Abstract
Cucurbitacin B (Cu B), a potent anti-cancer agent, suffers with the problems of water-insoluble, gastrointestinal side effects and non-specific toxicity via oral administration and drawbacks in patient’s compliance and acceptance through injections. An integration of nanoscale carriers with mucoadhesive buccal films drug delivery system would resolve these issues effectively with greater therapeutic benefits and clinical significance. Thus, the drug loaded mucoadhesive buccal film was developed and characterized in this study and the carboxymethyl chitosan (CCS) was chosen as a bioadhesive polymer, glycerol was chosen as a plasticizer and phospholipid-bile salts-mixed micelles (PL-BS-MMs) was selected as the nanoscale carriers. The CCS-films containing Cu B loaded PL-SDC-MMs was evaluated for the mechanical properties, mucoadhesion properties, in vitro water-uptake, in vitro release and morphological properties, respectively. The optimal CCS-films containing Cu B loaded PL-SDC-MMs was easily reconstituted in a transparent and clear solution with spherical micelles in the submicron range. The in vivo study revealed a greater and more extended release of Cu B from nanoscale CCS-films compared to that from a conventional CCS films (C-CCS-films) and oral marketed tablet (Hulusupian). The absorption of Cu B from CCS-films containing Cu B loaded PL-SDC-MMs resulted in 2.69-fold increased in bioavailability as compared to conventional tablet formulation and 10.46 times with reference to the C-CCS-films formulation. Thus, this kind of mucoadhesive buccal film might be an alternative safe route for delivery of Cu B with better patient compliance and higher bioavailability for the treatments.
Introduction
Cucurbitacin B (Cu B) is one of the main efficient components extracted from muskmelon pedicel and it could be also found in many other plants (Hu et al., Citation2013). Recently, Cu B has been considered as a potent anti-cancer agent with selective inhibitory effect on signal transducer and activator of transcription 3 (STAT3) pathway (Oh et al., Citation2002; Ukiya et al., Citation2002; Tannin-Spitz et al., Citation2007). Although the tablet and capsule of Cu B are available in Chinese market, problems of water-insoluble, gastrointestinal side effects and non-specific toxicity have restricted its clinical benefit (Bartalis & Halaweish, Citation2005; Yu et al., Citation2013). Applications of nanoscale carriers such as polymeric micelles and solid lipid nanoparticles have been studied to overcome the limitation (Molavi et al., Citation2008; Wang et al., Citation2010). But these approaches for the delivery of Cu B are all through injections. This method of delivery is largely associated with drawback in patient’s compliance and acceptance. Therefore, alternative safe routes for delivery of Cu B should be provided.
Compared to other dosage forms, the mucoadhesive buccal films may be preferred in terms of their thickness and small size (Lee et al., Citation1995). The interest in the mucoadhesive buccal films is driven not only by the promise of improved patient compliance, but also by the avoidance of first pass effect though exploiting absorption via the venous system that drains from the cheek area (Giovino et al., Citation2013). Thus, the integration of nanoscale carriers with mucoadhesive buccal films become an attractive safe route of administration for Cu B. However, this integration will be a significant challenge because aggregation during the film-forming process usually results in the inability to retain the desired nanometer particle size. Appropriate nanoscale carriers and film-formers would be highly desirable.
In this study, we want to select phospholipid (PL)-bile salts (BS)-mixed micelles (MMs) as the nanoscale carrier due to its locally and systemically well tolerated, with no embryo-toxic, teratogenic or mutagenic effects after administration (Alkan-Onyuksel et al., Citation1994; Hammad & Müller, Citation1998). The advantages of this proposed hypothesis are as follows:
The average particle size of the drug encapsulated in the PL-BS-MMs can reach to nano-level, which could allow relatively higher uptake compared to other particulate systems (Mosqueira et al., Citation2001; Giovino et al., Citation2013).
PL and BS are not only the shells of the nanostructures, but also considered as a source of membrane penetration enhancers in dermal and transdermal drug transport (Amsalem et al., Citation2009; El Zaafarany et al., Citation2010).
Some mucoadhesive polymers and plasticizers also have the ability to enhance drug absorption through mucosa without damaging the biological system.
Mucoadhesive buccal films were prepared by incorporating a liquid PL-BS-MMs formulation into a solid dosage form, combining the advantages of liquid PL-BS-MMs with those of a solid dosage form.
Mucoadhesive buccal films are generally constituted of plasticized hydrocolloids and blends, and can be laminated by solvent casting or hot-melt extrusion. Thus there is no problems with respect to large scale production.
