Abstract
The aim of this research was to increase the oral bioavailability of puerarin by N-trimethyl chitosan-modified microemulsions (TMC-MEs) loaded with puerarin. Different concentrations of TMC-modified microemulsions were prepared in our study, and then evaluated for particle size, zeta potential, morphological observation and changes of the microenvironment polarity of inner oil core. It was shown that the zeta potential of the microemulsion was increased with the increasing concentration of TMC, and the peak value was achieved when the concentration of TMC was 3.0 mg/mL. The enhancement of the ratio of I1/I3 (the ratio between the first band and the third band of the emission fluorescence spectrum of pyrene, I1 = 373 nm, I3 = 384 nm) indicated that polarity of the inner core of TMC-MEs was increased with the addition of the modifier. Pharmacokinetic studies demonstrated that after oral administration of puerarin N-trimethyl chitosan (TMC)-modified microemulsions (PUE-TMEs) and puerarin microemulsions (PUE-MEs) to rats at a dose of 100 mg/kg, relative bioavailability was enhanced about 6.8- and 1.2-fold, respectively, compared to puerarin suspension (PUE-SUS) as control. It indicated that the TMC-MEs could be used as an effective formulation for enhancing the oral bioavailability of puerarin.
Introduction
Puerarin (PUE) is a naturally occurring isoflavone C-glycoside, isolated from the root of Pueraria lobata (Willd.) Ohwi, which is used in the treatment of cardiovascular diseases, such as coronary heart disease, cardiac infarction, arteriosclerosis and arrhythmia (Ren et al., Citation2006; Yeung et al., Citation2006). However, it was reported that oral bioavailability of PUE was just approximately 4%, the cumulative excretion rate of unchanged PUE in urine and feces was 45.33% in 24 h and most of it was mainly excreted by dejection (Gou et al., Citation2006; Luo et al., Citation2010). Thus, it is very necessary to develop an effective drug delivery system to improve the bioavailability of PUE in vivo.
Among the various drug delivery systems, microemulsions may be a better choice to solve this problem. Microemulsions are defined as monodispersion spherical droplets consisting of oil, surfactant, co-surfactant and aqueous phase, with high drug solubilizing capacity, long-term stability and capability to enhance bioavailability of both hydrophobic and hydrophilic drugs (Yin et al., Citation2009). Based on our previous studies, employing microemulsion formulation improved oral bioavailability of PUE as indicated in its AUC value which was more than 1.2 times larger compared to that of its suspension. On this basis, we further develop a new type of modified-microemulsions that could significantly improve the metabolic process of PUE. The crucial problem was the search for an appropriate modifier to increase the intestinal tract absorption of the PUE microemulsions (PUE-MEs) and therefore improve the bioavailability of PUE.
Among various modifiers, N-trimethyl chitosan chloride (TMC) has been widely used as an absorption enhancing agent in the new delivery systems of peptides, proteins and other macromolecules (Pakhomov et al., Citation2010; Katrin & Shlomo, Citation2012). TMC is a partially quaternized derivative of chitosan, characterized by superior water solubility and permeation enhancing effect in neutral environment (Amidi et al., Citation2006; Florea et al., Citation2006). TMC could enhance the absorption of macromolecules through mucosal epithelia by triggering the reversible opening of tight junctions. Moreover, TMC can be incorporated into drug formulations easily, leading to the enhanced intestinal absorption of drug molecules (Amidi et al., 2010). Therefore, we successfully prepared TMC-modified microemulsions (TMC-MEs) that would facilitate the intestinal absorption process of PUE, thereby further increasing its oral bioavailability.
Taking advantage of the intestinal absorption enhancing effect of TMC, TMC-MEs were evaluated as potential vehicles to improve the oral bioavailability of PUE, a more challenging drug delivery system for poor intestinal absorption.
