Abstract
The pH-sensitive liposomes have been extensively used as an alternative to conventional liposomes in effective intracellular delivery of therapeutics/antigen/DNA/diagnostics to various compartments of the target cell. Such liposomes are destabilized under acidic conditions of the endocytotic pathway as they usually contain pH-sensitive lipid components. Therefore, the encapsulated content is delivered into the intracellular bio-environment through destabilization or its fusion with the endosomal membrane. The therapeutic efficacy of pH-sensitive liposomes enables them as biomaterial with commercial utility especially in cancer treatment. In addition, targeting ligands including antibodies can be anchored on the surface of pH-sensitive liposomes to target specific cell surface receptors/antigen present on tumor cells. These vesicles have also been widely explored for antigen delivery and serve as immunological adjuvant to enhance the immune response to antigens. The present review deals with recent research updates on application of pH-sensitive liposomes in chemotherapy/diagnostics/antigen/gene delivery etc.
Introduction
Liposomes are pharmaceutical carriers of choice for numerous practical applications such as for controlled and targeted delivery of drugs/antigen/DNA and/or diagnostic materials. The surface modified targeted liposomes serve as one of the smartest cargo capable of bypassing barriers imposed by the biological environment even up to cellular and sub-cellular levels (Paliwal et al., Citation2011). The liposomal products like Doxil® and Ambisomes® have reached the market and are being continuously used by clinicians. The development and application of novel targeting and/or release triggering schemes to improve the therapeutic index of drugs encapsulated within liposomes are widely reported in the literature. The composition of liposomal systems can be easily modified to facilitate triggered release in response to environmental conditions. For example, liposomes are specifically designed to control the release of their contents in response to acidic pH of the endosomal system. Such plasma stable liposomes are known as pH-sensitive liposomes (Hong et al., Citation2002). The concept of pH-sensitive liposomes tremendously improves the intracellular delivery of various materials such as anti-tumor drugs, toxins, proteins and DNA (Gerasimov et al., Citation1999; Roux et al., Citation2004). The pH-sensitive liposomes offer the protective effects of other liposomal formulations with specific environment-controlled drug release. Mechanistically, the pH-sensitive liposomes undergo rapid destabilization in acidic environments such as endosomes. They are generally composed of a neutral cone-shaped lipid dioleoylphosphatidyl-ethanolamine (DOPE) and a weakly acidic amphiphile, such as cholesteryl hemisuccinate (CHEMS) (Lai et al., Citation1985). The fusogenic behavior of these liposomes is due to the DOPE present in its lipid layer, which does not form bilayer structure when dispersed in aqueous media but form hexagonal structure. Another lipid such as dioleoylphosphatidylcholine (DOPC) (Webb et al., Citation1995) or N-succinyl-DOPE (Nayar & Schroit, Citation1985) can also be incorporated to induce pH sensitivity. These lipids have negatively charged group, which can be neutralized on acidification in endosome, leading to destabilization, fusion with endosomal membrane and content release (Drummond et al., 2000; Lutwyche et al., Citation1998; Venugopalan et al., 2002).
The pH-sensitive liposomes rapidly get destabilized in the acidic pH environment of the tumor tissue. However, the applications of pH-sensitive liposomes become limited due to their recognition and sequestration by the phagocytes of the reticulo-endothelial system (RES). This leads to a condition of very short circulation half-life of these carriers. To avoid their RES uptake and prolong circulation time, grafting of the liposomal membranes with pegylated phospholipids (PEG-lipids) is recommended (Momekova et al., Citation2010). In addition, the development of nano-size liposomes (about 150 nm or less) can contribute to the increase of the additional circulation time. The present review updates with recent development, mechanism and application of pH-sensitive liposomes as smart delivery vehicle for a variety of therapeutics.
The pH-sensitive liposomes in drug delivery
Liposomes offer numerous advantages as drug delivery systems, such as their size can be easily optimized for crossing the fenestration into interstitial space and efficient cellular uptake via endocytosis/phagocytosis. Furthermore, their surface can be equipped with a hydrophilic polymer, such as with a layer of PEG, to minimize the recognition and subsequent uptake by RES, and thus to have a longer circulation time for passive accumulation in cancer tissues via the enhanced permeation and retention (EPR) effect (Andersen et al., 2005; Yu et al., Citation2010). The EPR effect is schematically presented in . Further, the availability of surface for easy modification using site-directing ligand provides opportunity for targeted liposomal drug delivery. The idea of pH-sensitive drug delivery was introduced in 1980 (Yatvin et al., 1980a,b). Later on, it was found that altered pH in different locations such as tumor extracellular environments is advantageous in the design of pH-responsive liposomes for specific cancer cell targeting, enhanced cellular internalization and rapid intracellular drug release (Bertrand et al., Citation2010; Sawant et al., Citation2006). depicts the mechanism of drug delivery via pH-sensitive liposomes. The intracellular delivery of cytotoxic drugs by the pH-sensitive liposomes presents an efficient means of overcoming the multidrug resistance, one of the causes for cancer treatment failures. Further, the anchoring of site-directing ligands on such pH-sensitive liposomes potentiates the effect by targeted drug delivery (Simard & Leroux, Citation2009, Citation2010).