Based on the above advantages, the integration of PL-BS-MMs with mucoadhesive buccal films will be an appropriate way to provide a non-invasive route of administration for Cu B. In order to improve buccal absorption, carboxymethyl chitosan (CCS) is an appropriate bioadhesive polymer which is biologically safe and well accepted as an absorption enhancer (Agnihotri et al., Citation2004; Di Colo et al., Citation2008; Duan et al., Citation2013). Glycerol can easily penetrate the skin and has also been assessed as a skin penetration enhancer in several in vitro studies (Park et al., Citation2012), thus we chose it as plasticizer in this study to obtain elegant films.
The purpose of present study was therefore to develop and characterize a CCS films (CCS-films) containing Cu B loaded PL-BS-MMs. The approach employed focused on combining PL-BS-MMs with mucoadhesive buccal films for the potential administration of Cu B across the buccal mucosa. We intend to provide a delivery device with functionalities of mucoadhesion, drug protection by avoiding passage through the gastrointestinal tract and moreover a “depot” of nanoparticles which could enhance uptake across the epithelium.
In this study Cu B loaded PL-SDC-MMs was encapsulated in the CCS-films and evaluated for the mechanical properties, mucoadhesion properties, in vitro water-uptake, in vitro release and morphological properties. Then the optimal CCS-films containing Cu B loaded PL-BS-MMs were reconstituted, characterized and compared with corresponding results of initial Cu B-PL/SDC-MMs solution. Finally, the CCS-films containing PL-BS-MMs was applied to rabbits for in vivo studies and compared with marketed tablet and conventional CCS films of Cu B.
Experimental
Materials
Cucurbitacin B (98%) was from Nantong Feiyu Biological Technology Co. Ltd. (Nantong, China); Phospholipid (PC > 95%) was obtained from Shanghai TaiWei Pharmaceutical Industry Co. Ltd. (Shanghai, China); sodium deoxycholate (SDC) was from Beijing Hotaibio Science and Technology Co. Ltd. (Beijing, China); Glycerol was purchased from Resource (boxing) Olecchemicals Co., Ltd. (China); N,O-Carboxymethyl chitosan (CCS) (carboxymethyl degree 90.5% and N-deacetylation degree 90.6%) was purchased from Qingdao Haihui Biotechnology Co. Ltd. (Qingdao, China); Cucurbitacin tablet (Hulusupian) was purchased from Hunan DiNuo Pharmaceutical Industry Co. Ltd. (Hunan, China); methanol of HPLC-grade was purchased from Promptar Ltd. (Elk Grove, CA); All other chemicals were of analytical grade and were used as received.
Preparation and characterization of CCS-films containing Cu B loaded PL-SDC-MMs
Formulation development
Preparation of Cu B-PL/SDC-MMs
The preparation of Cu B-PL/SDC-MMs was carried out by film dispersion method based on our previous researches (Lv et al., Citation2013). In the optimized formulation, the total concentration of PL/SDC was 54 mg/mL and the mass concentration ratio was 1:0.8. Briefly, PL and SDC were dissolved by anhydrous ethanol in round-bottom flask. A thin film was formed after evaporation of organic solvent by rotary evaporator (Laborota 4000, Heidolph Instruments Ltd., Germany). The film was rehydrated in a given amount of distilled water to give clear solution. Cu B (the mass concentration was 5 mg/mL) was added to this solution, nitrogen sealed and mixed 24 h on a magnetic stirrer (85-2, Guohua Instrument Co. Ltd., China) at room temperature to form clarified Cu B-PL/SDC-MMs.
Film preparation and Cu B loaded PL-SDC-MMs incorporation
The films were prepared by solvent casting method. CCS gel at various concentration was produced by dissolving the given amount of CCS in 10 mL Cu B-PL/SDC-MMs solution at room temperature under magnetic stirring. For the fabrication of films, different concentration of glycerol was added to this gel and stirred for 30 min so as to get a homogeneous system. Then it was casted on a petridish and dried at room temperature for 24 h. The composition of various films is given in .
Table 1. Composition of the CCS films.
Determination of mechanical properties
The thickness of films was determined by a calibrated Vernier caliper (Mitutoyo, Japan) at five different locations on the same film prior to mechanical tensile characterization. The mechanical properties of the films were investigated based on American Standard Testing Methods (ASTM) D882 (1991) and measured using Texture Analyzer (TA.HD. plus) (Stable Micro Systems Ltd., Surrey, UK). The elongation at break, tensile strength and elastic modulus were estimated according to Equations (Equation1–3). Results of the films for each formulation were expressed as the mean values ± SD:
(1)
(2)
(3)
Mucoadhesion properties studies
The mucoadhesive properties of the films were measured using porcine buccal mucosa as biological membrane due to the similarity to the human buccal tissue (Hoogstraate et al., Citation1992). Porcine buccal mucosa was obtained from a freshly killed pig which obtained from a local slaughter house. The tissue was stored in phosphate buffer (pH 7.4) 4 °C after collection. Then the buccal mucosa was surgically removed from the oral cavity using fine-point forceps and surgical scissors to turn away the connective tissue. Finally, buccal mucosa was cleaned in phosphate buffer (pH 7.4) and immediately used for test.