Materials and methods
Materials
PUE (98% purity) was purchased from Nanjing Zelang Chinese Medicine Science and Technology Co, Ltd (Nanjing, China). Chitosan was obtained from Shandong Qingdao Haipu Biotechnology Co, Ltd (Qingdao, China). N-trimethyl chitosan chloride (TMC) was synthesized by our laboratory (). Ethyl oleate, isopropyl myristate, ethyl acetate, ethanol, propylene glycol, methyl pyrrolidone, sodium hydroxide, methyl iodide and sodium iodide were purchased from Sinopharm Group Chemical Reagent Co., Ltd (Shanghai, China). Polyoxyl 40 hydrogenated castor oil (Cremophor RH40) was donated by BASF Co., Ltd (Ludwigshafen, Germany). Lecithin with a phosphatidylcholine (PC) content of (70–97%) was obtained from Shanghai Tai-wei Pharmaceutical Co., Ltd (Shanghai, China). Cycloheximide was purchased from Sigma Chemical Co., Ltd (St Louis, MO, USA). HPLC grade acetonitrile and methanol was purchased from Fisher (Fair Lawn, NJ, USA). All other reagents were analytical grade and obtained through commercial sources. Super-pure water was obtained following distillation in glass and passage through a Milli-Q system (Millpore, USA) and was used to prepare all solutions.
Synthesis and characterization of TMC
As the paper reported before (Florea et al., Citation2006), TMC with the quaternization degree of 55% was synthesized by a two-step methylation procedure. Briefly, low molecular weight chitosan was dispersed in a basic solution of methyl pyrrolidone and sodium hydroxide. Methyl iodide and sodium iodide were, subsequently, added at 60 °C for 1 h. The polymer was then precipitated with acetone and separated by centrifugation. The aqueous NaCl (5.0%, w/w) solution was used to exchange I− with Cl−, subsequently, precipitated with acetone and dried to obtain a water-soluble white powder with the quaternization degree of 55%. The 1H NMR spectrum was obtained in D2O using a 500 MHz spectrum and the degree of quaternization (DQ) was calculated according to the following formula. showed the 1H NMR spectra of TMC, the trimethyl peak(-NH+(CH3)3) was observed at 3.35 ppm, the signal at 5.5–5.9 ppm corresponds to anomeric proton located in C1. According to the peak assignment, the quaternization degree of 55% could be obtained by calculation based on the formula. TMC had a higher positive charge and better solubility, which was suitable for the experiment.
Preparation of microemulsions
The mixture was blended until the oil (ethyl oleate) and the surfactant (RH40)/co-surfactant (1,2-propanediol) were completely mixed. The weight ratio of RH40 to 1,2-propanediol was 2:1, and then, a series of concentration-gradient (1.0, 1.5, 3.0, 4.5 mg/mL) TMC solutions were added to the mixture solutions drop by drop and stirred until obtaining a clear solution, the resultant suspension was transparent in appearance with opalescence of blue, consequently facilitating the formation of O/W TMC-modified microemulsions (TMC-MEs). The conventional microemulsions(C-MEs) were prepared in the similar way, just without the addition of TMC. To prepare drug-loaded microemulsions, excessive PUE powder was added to the pre-formed TMC-MEs and C-MEs, and the solution was stirred to ensure homogeneity. Excessive puerarin was removed by centrifugation at 3000 rpm for 10 min. The concentration of PUE in TMC-MEs and C-MEs was determined by high performance liquid chromatography (HPLC) as described by Liu and Xiang (Citation2007). The results showed that drug loading in TMC-MEs and C-MEs was 10.67 mg/mL and 8.82 mg/mL.
Physicochemical characterization of C-MEs and TMC-MEs
The newly prepared C-MEs and TMC-MEs were filtered through a 0.22 μm organic membrane, the droplet size and zeta potential were determined by dynamic light scattering (DLS) using a Zetasizer Nano ZS90 instrument (Malvern Instruments Ltd, Malvern, UK). The particle size, particle size distribution, and aggregation state of the C-MEs and TMC-MEs were characterized by freeze-etching replication transmission electron microscope (FER-TEM; Leica, Wetzlar, Germany).