Figure 1. Selective uptake of liposomes by enhanced permeation and retention (EPR) effect. The disrupted endothelial lining allows entry of liposomes to tumor tissues and absence of lymphatic drainage support the retention of drugs in the tumor area hence called enhanced permeation and retention effect (EPR).
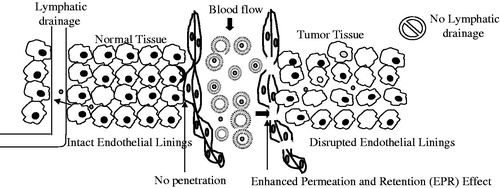
Figure 2. Targeted drug delivery from pH-sensitive liposome. (A) Mechanism involved in intracellular drug delivery; [1] Destabilization of pH-sensitive liposomes triggers the pore formation in endosomal membrane, [2] Destabilization of liposomes release the encapsulated molecules in endosome which diffuse to the cytoplasm through the endosomal membrane, [3] Fusion between the liposome and the endosomal membranes. (B) Localized delivery of anticancer drug to tumor site.
![Figure 2. Targeted drug delivery from pH-sensitive liposome. (A) Mechanism involved in intracellular drug delivery; [1] Destabilization of pH-sensitive liposomes triggers the pore formation in endosomal membrane, [2] Destabilization of liposomes release the encapsulated molecules in endosome which diffuse to the cytoplasm through the endosomal membrane, [3] Fusion between the liposome and the endosomal membranes. (B) Localized delivery of anticancer drug to tumor site.](/cms/asset/d95c9d76-cfcd-439e-95e7-edb422ffe7a8/idrd_a_882469_f0002_b.jpg)
The intracellular delivery of biologically active molecules using liposomes has been the subject of intensive research (Simoes et al., Citation2001, Citation2004). The pH-sensitive liposomes are able to interact and promote fusion or destabilization of endosomal membrane and efficiently release the encapsulated contents into the cell cytosol. The concept of pH-sensitive liposomes emerged from the fact that certain enveloped viruses developed strategies to take advantage of the acidification of the endosomal lumen to infect cells, as well as from the observation that some pathological tissues i.e. tumors, inflamed and infected areas, exhibit an acidic environment as compared to normal tissues. One of the mechanism triggering pH-sensitivity in liposomes is the combination of phosphatidylethanolamine (PE) or its derivatives with compounds containing an acidic group (e.g. carboxylic group) that act as a stabilizer at neutral pH. The pH-sensitive liposomes have been used to deliver anticancer drugs, antisense oligonucleotides, ribozymes, plasmids, proteins and peptides to cells in culture or in vivo (Slepushkin et al., Citation1997). Some of the recent reports are summarized in .
Table 1. List of recently published reports on pH-sensitive liposomes.
Fattal et al. (Citation2004) reported pH-sensitive liposomes for the “smart” delivery of antisense oligonucleotides. These are molecules that are able to inhibit gene expression, and potentially active for the treatment of viral infections cancer or inflammatory diseases. However, because of their poor stability and poor intracellular penetration, “smart” delivery systems such as anionic pH-sensitive liposomes are required. The liposomes composed of PE released their contents in response to acidic pH within the endosomal system while remaining stable in plasma, thus improving the cytoplasmic delivery of oligonucleotides after endocytosis (Fattal et al., Citation2004).
Chen et al. (Citation2011) evaluated a novel modified pH-sensitive liposome with a cleavable double smart PEG-lipid derivative (mPEG-Hz-CHEMS) to clarify the mechanism. The mPEG-Hz-CHEMS is cleaved to pH-responsive hydrazone and esterase-catalyzed at ester linkages so that the PEGylated coating can be efficiently removed by the lower pH and esterase in the serum. This reduces or eliminates the accelerated blood clearance (ABC) phenomenon induced by repeated injection of PEGylated liposomes and hence pH-sensitive stealth liposomes have been proposed as improved carrier (Chen et al., Citation2011).