The mucoadhesive properties were measured in terms of the force needed to pull out the buccal mucosa (surface area 1 mm2) from the film with an adapted tensiometer (Krüss 132869; Hamburg, Germany). The method has been described by Luppi et al. (Citation2010) in previous studies. Briefly, the mucosa was fixed to a support with cyanoacrylate adhesive and then suspended from the tensiometer spring. The buccal mucosa was hydrated with phosphate buffer (pH 6.8) for 5 min and then lowered until it just contacted the surface of the film. A 20 dyne force was applied to the film for 60 s which was measured by the torsion balance of the instrument as a negative force. Then the buccal mucosa was raised until it was separated from the film. The assay was performed for three different circles from each film and it was expressed as the mean value.
In vitro water-uptake studies
Water uptake gives an indication of the ability of film to maintain their integrity after absorption of moisture. The study was performed in phosphate buffer (pH 6.8) which was simulated to human saliva. Circles of 2 cm2 of each film was weighted (W1) and dipped in simulated saliva fluid for 1 h. Then, the circle was wiped off from the surface water using filter paper and weighted (W2). Water-uptake ability was determined as a weight increased of the film according to the follow equation:
(4)
where W1 was the initial weight of the dry film and W2 is the weight of the hydrated film.
In vitro release studies
Release test of films was performed using Franz diffusion cells (Abruzzo et al., Citation2012). Porcine esophageal mucosa, which was obtained from a local slaughterhouse, was used as a permeation membrane. Films were placed in the donor compartment and 20 µL of phosphate buffer (pH 6.8) simulating human saliva were placed on the mucosa. The receptor medium, 6 mL of PBS (pH 7.4), was stirred constantly in a circulating water bath at a constant temperature of 37 °C. In order to assess the amount of drug released, samples of 100 µL were taken and replaced by fresh medium at predetermined time intervals.
Concentrations of the taken samples were determined by a high performance liquid chromatography (HPLC) consisted of a quaternary pump (G1100A Quat Pumpt), degasser, diode array detector (G1100A DAD) and HP Chemstation Data system (Agilent Technologies, Palo Alto, CA) (Wang et al., Citation2010; Yu et al., Citation2013). Separations were performed on a Pinnacle DB C18 column (250 mm × 4.5 mm, 5μm, Agilent) at 25 °C, protected by an Alltima C18 guard column (20 mm × 4 mm, 5 μm), and quantified by the DAD detector at 228 nm. A mobile phase was 68% (v/v) of methanol and 32% (v/v) of deionized water solution, and was delivered at flow rate of 1.0 mL/min. About 20 μL of the sample was injected into the HPLC system.
Determination of morphological properties of CCS-films
To investigate the microstructure of Cu B loaded PL-SDC-MMs and the uniformity of nanoparticles dispersed in the CCS-films, peripheral and middle portions of films were coated with a thin layer of gold and analyzed using the SEM.
Reconstitution and characterization of Cu B loaded PL-SDC-MMs based on CCS-films
The Cu B loaded PL-SDC-MMs based on CCS-films were reconstituted by adding water to obtain a colloidal system. Reconstitution was tested both by simple shaking and string for 30 min. After reconstitution, the MMs solutions were characterized and compared with the corresponding data of initial Cu B-PL/SDC-MMs.
Determination of encapsulation efficiency
The content of the Cu B was determined by HPLC as described above. To determine the level of encapsulated Cu B in MMs, aqueous solution of MMs was centrifuged at 16 000 × g (Allegra X-22 R Centrifuge, F2402H rotor, Beckman Coulter Inc, CA) for 10 min to separate MMs-incorporated and free drug. Then 50 µL aliquot of the MMs solution was diluted in 0.95 mL methanol to disrupt the micellar structure and the incorporated drug was released. The solution was injected to HPLC after filtration with a 0.45 μm membrane filter. The encapsulation efficiency (EE%) was calculated by the following equation:
(5)
Measurement of particle size
The particle size and polydispersity index (PI) of Cu B-PL/SDC-MMs were determined by photon correlation spectroscopy (PCS) using a Zeta-sizer (3000SH, Malvern Instruments Ltd., UK). Before measurement, Cu B-PL/SDC-MMs were diluted with bidistilled water to achieve required dilution for PCS analysis. Each sample was measured at a fixed angle of 90° at 25 °C in triplicate.
