian and teleost tropoelastins: clues to the evolutionary history of elastins. Matrix Biol 25:492–504
- Daamen WF, Veerkamp JH, Van Hest JC, Van Kuppevelt TH. (2007). Elastin as a biomaterial for tissue engineering. Biomaterials 28:4378–98
- De Duve C, De Barsy T, Poole B, et al. (1974). Lysosomotropic agents. Biochem Pharmacol 23:2495–531
- Dreher MR, Raucher D, Balu N, et al. (2003). Evaluation of an elastin like polypeptide-doxorubicin conjugate for cancer therapy. J Control Release 91:31–43
- Dreher MR, Liu W, Michelich CR, et al. (2007). Thermal cycling enhances the accumulation of a temperature sensitive biopolymer in solid tumor. Cancer Res 67:4418–24
- Duncan R. (1992). Drug-polymer conjugate: potential for improved chemotherapy. Anticancer Drugs 3:175–210
- Duncan R. (2006). Polymer conjugates as anticancer nanomedicines. Nat Rev Cancer 6:688–701
- Duncan R. (2009). Development of HPMA copolymer anticancer conjugates: clinical experience and lessons learnt. Adv Drug Deliv Rev 61:1131–48
- Duncan R, Kopecek J. (1984). Soluble synthetic polymers as potential drug carriers. Adv Polym Sci 57:51–101
- Duncan R, Seymour LW, O’Hare KB, et al. (1992). Preclinical evaluation of polymer bound doxorubicin. J Control Release 19:331–46
- Duncan R, Vicent MJ. (2013). Polymer therapeutics-prospects for 21st century: the end of the beginning. Adv Drug Deliv Rev 65:60–70
- Fujiwara K, Watanabe T. (1990). Effects of hyperthermia, radiotherapy and thermoradiotherapy on tumor microvascular permeability. Acta Pathol Jpn 40:79–84
- Furgeson DY, Dreher MR, Chilkoti A. (2006). Structural optimization of a small Doxorubicin polypeptide conjugate for thermally targeted delivery to solid tumors. J Control Release 110:362–9
- He D, Chung M, Chan E, et al. (2007). Comparative genomics of elastins: sequence analysis of a highly repetitive protein. Matrix Biol 26:524–40
- Herrero-Vanrell R, Rincon AC, Alonso M, et al. (2005). Self-assembled particles of an elastin-like polymer as vehicles for controlled drug release. J Control Release 102:113–22
- Huang PS, Oliff A. (2001). Drug-targeting strategies in cancer therapy. Curr Opin Genet Dev 11:104–10
- Jain RK. (1987). Transport of molecules across tumor vasculature. Cancer Metastasis Rev 6:559–93
- Jain RK. (1989). Delivery of novel therapeutic agents in tumors: physiological barriers and strategies. J Natl Cancer Inst 81:570–6
- Jain RK. (2001). Delivery of molecular and cellular medicine to solid tumors. Adv Drug Deliv Rev 46:149–68
- Jensen SA, Vrhovski B, Weiss AS. (2000). Domain 26 of tropoelastin plays a dominant role in association by coacervation. J Biol Chem 275:28449–54
- Kaufmann D, Weberskirch R. (2006). Efficient synthesis of protein drug conjugates using a functionalizable recombinant elastin mimetic polypeptides. Macromol Biosci 6:952–8
- Li B, Alonoso DO, Daggett V. (2001). The molecular basis for the inverse temperature transition of elastin. J Mol Biol 305:581–92
- Liu W, Dreher MR, Furgeson DY, et al. (2006). Tumor accumulation, degradation and pharmacokinetics of elastin like polypeptides in nude mice. J Control Release 116:170–8
- Liu W, Mackay JA, Dreher MR, et al. (2010). Injectable intratumoral depot of thermally responsive polypeptideradionuclide conjugates delays tumor progression in a mouse model. J Control Release 144:2–9