The pH-sensitive component
To understand the pH-sensitive behavior of liposomes, a study of the physiochemical aspects of pH-sensitive component is important. The phosphatidylethanolamine (PE), commonly used component in liposome, presents a minimally hydrated and small head group as compared to the respective hydrocarbon chains and exhibits a cone shape in spite of a lamellar phase (Seddon et al., Citation1983). The cone shape favors the formation of inverted hexagonal phase above the phase transition temperature due to interaction of amine and phosphate groups of the polar head group. When we intercalate amphiphilic molecules containing a protonatable acidic group in PE, it will form a bilayer structure stable at physiological pH (Lai et al., Citation1985). Although stable liposomes are formed at physiological pH, on acidification, protonation of the acidic groups of the amphiphiles leads to the destabilization of liposomes (Torchilin et al., Citation1993). Hence, selection of the pH-sensitive lipid and amphiphilic stabilizers is desired for the formation of stable pH-sensitive liposomes and determine the properties such as extent of cellular internalization, the fusogenic ability, pH sensitivity and stability in biological fluids. The dioleylphosphatidylamine (DOPE) is one of the key ingredients of pH-sensitive liposomes. The liposomes containing DOPE with phosphatidylglycerol (PG), phosphatidylserine (PS), phosphatidylcholine (PC) and cholesteryl hemisuccinate (CHEMS), when incubated in acidic pH of endosomes, such liposomes undergo destabilization due to the presence of DOPE. This effect is mediated by low hydration of the polar head group of DOPE which is converted to a hexagonal inverted phase leading to the formation of non-lamellar structures and trigger destabilization at acidic pH (Litzinger & Huang, Citation1992). lists some of the lipid/polymer components of pH-sensitive liposomes.
Table 2. Lipids or polymer used for the preparation of pH-sensitive liposomes.
Novel pH-sensitive lipids
Some novel types of pH-sensitive lipids have been introduced for the formation of pH-sensitive liposomes. A liposome formulation consisting of egg PC and succinylated poly (glycidol), a PEG derivative having carboxyl groups is reported in literature. Although succinylated poly(glycidol) is not a pH-sensitive lipid itself; however a combination of this lipid with PC molecules results in the formation of a complex with geometry similar to that of DOPE. This phenomenon occurs due to the formation of hydrogen bonds between its hydroxyl group and an oxygen atom of the phosphate group of PC and hence under acidic conditions develops fusion ability with the endosomal membrane (Kono et al., Citation1994). Three different techniques have been used for the preparation of pH-sensitive liposomes in the absence of DOPE. First, the combination of cationic/anionic lipid is used for efficient vehicle for cytosolic delivery. The pH-sensitive liposomes containing EPC, dimethyldioctadecylammonium bromide (DDAB), CHEMS and Tween-80 undergo destabilization under acidic pH and showed retention of pH sensitivity in the presence of serum (Shi et al., Citation2002). Second, a lipid diolein with CHEMS in the ratio 6:4 have been reported for the preparation of anionic pH-sensitive liposomes. These liposomes were found to be stable at physiological pH, but rapidly released encapsulated content at pH 5.0 (Guo et al., Citation2002). The third formulation containing egg phosphatidylcholine (PC), cholesteryl hemisuccinate (CHEMS), oleyl alcohol (OAlc) and Tween-80 (T-80) as ingredients has been reported for its pH-sensitive characteristics in order to provide option to first generation pH-sensitive liposomes, based on the cone-shaped lipid dioleoylphosphatidylethanolamine (DOPE) (Sudimack et al., Citation2002). Results indicated that in comparison to DOPE-based pH-sensitive liposomes, the above formulations showed much better retention of their pH-sensitive properties in the presence of 10% serum. Additionally, such a system, when appended with ligands for folate receptor (FR) of tumor cells, showed approximately 17-times greater FR-dependent cytotoxicity in KB cells compared to FR-targeted non-pH-sensitive liposomes.
pH-sensitive polymers
The alkylated N isopropylacrylamide (NIPAM) co-polymer has been reported to confer pH sensitivity on liposomes. Incorporation of NIPAM to egg PC and cholesterol liposomes resulted in the formation of pH-sensitive liposomes that released the encapsulated moieties on reaching to the acidic environment (Leroux et al., Citation2001; Roux et al., Citation2004). Roux et al. (Citation2002b) reported randomly alkylated NIPAM-anchored liposomes of ara-c. The developed liposomes showed a higher cytotoxicity towards J774 macrophage cell in comparison to liposomes without NIPAM. Various NIPAM-based copolymers have been synthesized and evaluated for the pH sensitivity, serum stability (Roux et al., Citation2002a, Citationb, Citation2003) and stearic stabilization in sequential studies by a research group (Roux et al., Citation2002b, Citation2003).
Uptake and intracellular delivery of bioactive by pH-sensitive liposomes
The pH-sensitive liposomes are internalized more efficiently as phosphatidylehtanolamine (PE)-containing liposomes have the tendency of form aggregates, due to the poor hydration of its head group which can explain their high affinity to adhere to cell membranes (Seddon et al., Citation1983). The cone shape of PE molecules favors the establishment of strong intermolecular interactions between the amine and phosphate groups of the polar head groups, justifying the strong tendency of these molecules to acquire the inverted hexagonal phase above the phase transition temperature. While at physiological pH stable liposomes are formed, acidification triggers protonation of the carboxylic groups of the amphiphiles, reducing their stabilizing effect and thus leading to liposomal destabilization, since under these conditions PE molecules revert into their inverted hexagonal phase (Torchilin et al., Citation1993).