Determination of morphological properties of Cu B-PL/SDC-MMs
The morphology of optimum Cu B-PL/SDC-MMs was observed with the transmission electron microscope (TEM) (Tecnai G 2 F30, FEI, Eindhoven, The Netherlands). The sample was placed on copper grids. After being air dried, the films were observed by TEM.
Tissue viability assay
Tissue viability was assessed using the 3-[4,5-dimethylthiazol-2-yl] 2,5-diphenyltetrazolium bromide (MTT) reduction assay. The porcine buccal mucosa, which was same as 2.2.3, was used in this study. The mucosa tissue inserts were placed in contact with the the CCS-films containing Cu B loaded PL-SDC-MMs for 36 h after which the tissue inserts were transferred into 24-well plates pre-filled with MTT (0.3 mL) dissolved in PBS (5 mg/mL), incubated for 3 h and the MTT medium was gently aspirated from all wells. Then, the cultures were extracted into 2 mL of acidified isopropanol for 2 h with gentle shaking. Two aliquots each of extract (200 µL) were placed into two separate wells of a 96-well plate and the absorbance of the extracted formazan was determined using the Multiskan EX microplate photometer equipped with Ascent software (Thermo Scientific, Hampshire, UK) at 570 nm. The highest absorbance values were obtained for viable cells which had the greatest amount of MTT reduction. Data were processed from three replicates for the mean percentage viability.
In vivo study in rabbit
The studies were approved by the Animal Ethics Committee of China Military Institute of Chinese Materia Medic. Healthy, male rabbits weighing ∼2.5 ± 0.2 kg were used as animal model because its buccal membrane closely resembles the human buccal membrane in structure and permeability (Navneet & Pronobesh, Citation2012). The rabbits selected for study were housed in separate cages and had no medication for 2 weeks prior to the study. They were fasted during the study. The animals were divided randomly into three groups, and each groups had five animals.
Conventional CCS films (C-CCS-films) without nanoscale Cu B was also prepared as a control, containing the same amount of ingredients as the optimal formation and following the procedure described as follow: CCS gel at determined concentration was produced by dissolving the required amount of CCS in 10 mL distilled water of acetic acid 1% (v/v) at room temperature under magnetic stirring. For the fabrication of the drug loaded films, SDC, PL, glycerol, Cu B were added to this gel, respectively, stirred for 30 min so as to get a homogeneous system and then followed the same procedure described previously.
Three groups received the following dosage forms, respectively: the CCS-films containing Cu B loaded PL-SDC-MMs, the C-CCS-films and the conventional tablet (Hulusupian), all corresponding to a dose of 1 mg/kg. The CCS-films and C-CCS-films were applied to buccal mucosa of the rabbits and a gentle pressure was applied with a finger for 1 min to ensure good adhesion to the mucosa. While the tablet was administered as suspension orally using oral feeding sonde. The rabbits were placed on a surgery table, 2 mL of blood was collected from the ear vein at predetermined time for 36 h, and after centrifuging, the serum separated was stored at −20 °C until analyzed.
To assay the Cu B concentration in plasma, the HPLC system was used (Li et al., Citation2001). Briefly, the flow rate and UV wavelength were 1.0 mL/min and 228 nm, respectively. The mobile phase was methanol-H2O (64:36, v/v). Cu B and the internal standard (methylnorethindrone) were extracted from plasma using a simple protein precipitation procedure with 4-fold acetonitrile, followed by centrifugation at 10 000 rpm for 20 min. The organic phase was collected and dried by blowing nitrogen at 30 °C. The dried residue was dissolved in 0.1 mL of methanol, and was injected into HPLC system.
The plasma concentration of Cu B at different time intervals was subjected to PK analysis to calculate various parameters: maximum plasma concentration (Cmax), time to reach maximum concentration (Tmax) and area under the plasma concentration–time curve (AUC0–36h). The relative bioavailability (F) of Cu B after the buccal delivery versus the oral administration was calculated as follows:
(6)
Pharmacokinetic analysis was performed by using statistics software (DAS® 2.0, Boying Corporation, China). Data were subjected to a one-way analysis of variance (ANOVA) followed by the least significant difference (LSD) post hoc test. Results were presented as a mean and standard error of mean.