- MacEwan SR, Callahan DJ, Chilkoti A. (2010). Stimulus responsive macromolecule and nanoparticles for cancer drug delivery. Nanomedicine (Lond) 5:793–806
- MacEwan SR, Chilkoti A. (2012). Digital switching of local arginine density in a genetically encoded self-assembled polypeptide nanoparticle controls cellular uptake. Nano Lett 12:3322–8
- Mackay JA, Chen M, McDaniel JR, et al. (2009). Self-assembling chimeric polypeptide-doxorubicin conjugate nanoparticles that abolish tumors after a single injection. Nat Mater 8:993–9
- Mackay JA, Chilkoti A. (2008). Temperature sensitive peptides: engineering hyperthermia-directed therapeutics. Int J Hyperthermia 24:483–95
- Maeda H, Bharate GY, Daruwalla J. (2009). Polymeric drugs for efficient tumor-targeted drug delivery based on EPR-effect. Eur J Pharm Biopharm 71:409–19
- Massodi I, Bidwell GL III, Davis A, et al. (2009a). Inhibition of ovarian cancer cell metastasis by a fusion polypeptide Tat-ELP. Clin Exp Metastasis 26:251–60
- Massodi I, Thomas E, Raucher D. (2009b). Application of thermally responsive elastin-like polypeptide fused to a lactoferrin-derived peptide for treatment of pancreatic cancer. Molecules 14:1999–2015
- Massodi I, Moktan S, Rawat A, et al. (2010). Inhibition of ovarian cancer cell proliferation by a cell cycle inhibitory peptide fused to a thermally responsive polypeptide carrier. Int J Cancer 126:533–44
- Matsumura Y, Maeda A. (1986). A new concept for macromolecular therapeutics in cancer chemotherapy: mechanism of tumoritropic accumulation of proteins and the antitumor agent smancs. Cancer Res 46:6387–92
- McDaniel JR, Callahan DJ, Chilkoti A. (2010). Drug delivery to solid tumors by elastin-like polypeptides. Adv Drug Deliv Rev 62:1456–67
- McDaniel JR, MacEwan SR, Dewhirst M, Chilkoti A. (2012). Doxorubicin conjugated chimeric polypeptide nanoparticles that respond to mild hyperthermia. J Control Release 159:362–7
- Meyer DE, Chilkoti A. (2002). Genetically encoded synthesis of protein-based polymers with precisely specified molecular weight and sequence by recursive directional ligation: examples from the elastin-like polypeptide system. Biomacromolecules 3:357–67
- Meyer DE, Kong GA, Dewhirst MW, et al. (2001a). Targeting a genetically engineered elastin-like polypeptide to solid tumors by local hyperthermia. Cancer Res 61:1548–54
- Meyer DE, Shin BC, Kong GA, et al. (2001b). Drug targeting using thermally responsive polymers and local hyperthermia. J Control Release 74:213–24
- Moktan S, Perkins E, Kratz F, Raucher D. (2012). Thermal targeting of an acid-sensitive doxorubicin conjugate of elastin-like polypeptide enhances the therapeutic efficacy compared with the parent compound in vivo. Mol Cancer Ther 11:1547–56
- Moses MA, Brem H, Langer R. (2003). Advancing the field of drug delivery: taking aim at cancer. Cancer Cell 4:337–41
- Muiznieks LD, Weiss AS, Keeley FW. (2010). Structural disorder and dynamics of elastin. Biochem Cell Biol 88:239–50
- Na K, Lee SA, Jung SH, et al. (2012). Elastin-like polypeptide modified liposomes for enhancing cellular uptake into tumor cells. Colloids Surf B Biointerfaces 91:130–6
- Nakayama M, Okano T. (2011). Multi-targeting cancer chemotherapy using temperature-responsive drug carrier system. React Funct Polym 71:235–44