Evaluation of microenvironment polarity of the inner core
Pyrene, a widely used fluorescence probe, which was particularly attractive because of its relatively long fluorescence lifetime, and the ability to measure the polarity of its microenvironment. When transferred from a polar to a nonpolar environment, the intensity ratio (I1/I3) of the first and third band in its emission spectrum decreased remarkably (Capec et al., Citation2003).
Pharmacokinetic studies
The animal study protocol was reviewed and approved by the Animal Ethics Committee at the Second Xiangya Hospital of Central South University. Male Sprague-Dawley rats (220–250 g) were supplied by Hunan Laboratory Animal Center (Hunan, China). The healthy male SD rats were randomly divided into three groups, each animal was fasted overnight with free access to water before drug administration. Rats in the control group were orally administered with the puerarin (100 mg/kg) suspended (PUE-SUS), the other groups were administered with 3.0 mg/mL TMC-modified microemulsions loaded with puerarin (PUE-TMEs) and puerarin microemulsions (PUE-MEs) at the same dose of the control group. At designated time points after dosing, blood samples (0.4 mL) were collected from the retro-orbital plexus of rats under light ether anesthesia into microcentrifuge tubes containing heparin as anticoagulant at 0, 0.083, 0.166, 0.333, 0.75, 1, 1.5, 2, 4, 6, 8, 12 h post-dosing. Then all the blood samples were centrifuged at 3500 rpm for 5 min to obtain 100 μL plasma, they were stored at −80 °C until analysis within a week.
Statistics
Results are expressed as mean ± SD. Statistical significance was tested on the basis of Student’s t test at 95% confidence intervals.
Result and discussion
Characterization of TMC-MEs and C-MEs
The observed particle size of C-MEs and TMC-MEs were shown in . With the addition of different concentrations of TMC, the particle size increased slightly, that is to say, TMC was merged with the aqueous phase, without hindering the formation of the microemulsions, rather than the coating process reported as Wei et al. (Citation2011).
Table 1. Particle size of C-MEs and TMC-MEs.
Zeta potential is a representation of repulsive force between dispersed particles and provides insight into the possibility of particle aggregation. Higher the zeta potential, greater is the repulsion, which in turn reduces aggregation of the particles (Nair et al., Citation2012).
In this study, the zeta potential was enhanced with the increase of the concentration of TMC, and the highest value was achieved when the concentration of TMC was 3.0 mg/mL, the data was shown in . The higher zeta potential of TMC-MEs may be attributed to intrinsic positive charge of TMC. As we all know, TMC is a quaternized derivative of chitosan which provides positive charged ammonium group, therefore, TMC-MEs could be decorated with positive charge effectively. The high zeta potential particle is not only good for the stable of the microemulsions, but also contributes to the adhension with the negatively charged intestinal mucosal, which is a prerequisite for the absorption of the drug in intestinal.
TEM was often used to have an insight into the morphology of the particles. According to the TEM results shown in , C-MEs looked round to spherical in shape and had a smooth surface, the size was consistent with the detected results by the Zetasizer Nano ZS90 instrument. The morphology of low concentration TMC-MEs showed no much difference with the C-MEs. In contrast, the morphology of microemulsions with high TMC presented irregular spherical structure, especially when the concentration of TMC was 4.5 mg/mL, the particles got crosslinked to a certain extent. This irregular and aggregation state may be attributed to a strong interaction between polyions, occurring rapidly by random incorporation of different polymeric chains into the particle structure (Sarmento et al., Citation2007).