In case of ligand anchored pH-sensitive liposomes, the different steps are involved in the internalization and intracellular delivery (although numerous other approaches are also used for this purpose). After ligand binding to cell receptors, ligand anchored liposomes are internalized through the endocytotic pathway. The internalized liposomes will be retained in early endosomes, which mature into late endosomes. The fusogenic potential of pH-sensitive liposomes enables them for rapid destabilization at this stage, which prevents degradation of drug at the lysosomal level leading to their higher assessment to the cytosolic or nuclear targets. Interestingly, intracellular drug delivery via pH-sensitive liposomes is dependent on the pH drop in the endosome upon maturation to late endosome. This can be evaluated using lysosomotropic agents, which prevent endosome acidification. Overall, three mechanisms have been proposed for intracellular release of the drug from pH-sensitive liposomes. First, the destabilization of pH-sensitive liposomes triggers the destabilization of the endosomal membrane, most likely through pore formation, leading to cytoplasmic delivery of their contents (Collins, Citation1995). Second, upon liposome destabilization, the encapsulated molecules diffuse to the cytoplasm through the endosomal membrane. Third, the fusion between the liposome and the endosomal membranes leads to cytoplasmic delivery of their contents. The fusogenic properties of PE favor the hypotheses first and third.
Application of pH-sensitive liposomes
lists the patents in the area of pH-sensitive liposomes. The various applications of these carrier systems are described in different sections as follows:
Table 3. List of patents on pH-sensitive liposomes.
The pH-sensitive liposomes in chemotherapy
The pH-sensitive liposomes have been prepared for numerous applications in drug delivery including chemotherapy (Karanth & Murthy, Citation2007). In a study, engineered drug carriers capable of spontaneous accumulation in tumor areas via the enhanced permeability and retention (EPR) effect have been reported. These liposomes were simultaneously bearing on their surface cell penetrating peptide such as TAT (transactivator of transcription) peptide (TATp) moieties and protective PEG chains. PEGylated liposomes are expected to accumulate in targets via the EPR effect, but inside the “acidified” tumor ischemic tissues lose their PEG coating due to the lowered pH-induced hydrolysis and penetrate inside cells via the now-exposed TATp moieties (Kale & Torchilin, Citation2007). Cisplatin is one of the most active cytotoxic agents and has been widely used in the treatment of carcinomas. A biodistribution study of stealth pH-sensitive liposomes containing cisplatin and free cisplatin, in solid Ehrlich tumor-bearing mice has been reported (Junior et al., Citation2007). After administering a 6 mg/kg single intravenous bolus injection of either free radiolabeled cisplatin or stealth pH sensitive liposomes containing radiolabeled cisplatin, blood and tissues were analyzed. The area under the concentration-time curve (AUC) obtained for blood using stealth pH sensitive liposomes administration was found to be 2.1 fold larger when compared with free cisplatin treatment (Araujo et al., Citation2011).
A similar report is also published very recently describing the importance of pH-sensitive liposomes for improved biodistribution and targeted delivery of cisplatin to tumor-bearing mice. Though long-circulating and pH-sensitive liposomes, containing cisplatin successfully avoid severe side effects as well as drug resistance and have been proven effective too. However, physical (i.e. aggregation/fusion) and chemical instabilities limit the use of these drug carriers as pharmaceutical products. Therefore, it is suggested that freeze-dried liposomes may be a successful strategy implemented to improve the stability of these formulations (dos Santos et al., Citation2011). Koren et al. (Citation2012) reported pH-sensitive pegylated long-circulating liposomes modified with cell-penetrating TAT peptide (TATp) moieties and cancer-specific mAb. A degradable pH-sensitive hydrazone bond between a long shielding PEG chains and PE was introduced. The approach was using TATp conjugation with a short PEG1000-PE spacer and mAb to a long PEG chain (PEG3400-PE). The “shielding” effect of TATp by long PEG chains was the aim which was further investigated using three liposomal models. At normal pH, surface TATp moieties are “hidden” by the long PEG chains. Upon the exposure to lowered pH, this multifunctional carrier exposes TATp moieties after the degradation of the hydrazone bond and removal of the long PEG chains. Enhanced cellular uptake of the TATp-containing immunoliposomes loaded with doxorubicin was observed in vitro after pre-treatment at lowered pH. The presence of mAb 2C5 on the liposome surface further enhanced the interaction between the carrier and tumor cells but not normal cells. Furthermore, this multifunctional immuno-Doxil® preparation showed increased cellular cytotoxicity of B16-F10, HeLa and MCF-7 cells when pre-incubated at lower pH, indicating TATp exposure and activity. A multifunctional immunoliposomal nanocarrier was claimed for intracellular drug delivery after exposure to lowered pH environment, typical of solid tumors (Koren et al., Citation2012).