- Nicolini C, Ravindra R, Ludolph B, Winter R. (2004). Characterization of the temperature- and pressure-induced inverse and reentrant transition of the minimum elastin-like polypeptide GVG(VPGVG) by DSC, PPC, CD, and FT-IR spectroscopy. Biophys J 86:1385–92
- Nitta SK, Numata K. (2013). Biopolymer-based nanoparticles for drug/gene delivery and tissue engineering. Int J Mol Sci 14:1629–54
- Patel NU, Purser CA, Baker RC, Janorkar AV. (2013). Effect of processing temperature on the morphology and drug-release characteristics of elastin-like polypeptide-collagen composite coatings. Biomacromolecules 14:2891–9
- Ponce AM, Vujaskovic Z, Yuan F, et al. (2006). Hyperthermia mediated liposomal drug delivery. Int J Hyperthermia 22:205–13
- Rapaka RS, Urry DW. (1978). Coacervation of sequential polypeptide models of tropoelastin. synthesis of H-(Val-Ala-Pro-Gly)n-Val-OMe and H-(Val-Pro-Gly-Gly)n-Val-OMe. Int J Pept Protein Res 11: 97–108
- Raucher D, Chilkoti A. (2001). Enhanced uptake of a thermally responsive polypeptide by tumor cells in response to its hyperthermia-mediated phase transition. Cancer Res 61:7163–70
- Reiersen H, Clarke AR, Rees AR. (1998). Short elastin like peptides exhibit the same temperature induced structural transitions as elastin polymers: implication of protein engineering. J Mol Biol 283:255–64
- Ringsdorf H. (1975). Structure and properties of pharmacologically active polymers. J Polym Sci Polym Symp 51:135–53
- Rousseau RE, Schreiner E, Kohlmeyer A, Marx D. (2004). Temperature dependent conformational transitions and hydrogen bond dynamics of the elastin like octapeptide GVG(VPGVG): a molecular dynamics study. Biophys J 86:1393–407
- Shah M, Hsueh PY, Sun G, et al. (2012). Biodegradation of elastin-like polypeptide nanoparticles. Protein Sci 21:743–50
- Shi P, Aluri S, Lin YA, et al. (2013). Elastin-based protein polymer nanoparticles carrying drug at both corona and core suppress tumor growth in vivo. J Control Release 171:330--8
- Simnick AJ, Amiram M, Liu W, et al. (2011). In vivo tumor targeting by a NGR-decorated micelle of a recombinant diblock copolypeptide. J Control Release 155:144–51
- Toonkool P, Jensen SA, Maxwell AL, Weiss AS. (2001). Hydrophobic domains of human Tropoelastin interact in a context-dependent manner. J Biol Chem 276:44575–80
- Urry DW. (1992). Free energy transduction in polypeptides and proteins based on inverse temperature transition. Prog Biophys Mol Biol 57:23–57
- Urry DW. (1997). Physical chemistry of biological free energy transduction as demonstrated by elastic protein-based polymers. J Phys Chem B 101:11007–28
- Urry DW, Parker TM, Reid MC, Gowda DC. (1991). Biocompatibility of the bioelastic material, poly(GVGVP) and its gamma irradiation cross-linked matrix-summary of generic biological test-results. J Bioact Compat Polym 6:263–82
- Vrhovski B, Weiss AS. (1998). Biochemistry of Tropoelastin. Eur J Biochem 258:1–18
- Walker L, Perkins E, Kratz F, Raucher D. (2012). Cell penetrating peptides fused to a thermally targeted biopolymer drug carrier improve the delivery and antitumor efficacy of an acid-sensitive doxorubicin derivative. Int J Pharm 436:825–32
- Wu Y, MacKay JA, McDaniel JR, et al. (2009). Fabrication of elastin-like polypeptide nanoparticles for drug delivery by electrospraying. Biomacromolecules 10:19–24
- Yuan F, Dellian M, Fukumura D, et al. (1995). Vascular permeability in a human tumor xenograft: molecular size dependence and cutoff size. Cancer Res 55:3752–6