Result and discussion
Preparation and characterization of CCS-films containing Cu B loaded PL-SDC-MMs
Formulation development
In previous study (Boateng et al., Citation2009), it was demonstrated that the addition of plasticizer imparts flexibility to films with increased thickness, which makes for easy removal without damage to film configuration. Thus glycerol was used in all formulated films resulting in optimal plasticized films. The films were prepared according to and evaluated for film forming capacity, mechanical properties, mucoadhesive properties and in vitro water-uptake ability as shown in . As can be seen, all trials have average or good film forming capacity. It was suggested that it might be due to the characteristic nature of film-formers. There are a lot of hydrophilic groups such as –NH2, –OH and –COOH groups on the CCS backbone, these groups can form a hydrogen band with glycerol molecules, and interrupts inter and intra-molecular H-bonds between the polymer chains with consequent partial disintegration of the H-bonded network structure (Bajdik et al., Citation2009; Duan et al., Citation2013). The elongation at break increased upon the increasing concentration of plasticizer in trial 4, which stresses the importance of addition of plasticizer. When the concentration of glycerol increased from 0.25% to 0.4%, the tensile strength decreased from 49.2 to 38.9 N as shown in (trial 3 and trial 4), which indicates that the increasing concentration of plasticizer might decrease the tensile strength. Values of elastic modulus indicates toughness of the film. The elastic modulus values increased upon the increasing concentration of polymer. But the values of elastic modulus was decreased upon the increasing concentration of plasticizer in trial 4 indicating that addition of plasticizer might reduce toughness of the film to a certain extent. However, it was within the acceptable range. Films with the more amount of CCS seems to show the better in vitro mucoadhesive properties among all films. Moreover, films containing the more glycerol, seems to show the better mucoadhesion properties. In fact, in addition to ionic interaction, glycerol promoted the entry of water, a more efficient chain mobility and physical entanglement with mucus (Abruzzo et al., Citation2012). The water-uptake ability was increased with the presence of a higher amount of polymer. As previously described the presence of free charges in the formulation is extremely important for film hydration and greater amount of polymers in the films can further improve water-uptake ability. As can be expected, films with a higher amount of glycerol (trial 4) showed a greater water-uptake ability than other films, due to the presence of a hydrophilic molecule able to favor a major entry of water in the system.
Table 2. Evaluation of trials (mean ± SD, n = 3).
As shown in , the release of Cu B across porcine buccal mucosa from various films was tested for 5 h near the biological pH of saliva and the release of Cu B increased as the CCS concentration increased from 1% to 1.5%. It`s due to the absorption enhancing ability of CCS (Senel et al., Citation2000; van der Lubben et al., Citation2001). The amount of released Cu B from the films prepared with 1.5% CCS (trial 3 and trial 4) reached plateau faster than those from the films prepared with 1% and 1.25% CCS (trial 1 and trial 2). The result indicated that the binding forces in the matrices of the films prepared with 1% and 1.25% CCS are relatively weaker than that of 1.5% CCS films (trial 3 and trial 4).
Figure 1. Release profiles of Cu B from CCS-films prepared by trial 1, trial 2, trial 3 and trial 4 (n = 6, mean ± SD).
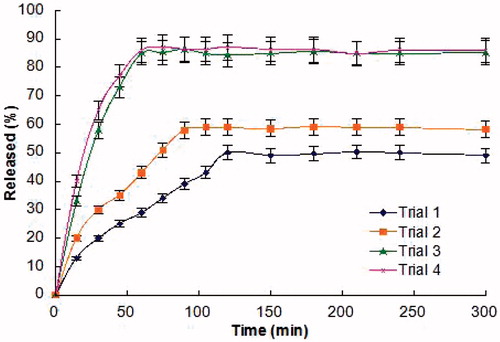
After comprehensive evaluation of all results, trial 4 was optimized as the final formulation.
Structural morphology by SEM
For a good representation of nanoparticles in the CCS-films as well as to examine its distribution in the films, SEM studies were performed on the peripheral and middle portions of final formulated films. The SEM images of trial 4 are shown in . In the formulated films, the CCS network formed a dense continuous sheet with no fractures and the films exhibited an unequivocally uniform distribution of nanoparticles in both peripheral and middle portions. These results suggest that CCS-films containing Cu B loaded PL-BS-MMs have been successfully prepared.
Reconstitution and characterization of Cu B loaded PL-SDC-MMs based on CCS-films
After the addition of water and shaking, the formulation became immediately as clear as the initial MMs solution. The EE (%), particle size analyses and MMs morphology by HPLC, PCS and TEM after reconstitution were carried out, respectively. These data are summarized and compared with corresponding data of the initial MMs solution. The comparison results are presented in . Regardless of slight variation, the MMs sizes and EE was not affected by the film-forming procedure. This CCS-films containing Cu B loaded PL-SDC-MMs was easily reconstituted in a transparent and clear solution. Both the initial MMs solution and the reconstituted solution are spherical micelles in the submicron range as presented in . All of these results suggest that the Cu B loaded PL-SDC-MMs have been successfully prepared by incorporating it into CCS-films and the desired nanometer particle size has been retained during the film-forming process.