Evaluation of microenvironment polarity of the inner core
The fluorescence spectra of pyrene in C-MEs and TMC-MEs had been measured, as shown in , the fluorescence emission bands of I1 and I3 were 373 nm and 384 nm, respectively, which were consistent with the earlier report (Kalyanasundaram & Thomas, Citation1977), the results were concluded in . In the C-MEs, the value of I1/I3 was just 0.85, lower than that of the TMC-MEs, and the values increased with the concentration of TMC as a whole. Recent literature showed that the value of I1/I3 was lowest in the oil phase, and it was the highest in aqueous phase (Shaq et al., 2001). It implied that the microenvironment polarity of the inner core was higher in the TMC-MEs, then we can speculate that part of TMC molecules could pass through the barrier which formed by RH40 and 1,2-propanediol to lower interfacial tension between the oil core and the aqueous phase outside, and even penetrate into the oil core, that is to say, the addition of the TMC could go into the inner core and increase the polarity of the oil core microenvironment.
Table 2. The results of the value of I1 and I3 in different microemulsions (n = 5,
± s).
Pharmacokinetic studies
Plasma concentration–time curves of PUE were shown in . The relevant pharmacokinetic parameters, including Tmax, Cmax, AUC, t1/2 and MRT, were summarized in . Compared with the PUE-SUS group, both of the PUE-TMEs and PUE-MEs treated rats groups had higher concentrations of PUE in plasma during 1–24 h and the area under the plasma concentration-time curve (AUC) was significantly increased by 6.8 and 1.20 times, respectively. In addition, the half-life period (t1/2) of PUE-TMEs and PUE-MEs were both longer than that of the PUE-SUS group. The improvement of the relative bioavailability of the PUE-TMEs could be explained by the following reasons. To begin with, the strong positive charge of the TMC-MEs could prolong the gastric residence time for the dosage forms as well as facilitate the intimate contact with underlying absorptive surface to enhance the oral bioavailability of drugs. On the other hand, as a result of the interaction of the positively charged amino group on the TMC with the negatively charged cell surface, the F-actin and Claudin between cells will be redistributed, which trigger the opening of the tight junctions to allow paracellular transport of drugs and the tight junctions could be opened nearly 20 nm in width (Marttin et al., Citation1999; Adamson et al., Citation2004). Thirdly, lymphatic absorption was a major way to promote the absorption of micoemulsions and the uptake of the particulates by the Peyer’s patch was found to be significantly more when the zeta potential was high, so the bioavailability of puerarin could be increased by TMC-MEs. It was very interesting that both the pharmacokinetics curve of PUE-TMEs and PUE-MEs revealed a double peak phenomenon which was probably associated with the hepatoenteral circulation of PUE in microemulsion drug delivery system. It may also be related to drug release rate in the microemulsion or mult-sites absorption of microemulsion. The elucidation of the details of this phenomenon require further research.
Figure 5. Plasma concentration-time profiles of puerarin in rats after an oral administration of PUE-MEs, PUE-TMEs and PUE-SUS (n = 6, ± s).
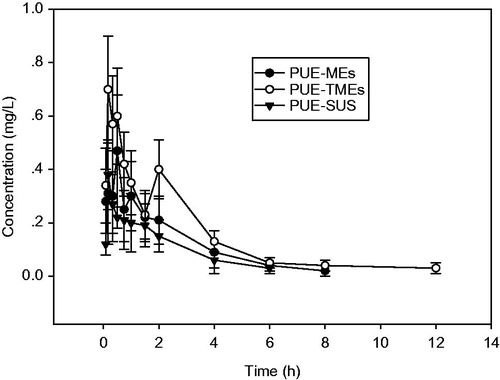
Table 3. The main pharmacokinetic parameters of puerarin in rats after an oral administration of PUE-MEs, PUE-TMEs and PUE-SUS (n = 6,
± s).
Conclusions
TMC-MEs were successfully prepared and characterized for particle size and morphology observation and the results showed that TMC incorporated into the microemulsions well. The higher value of I1/I3 in TMC-MEs indicated that parts of TMC could insert into the phase film or even go into the inner oil core. The oral relative bioavailability study showed that TMC-MEs showed higher AUC value and a prolonged residence time of PUE in the blood circulation as compared to PUE-MEs and PUE-SUS. These results suggested that TMC could be used as an absorption enhancer to increase the intestinal absorption of microemulsions and improve oral bioavailability of puerarin effectively.