Jiang et al. (Citation2012) designed dual-functional liposomes with pH-responsive cell-penetrating peptide and targeting ligand hyaluronic acid for tumor therapy. Paclitaxel-loaded hyaluronic acid (HA)-anchored pH-sensitive liposomes possess a remarkably stronger cytotoxicity toward the hepatic cancer (HepG2) cells at pH 6.4 as compared to at pH 7.4. The confocal laser scanning microscopy of coumarin 6-loaded HA-anchored liposomes showed efficient intracellular trafficking including endosomal/lysosomal escape and cytoplasmic release (Jiang et al., Citation2012). The engineered estrogen receptor (ER) targeted pH-sensitive liposomes for the site-specific intracellular delivery of doxorubicin (DOX) for breast cancer therapy have been developed by our group. Estrone, a bioligand, was anchored on the surface of pH-sensitive liposome for drug targeting to ERs over-expressed by breast cancer cells. The estrone-anchored pH-sensitive liposomes (ES-pH-sensitive-SL) showed fusogenic potential at acidic pH (5.5). In vitro cytotoxicity studies, which were performed on MCF-7 cells, revealed that ES-pH-sensitive-SL formulation was more cytotoxic than non-pH-sensitive targeted liposomes (ES-SL). Further, in vivo biodistribution studies and antitumor activities of formulations were conducted on tumor bearing female Balb/c mice followed by intravenous administration. The ES-pH-sensitive-SL efficiently suppressed the breast tumor growth in comparison to both ES-SL and free DOX. The ES-pH-sensitive-SL accelerated the intracellular trafficking of encapsulated DOX, thus increasing the therapeutic efficacy. This suggested that estrone-anchored pH-sensitive liposomes may serve as one of the promising drug delivery carriers for the targeted intracellular delivery of anticancer agents to breast cancer with reduced systemic side effects (Paliwal et al., Citation2012). The PEG-coated pH-sensitive and PEG-folate-coated pH-sensitive liposomes containing the Gd-DTPA-BMA and radiolabeled have been reported to study the in vivo anti-tumoral activity on mice bearing solid Ehrlich tumor by Soares et al., Citation2012. The study revealed that after 31 days of treatment, mice treated with formulations had a lesser increase in tumor volume and a significantly higher percentage of necrosis compared with controls. Furthermore, mice treated with radioactive formulations exhibited lower weight gain without significant hematological or biochemical changes, except for toxicity to hepatocytes (Soares et al., Citation2012).
Cationic liposomes facilitate the cellular uptake by electrostatic absorptive endocytosis due to negative charge on the cell membrane (Bareford et al., Citation2007). These liposomes after internalization fuse with the endosomal membrane to release their contents into the cytoplasm (Koynova et al., Citation2006) or exhibit the proton sponge effect leading to swelling and subsequent disruption of endosomal membrane resulting into cytoplasmic delivery of intact liposomes (Shigeta et al., Citation2007). Although these liposomes posses capability of intracellular delivery, they are associated with the limitations such as cytotoxicity and rapid clearance from RES (Soenen et al., Citation2009). The incorporation of poy(ethylene glycol) modified lipid (PEG-lipid) into the membrane of cationic liposomes increase half life of liposomes to some extent, however, it impaired the interaction between liposomes and cell membrane of tumor cell (Moore et al., Citation2008). To circumvent this pH-sensitive hydrazone bond is incorporated between PEG and lipid that can be degrade in the tumor bioenvironment and shedding PEG for better interaction of carrier and cell membrane (Rodrigues et al., Citation1999). However, little success is achieved as the linkage cannot be broken in the extracellular environment (pH 6.0–7.0) but generally in the endosomal compartment (pH 4.0–6.0).
To exploit the merits of anionic liposomes (lower hematotoxicity), pegylated liposomes (longer circulation time), and cationic liposomes (enhanced uptake) Mo et al. (Citation2013) developed pH-sensitive zwitterionic oligopeptide liposomes for intracellular delivery of temsirolimus (CCI-779). They developed and evaluated pH-sensitive liposomes based on zwitterionic oligopeptide lipids (HHG2C(18)-L and PEGHG2C(18)-L) as anticancer drug carriers for intracellular delivery and enhanced antitumor activity. The (HHG2C(18)-L; 1,5-dioctadecyl-l-glutamyl 2-histidyl-hexahydrobenzoic acid and PEGHG2C(18)-L); 5-distearyl N-(N-α-(4-mPEG2000) butanedione)-histidyl-l-glutamate are amino based lipid which have multistage pH-response to tumor bioenvironmental pH (pH 6.0–7.0) and endosomal/lysosomal pH (pH 4.0–6.0) successively. To understand localization of these carriers within the cell, coumarin 6-loaded pH-sensitive liposomes with these lipids were also prepared which showed higher tumor cellular uptake due to electrostatic absorptive endocytosis at (pH 6.5). This produced proton sponge effect for endo-lysosomal escape, and accumulated to the mitochondria based on stronger positive charge by the hydrolysis of a pH-sensitive linker at pH 5.5 and pH 4.5 (Mo et al., Citation2013).