Figure 3. Transmission electron micrographs of Cu B-PL/SDC-MMs. The images of the initial MMs solution and reconstituted MMs solution are represented on the left and right side, respectively (scale bar = 100 nm).
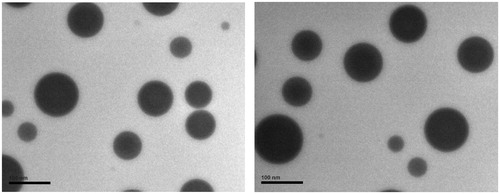
Table 3. MMs sizes and EE of the initial MMs solution and reconstituted MMs solution (mean ± SD, n = 3).
The structure of PL-BS-MMs is widely accepted to be a “mixed disk model” (Mazer et al., Citation1980; Hammad & Müller, Citation1998; Jin et al., Citation2012). In the aqueous environment, the hydrophilic surfaces of disk form the outer shell of the MMs while the hydrophobic surfaces form the core. The outer shell formed by hydrophilic blocks acts as a stabilizing interface between the hydrophobic core and the external medium (Allen et al., Citation1999). And van der Waals interactions between the exposed cores are responsible for aggregation of micelle particles resulting in the formation of large clusters (La et al., Citation1996). The high degree of hydration and large excluded volume of hydrophilic blocks induce repulsive force which competes with the interparticle van der Waals attractive force. Aggregation will be prevented if the repulsive force overwhelm the attractive force operative between the particles (Lee et al., Citation1995). The amount of core material that remains exposed to the aqueous medium will be influenced by the surface density of the hydrophilic blocks in the shell. So the extent to which the hydrophilic block corona could sterically stabilize the micelle depends on the surface density of hydrophilic block on the hydrophobic core. Similar results were reported by Yang et al. (Citation2008). In the film-forming procedure, CCS and glycerol were added to the CCS-films as the polymer and plasticizer, respectively. Both of the film formers have good solubility in water and provide a large number of hydrophilic blocks which could sterically stabilize the micelle in turn. Thus, the multiple functions of ingredients make it possible to reconstitute PL-SDC-MMs with favorable characteristics.
Tissue viability
Average percentage viability was determined for the CCS-films containing Cu B loaded PL-SDC-MMs using non-treated control as the 100% viable tissue. The results showed that the buccal mucosa tissue inserts maintained >95% viability, indicating that the CCS-films containing Cu B loaded PL-SDC-MMs had no toxic effect on the cells of the porcine buccal mucosa tissue construct.
In vivo study of the optimal formulation
Plasma concentration–time profiles of Cu B in different formulations to the rabbits were illustrated in . The pharmacokinetic parameters are listed in . Tmax was found to be 5.03 ± 0.34 h from CCS-films containing Cu B loaded PL-SDC-MMs while it was ∼7.96 ± 0.97 h from C-CCS-films. On the other hand, Tmax from Cu B tablet was only 3.01 ± 0.82 h. Compared to the Cu B tablet, the Tmax from CCS-films and C-CCS-films were higher significantly (p < 0.05) and the Cmax from CCS-films and C-CCS-films were lower significantly (p < 0.05). The low Cmax and prolonged Tmax observed with the buccal delivery formulations in contrast to the oral administration can be attributed to the efficient barrier properties of the buccal mucosa.
Figure 4. Plasma concentration-time profile of Cu B in rabbits after administration of 1 mg/kg Cu B in different formulations. Each point represents the mean ± SD of five experiments.
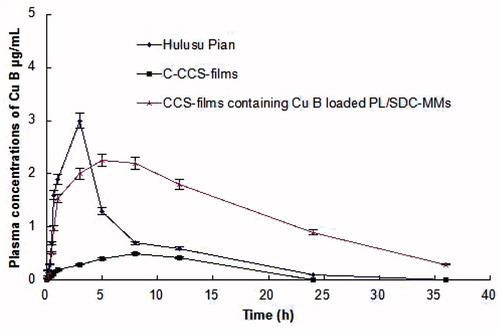
Table 4. Pharmacokinetic parameters after oral administration of Cu B formulations to rabbits (mean ± SD, n = 5).