Notice of Correction
Since the original online publication of this article on January 27th 2014 the affiliations have been altered. The publishers apologise for this error.
Declaration of interest
The authors report no conflicts of interest in this work. This work is supported by Hunan Provincial Natural Science Foundation (08JJ3053).
References
- Adamson RH, Lenz JF, Zhang X, et al. (2004). Oncotic pressures opposing filtration across non-fenestrated rat microvessels. J Physiol 557:889–907
- Amidi M, Romeijn SG, Borchard G, et al. (2006). Preparation and characterization of protein-loaded N-trimethyl chitosan nanoparticles as nasal delivery system. J Control Release 111:107–16
- Capec I, Liaw DJ, Huang CC. (2003). Partitioning of unsaturated hydrophilic monomer in microemulsion media monitored by pyrene fluorescence method. J Polym Sci Pol Phys 41:571–81
- Florea BI, Thanou M, Junginger HE, Borchard G. (2006). Enhancement of bronchial octreotide absorption by chitosan and N-trimethyl chitosan shows linear in vitro/in vivo correlation. J Control Release 110:353–61
- Gou Y, Wan TH, Zhang L. (2006). overview research of pharmacokinetics of puerarin. Lishizhen Medicine and Materia Medica Research 17:1083–4
- Kalyanasundaram K, Thomas JK. (1977). Environmental effects on vibronic band intensities in pyrene monomer fluorescence and their application in studies of micellar systems. J Am Chem Soc 99:2039–44
- Katrin MG, Shlomo M. (2012). Organic nanoparticles from microemulsions: Formation and applications. Curr Opin Colloid In 17:290–6
- Liu X, Xiang DX. (2007). Preparation and evaluation of puerarin microemulsion for oral delivery. Cent South Pharm 5:451–5
- Luo CF, Yuan M, Chen MS, et al. (2010). Metabolites of puerarin identified by liquid chromatography tandem mass spectrometry: Similar metabolic profiles in liver and intestine of rats. J Chromatogr B 878:363–70
- Marttin E, Verhoef JC, Spies F, et al. (1999). The effect of methylated-cyclodextrins on the tight junctions of the rat nasal respiratory epithelium: electron microscopic and confocal laser scanning microscopic visualization studies. J Control Rel 57: 205–13
- Nair AB, Kaushik A, Attimarad M, Al-Dhubiab BE. (2012). Enhanced oral bioavailability of calcium using bovine serum albumin microspheres. Drug Deliv 19:277–85
- Pakhomov AG, Bowman AM, Ibey BL, et al. (2010). Lipid nanopores can form a stable, ion channel-like conduction pathway in cell membrane. Int J Pharm 385:181–6
- Ren F, Jing Q, Shen Y, et al. (2006). Quantitative determination of puerarin in dog plasma by HPLC and study on the relative bioavailability of sustained release tablets. J Pharm Biomed Anal 41:549–53
- Sarmento B, Ribeiro A, Veiga F, et al. (2007). Oral bioavailability of insulin contained in polysaccharide nanoparticles. Biomacromolecules 8:3054–60
- Shao GQ, Shen Q, Mao HZ, et al. (2001). Phase Structures of Microemulsions Determined by the Steady-State Fluorescence Method. Chinese Chem Lett 12:1109–12
- Wei J, He HL, Zheng CL, Zhu JB. (2011). Chitosan-coated ophthalmic submicro emulsion for pilocarpine nitrate. Yao Xue Xue Bao 46:990–6
- Yeung DKY, Leung SWS, Xu YC. (2006). Puerarin, an isoflavonoid derived from Radix puerariae, potentiates endothelium-independent relaxation via the cyclic AMP pathway in porcine coronary artery. Eur J Pharmacol 552:105–11
- Yin YM, Cui FD, Mu CF, et al. (2009). Docetaxel microemulsion for enhanced oral bioavailability: Preparation and in vitro and in vivo evaluation. J Control Release 140:86–94