The pH-sensitive immunoliposomes
Liposomal drug accumulation in the target tissue can be improved by attachment of the ligand capable of recognizing and binding to receptor present on the cell of interest. Antibody and their fragments are the most widely explored targeting moieties which can be easily anchored to liposomal surface without alteration of liposome integrity and the antibody properties (Torchilin, Citation1985). Immunoliposomes directed by monoclonal antibodies are promising vehicles for tumor targeted drug delivery (Abra et al., Citation2002; Kim et al., Citation2008). To further enhance the efficacy and cytosolic release of encapsulated content pH-sensitive immunoliposomes have been developed which are taken up by the target cell by ligand mediated endocytosis and upon internalization they fuses with the endosomal membrane at low pH.
Several laboratories exploited pH-sensitive liposomes for the delivery of therapeutic macromolecule (Connor et al., Citation1984; Duzgunes et al., Citation1985). The pH-sensitive liposomes containing herpes simplex virus thymidine kinase gene released content into the cytoplasm and delivered DNA expressed efficiently in the cells (Wang & Huang, Citation1986). To improve binding efficiency, target cells can be improved by using immunoliposomes (Huang et al., Citation1982). Wang & Huang (Citation1987) reported immunoliposomes that bind to major histocompatibility antigen H2-Kk for the delivery of model gene Escherichia coli chloramphenicol acetyltransferase (CAT). Conner & Huang (Citation1986) developed pH-sensitive immunoliposomes composed of dioleoylphosphatidylethanolamine and oleic acid containing cytotoxic drug 1-0-D-arabinofuranosylcytosine (ara-C) modified with anti-H-2Kk antibody. It was claimed that these pH-sensitive immunoliposomes efficiently delivered drug to target L-929 cells; however they were ineffective against nontarget A-3I cells (Conner & Huang, Citation1986).
Kim et al. (Citation2009) developed long-circulating pH-sensitive liposomes with epidermal growth factor receptor antibody and were tested on A549 cells and BALB/c-nu/nu mouse tumor model. The developed formulations provided an efficient and targeted delivery of gemcitabine for tumors which over-express the EGFR (Kim et al., Citation2009). The knowledge of internalization pathway plays a fundamental role in optimizing drug targeting.
The pH-sensitive liposomes in tumor diagnosis
Liposomes are excellent candidates for the development of theragonostic agents and multimodal imaging probes. They release the entrapped imaging probe/radioactive agent in response to a change of physico-chemical variables like pH, redox potential, or concentration of specific enzymes that usually occur in the early asymptomatic stage of several diseases such as tumor. The pH-sensitive liposomes have also been utilized for diagnosis of tumor. The pH-sensitive liposomes entrapping 99mTc has been reported for scintigraphic imaging in Ehrlich tumor-bearing mice. The system showed high accumulation in tumor tissue with high tumor-to-muscle ratio and proposed as a potential agent for tumor diagnosis (de Barros et al., Citation2011).
In another report, paramagnetic pH-sensitive liposomes have been developed as imaging tools by magnetic resonance imaging (MRI). The proposed formulation allowed the fast and full release of Gadoteridol at pH 5.5. The leakage of the imaging reporter from the vesicles was associated with a relativity enhancement that allowed its visualization by MRI. It was claimed that the release mechanism implies the protonation of the basic sites that leads to vesicle aggregation, thus enabling the expression of the fusogenic property (Torres et al., Citation2011). Ferreira et al. (Citation2012) developed long-circulating and pH-sensitive liposomes containing a 99mTc-labeled antibiotic, ceftizoxime, SpHL-(99mTc-CF) to identify osteomyelitis, an infectious disease located in the bone or bone marrow. The biodistribution studies showed a high values in the target-non target ratio and indicated that SpHL-99mTc-CF as a potential agent for the diagnosis of bone infections (Ferreira et al., Citation2012).
The pH-sensitive liposomes in vaccine delivery
The pH-sensitive liposomes are being explored as promising vehicles for delivery of therapeutic and prophylactic vaccines (Chang et al., Citation2001; Watabe et al., Citation2001). These liposomes are employed as effective means for delivery of small sized peptides, producing efficient immune response and reducing their toxicity (Tachibana et al., Citation1998). It is well known that efficient vaccination strategies have been required to overcome new upcoming pathogens. Dendritic cells are known as potent professional antigen presenting cells, they play a crucial role in innate and adaptive immune responses. The DCs recognize, take up, process and present antigens to native and resting T cells for induction of an antigen-specific immune response. One of the most effective strategies for efficient introduction of antigenic proteins into cytosol of DC is to use membrane fusion especially for membrane-based liposomes (Yoshikawa et al., Citation2008). Chang et al. (Citation1999) developed V3 peptides encapsulated pH-sensitive liposomes to elicit the humoral and cell specific responses. No immune response was observed with the immunogen without liposomes, while antibodies and CTL response were detected with peptide encapsulated pH-sensitive liposomes. The results of this study conclude that immunogen encapsulated pH-sensitive liposomes may be used as a potentially immunomodulating adjuvant system for the development of HIV and other viral vaccines (Chang et al., Citation1999). In their another report, Chang et al. (Citation2001) delivered cytotoxic T lymphocyte (CTL) epitopes successfully to target cells by encapsulation into pH-sensitive liposomes. They reported that CTL epitopes are transferred to endoplasmic reticulum (RE) to associate with class I major histocompatibility complex (MHC) molecules (Chang et al., Citation2001). Lee et al. (Citation2002) investigated the immunization potential of pH-sensitive liposomes encapsulating fluorescein isothiocyanate (FITC)-conjugated H-2Kb CTL epitope. After 3 days of immunization, pH-sensitive liposomes elicited significant CTL activity against the delivered antigen. Authors suggested that pH-sensitive liposomes served as a strong peptide adjuvant, which may be useful for peptide delivery for the development of therapeutic or prophylactic vaccines (Lee et al., Citation2002).