The in vivo studies revealed greater extent of absorption from nanoscale CCS-films formulations than the tablet and C-CCS-films of Cu B. The observed difference in AUC0–36h from CCS-films containing Cu B loaded PL-SDC-MMs was found to be significant as compared to tablet and C-CCS-films (p < 0.05). The significantly high AUC0–36h value observed with CCS-films containing Cu B loaded PL-SDC-MMs indicated increased bioavailability of the drug as compared to oral administration. The absorption of Cu B from CCS-films containing Cu B loaded PL-SDC-MMs resulted in 2.69-fold increased in bioavailability as compared to conventional tablet formulation and 10.46 times with reference to the C-CCS-films formulation. The higher absorption achieved with respect to the nanoscale CCS-films formulation might be due to the presence of efficient permeation enhancers in the form of the shells of PL-SDC-MMs and the film-formers in them. Also, the drug was present in nanoscale particle size which resulted in better permeation when compared with C-CCS-films where the drug was in larger size. The small particle size ensures close contact to the buccal epithelium thus increasing the amount of drug penetrating into the blood. Increased bioavailability from nanoscale CCS-films formulation in comparison to oral tablet formulation might be due to the avoidance of the hepatic first pass metabolism.
Conclusions
In conclusion, the mucoadhesive Cu B buccal delivery system was developed using CCS as a bioadhesive polymer, glycerol as a plasticizer and PL-SDC-MMs as the nanoscale carriers. The optimized Cu B buccal film showed appropriate physical characteristics and in vitro release profile. The buccal administration of Cu B to rabbits showed that nanoscale CCS-films formulation of Cu B had good pharmacokinetic features which can replace oral dosage form for the same. This kind of mucoadhesive buccal film might be an alternative safe route for delivery of Cu B with better patient compliance and higher bioavailability for the treatments.
Declaration of interest
This study was supported by the National Key New Drugs Innovation Foundation (No. 2012ZX09J12108-04C and No. 2013ZX09J13109-06C) and the Scientific Research Foundation for the Returned Overseas Chinese Scholars, State Education Ministry (No. 20101561).
References
- Abruzzo A, Bigucci F, Cerchiara T, et al. (2012). Mucoadhesive chitosan/gelatin films for buccal delivery of propranolol hydrochloride. Carbohydr Polym 87:581–8
- Agnihotri SA, Mallikarjuna NN, Aminabhavi TM. (2004). Recent advances on chitosan-based micro- and nanoparticles. J Control Release 100:5–28
- Alkan-Onyuksel H, Ramakrishnan S, Chai HB, Pezzuto JM. (1994). A mixed micellar formulation suitable for the parenteral administration of taxol. Pharm Res 11:206–12
- Allen C, Maysinger D, Eisenberg A. (1999). Nanoengineering block copolymer aggregates for drug delivery. Colloids Surf B Bio-interf 16:3–27
- Amsalem O, Yuli-Amar I, Aserin A, Garti N. (2009). Phospholipids embedded fully dilutable liquid nanostructures. Part 1: compositions and solubilization capacity. Colloids Surf B Biointerfaces 73:15–22
- Bajdik J, Marciello M, Caramella C, et al. (2009). Evaluation of surface and microstructure of differently plasticized chitosan films. J Pharm Biomed Anal 49:655–9
- Bartalis J, Halaweish FT. (2005). Relationship between cucurbitacins reversed-phase high-performance liquid chromatography hydrophobicity index and basal cytotoxicity on HepG2 cells. J Chromatogr B Analyt Technol Biomed Life Sci 818:159–66
- Boateng JS, Stevens HNE, Eccleston GM, et al. (2009). Development and mechanical characterization of solvent-cast polymeric films as potential drug delivery systems to mucosal surfaces. Drug Dev Ind Pharm 35:986–96
- Di Colo G, Zambito Y, Zaino C. (2008). Polymeric enhancers of mucosal epithelia permeability: synthesis, transepithelial penetration-enhancing properties, mechanism of action, safety issues. J Pharm Sci 97:1652–80
- Duan B, Sun P, Wang X, Yang C. (2013). Preparation and properties of starch nanocrystals/carboxymethyl chitosan nanocomposite films. Starch 63:528–35
- El Zaafarany GM, Awad GA, Holayel SM, Mortada ND. (2010). Role of edge activators and surface charge in developing ultradeformable vesicles with enhanced skin delivery. Int J Pharm 397:164–72