Sakaguchi et al. (Citation2008b) developed poly(glycidol) derivatives modified pH-sensitive liposomes. These polymer-modified liposomes delivered calcein into cytosol of HeLa cells after internalization via endocytosis and subsequent fusion with membrane of endosome or lysosome (Sakaguchi et al., Citation2008b). In this sequence Yuba et al. (Citation2010) reported pH-sensitive fusogenic liposomes modified with carboxylated poly(glycidol) derivatives, such as polymer-succinylated poly(glycidol) (SucPG) and 3-methylglutarylated poly(glycidol) (MGluPG) that can deliver antigenic proteins ovalbumin (OVA) into the cytosol of DCs. The nasal administration of these liposomes activated the antigen-specific cellular immunity. The results suggested that the administration of the polymer-modified, OVA-loaded liposomes induced stronger cellular immune responses than the OVA-loaded plain liposomes. The MGluPG modified fusogenic liposomes exhibited high ability for CTL activation than SucPG. Thus it was concluded that MGluPG liposomes and their relevant liposomes might be promising antigen carriers for establishment of cancer immunotherapy and mucosal vaccination (Yuba et al., Citation2013). The pH-sensitive fusogenic SucPG modified liposomes as a vaccine carrier for ovalbumin (OVA) have been evaluated. After immunization only OVA-specific IgG1 antibody responses were observed with OVA-containing polymer-unmodified liposomes, whereas immunization with OVA-containing SucPG-modified liposomes leads to the induction of OVA-specific IgG1, IgG2a and IgG3 Ab responses. The results suggested that the pH-sensitive SucPG modified liposomes may serve effectively as an antigen delivery vehicle for inducing Th1 and Th2 immune responses (Watarai et al., Citation2013). Recently, Yuba et al. (Citation2013) developed polymer modified pH-sensitive liposomes for the delivery of OVA with 3-methylglutarylated poly(glycidol) of linear or hyperbranched structure. These polymer-modified liposomes have been efficiently taken up by murine dendritic cells and subcutaneous or nasal administration of liposomes induced OVA-specific cytotoxic T cells much more effectively than the unmodified liposomes. These polymer-modified liposomes have been proposed as promising carriers of antigen for efficient cancer immunotherapy (Yuba et al., Citation2013).
The pH-sensitive liposomes in gene delivery
The increase in our molecular understanding, the number of biotechnological products such as nucleic acids, proteins and peptides are increasing as therapeutics for several clinical situations. However, the in vivo efficacy of these products can be severely compromised by their unfavorable physicochemical characteristics. The major obstacle in their usefulness is large size and hydrophilic nature. Additionally, macromolecules are often delivered to their target located in the cytoplasm; such molecules may not reach their target without a delivery system to overcome cellular barrier and facilitating cytosolic delivery (Leserman et al., Citation1990). Therefore, many different drug delivery systems including liposomes have been explored for this purpose. Liposomes are able to provide protection and targeting of the encapsulated macromolecule and may facilitate cellular internalization (Di Marzio et al., Citation2008; Pedroso de Lima et al., Citation2001). The success of gene therapy required the development of multifunctional carrier that could overcome various barriers, such as the cell membrane, endosomal membrane, and nuclear membrane. Layer-by-layer technique is an efficient method with easy operation which can be used for the assembly of multifunctional gene carriers. A new gene delivery system was evaluated which consists of both the pre-condensed plasmid DNA with an arginine-based cationic surfactant, arginine-N-lauroyl amide dihydrochloride (ALA), and the blood protein transferrin (Tf) into the pH-sensitive liposomes (Rosa et al., Citation2008). They found that the transfection capacity of these systems was higher due to presence of ALA to pH-sensitive liposomes.