- Giovino C, Ayensu I, Tetteh J, Boateng JS. (2013). An integrated buccal delivery system combining chitosan films impregnated with peptide loaded PEG-b-PLA nanoparticles. Colloids Surf B Biointerfaces 112:9–15
- Hammad MA, Müller BW. (1998). Increasing drug solubility by of bile salt-phosphatidylcholine-based mixed micelles. Eur J Pharm Biopharm 46:361–7
- Hoogstraate AJ, Cullander C, Nagelkerke JF, et al. (1992). Diffusion rates and transport pathways of FITC-labelled model compounds through buccal epithelium. Pharma Res 11:83–9
- Hu H, Liu D, Zhao X, et al. (2013). Preparation, characterization, cellular uptake and evaluation in vivo of solid lipid nanoparticles loaded with cucurbitacin B. Drug Dev Ind Pharm 39:770–9
- Jin S, Fu S, Han J, et al. (2012). Improvement of oral bioavailability of glycyrrhizin by sodium deoxycholate/phospholipid-mixed nanomicelles. J Drug Target 20:615–22
- La SB, Okano T, Kataoka K. (1996). Preparation and characterization of the micelle-forming polymeric drug indomethacin-incorpo-rated poly(ethylene oxide)-poly(a-benzyl L-aspartate) block copolymer micelles. J Pharm Sci 85:85–90
- Lee JH, Lee HB, Andrade JD. (1995). Blood compatibility of polyeth-ylene oxide surfaces. Prog Polym Sci 20:1043–79
- Li CY, Hou SX, Yang K, Mao SJ. (2001). Study on quantitative determination of cucurbitacins B in plasma by HPLC. Chin Pharm J 36:557–9
- Luppi B, Bigucci F, Cerchiara T, Zecchi V. (2010). Chitosan-based hydrogels for nasal drug delivery: From inserts to nanoparticles. Expert Opin Drug Deliv 7:811–28
- Lv QY, Li XY, Shen BD, et al. (2013). A solid phospholipid-bile salts-mixed micelles based on the fast dissolving oral films to improve the oral bioavailability of poorly water soluble drugs. J Nanopart Res (under review)
- Mazer NA, Benedek GB, Carey MC. (1980). Quasielastic light-scattering studies of aqueous biliary lipid systems. Mixed micelle formation in bile salt-lecithin solutions. Biochemistry 19:601–15
- Molavi O, Ma Z, Mahmud A, et al. (2008). Polymeric micelles for the solubilization and delivery of STAT3 inhibitor cucurbitacins in solid tumors. Int J Pharm 347:118–27
- Mosqueira VC, Legrand P, Gulik A, et al. (2001). Relationship between complement activation, cellular uptake and surface physicochemical aspects of novel PEG-modified nanocapsules. Biomaterials 22:2967–79
- Navneet V, Pronobesh C. (2012). Preparation of mucoadhesive patches for buccal administration of metoprolol succinate: in vitro and in vivo drug release and bioadhesion. Trop J Pharma Res 11:9–17
- Oh H, Mun YJ, Im SJ, et al. (2002). Cucurbitacins from Trichosanthes kirilowii as the inhibitory components on tyrosinase activity and melanin synthesis of B16/F10 melanoma cells. Planta Med 68:832–3
- Park DM, Song YK, Jee JP, et al. (2012). Development of chitosan-based ondansetron buccal delivery system for the treatment of emesis. Drug Dev Ind Pharm 38:1077–83
- Senel S, Kremer MJ, Kas S, et al. (2000). Enhancing effect of chitosan on peptide drug delivery across buccal mucosa. Biomaterials 21:2067–71
- Tannin-Spitz T, Grossman S, Dovrat S, et al. (2007). Growth inhibitory activity of cucurbitacin glucosides isolated from Citrullus colocynthis on human breast cancer cells. Biochem Pharmacol 73:56–67
- Ukiya M, Akihisa T, Yasukawa K, et al. (2002). Anti-inflammatory and anti-tumor-promoting effects of cucurbitane glycosides from the roots of Bryonia dioica. J Nat Prod 65:179–83
- van der Lubben IM, Verhoef JC, Borchard G, Junginger HE. (2001). Chitosan and its derivatives in mucosal drug and vaccine delivery. Eur J Pharm Sci 14:201–7
- Wang W, Zhao X, Hu H, et al. (2010). Galactosylated solid lipid nanoparticles with cucurbitacin B improves the liver targetability. Drug Deliv 17:114–22
- Yang ZL, Li XR, Yang KW, Liu Y. (2008). Amphotericin B-loaded poly(ethylene glycol)-poly(lactide) micelles: Preparation, freeze–drying, and in vitro release. J Biomed Mater Res A 85A:539–46
- Yu C, Han J, Yuan X, et al. (2013). Improved bioavailability and reduced adverse drug reaction of poorly soluble cucurbitacin B by sodium deoxycholate/phospholipid mixed micelles. J Biomed Nanotechnol (under review)