The mannosylated pH-sensitive lipoplexes containing cholesterol derivative, Man-His-C4-Chol, which possesses histidine (His) residues was reported for transfection (Nakamura et al., Citation2009). These lipoplexes showed higher cellular uptake suggesting that they are taken up by macrophages via mannose receptor-mediated endocytosis. The transfection efficiency was also higher in case of pH-sensitive derivative as compared to normal one. Khalil et al. (Citation2011) reported a multifunctional envelope-type nanocarrier modified with octaarginine peptide (R8) and glutamic acid-alanine-leucine-alanine (GALA), as a pH-sensitive fusogenic peptide (R8-GALA-MEND) for liver gene delivery. The modification leads to higher gene expression efficiencies per nucleus-delivered pDNA. It was concluded that combination of a negatively charged core system and GALA modification of the R8-MEND could be efficiently used for delivering genes to the liver (Khalil et al., Citation2011). Macrophages express mannose receptors that can be exploited for targeted gene delivery to macrophages for the treatment of various immune diseases. Ducat et al., Citation2011 reported pH-sensitive stealth liposomes as a suitable vector for a therapeutic peptide (Print 3G, a peptidic agent that could reduce the angiogenic development of breast tumors) to its nuclear site of action (Ducat et al., Citation2011). They investigated cellular uptake of peptide-loaded liposomes in three cell lines; Hs578t human epithelial cells from breast carcinoma, MDA-MB-231 human breast carcinoma cells and WI-26 human diploid lung fibroblast cells. The difference between formulations in terms of peptide delivery from the endosome to the cytoplasm and even to the nucleus was observed as function of time. Using pH-sensitive stealth liposomes, the peptide was able to reach the nucleus of tumorigenic and non-tumorigenic breast cancer cells (Ducat et al., Citation2011). These reports clearly demonstrate utility of pH sensitive liposomes as intracellular carrier for bioactive(s).
A pH-sensitive multifunctional gene carrying delivery system has been proposed that offered long circulation time but avoid the inhibition of tumor cellular uptake of carriers associated with the use of polyethylene glycol (Li et al., Citation2012). Deoxyribonucleic acid (DNA) was firstly condensed with protamine into a cationic core which was used as assembly template. Then, additional layers of anionic DNA, cationic liposomes, and o-carboxymethyl-chitosan (CMCS) were alternately adsorbed onto the template via layer-by-layer technique and finally the multifunctional vector called CMCS-cationic liposome-coated DNA/protamine/DNA complexes was constructed. For in vitro test, the cytotoxicity and transfection investigation was carried out on HepG2 cell line and in vivo evaluation into tumor-bearing mice. The isolated tumor cells confirmed transfection efficiency by fluorescence determination (Li et al., Citation2012). CMCS-cationic liposome-coated DNA/protamine/DNA complexes had ellipsoidal shapes and showed “core-shell” structure which showed stabilization property in serum and effective protection of DNA from nuclease degradation. Similar other reports also describe importance of pH sensitivity within liposomal membrane for intracellular gene transfection (Sakaguchi et al., Citation2008a,Citationb,Citationc).
Chen et al. (Citation2012) developed pH-sensitive lipoplexes for efficient gene delivery with the three novel cationic lipids containing a linear ortho ester linker that conjugates either the head group (Type I) or one hydrocarbon chain (Type II) with the rest of the lipid molecule. The cationic lipids bear counter ion either an iodide or a chloride. These lipids contain a trimethyl ammonium head group, two unsaturated oleyl hydrocarbon chains and a linear ortho ester linker. The Type I lipoplexes aggregated and destabilized at the endosomal pH (pH 5.5), but the Type II lipoplexes were not. The Type I ortho ester-based lipoplexes containing helper lipids, DOPE or cholesterol and with the chloride counter ion, significantly improved the gene transfection efficiency, more than 100 fold in CV-1 cells (monkey kidney fibroblast) (Chen et al., Citation2012).
Conclusion
The pH-sensitive liposomes have been explored as therapeutic carrier in different areas of drug delivery including imaging. The unique characteristics of these nanocarriers enable them as one of the preferred choices for formulation development in cancer therapy. The efficient intracellular delivery of bioactive(s) is highly desired to overcome multidrug resistance in case of chemotherapy, which can be achieved by the use of pH-sensitive liposomes. Additionally, components used for designing pH-sensitive liposomes do not impose any regulatory restrictions as they possess no toxicity when used in vivo. Since pH-sensitive liposomes are the modified version of existing and well accepted liposomes hence after proven pre-clinical and clinical effectiveness their commercial viability has prominent chances.
Declaration of interest
The authors report no conflicts of interest. The authors alone are responsible for the content and writing of this article.
References
- Abra RM, Bankert RB, Chen F, et al. (2002). The next generation of liposome delivery systems: recent experience with tumor-targeted, sterically stabilized immunoliposomes and active loading gradients. J Liposome Res 12:1–3
- Andresen TL, Jensen SS, Jorgensen K. (2005). Advanced strategies in liposomal cancer therapy: problems and prospects of active and tumor specific drug release. Prog Lipid Res 44:68–97
- Andrews CD, Huh MS, Patton K, et al. (2012). Encapsulating immunostimulatory CpG oligonucleotides in listeriolysin O-liposomes promotes a Th1-type response and CTL activity. Mol Pharm 9:1118–25
- Araujo JG, Mota Ld, Leite EA, et al. (2011). Biodistribution and antitumoral effect of long-circulating and pH-sensitive liposomal cisplatin administered in Ehrlich tumor-bearing mice. Exp Biol Med (Maywood) 236:808–15
- Bareford LM, Swaan PW. (2007). Endocytic mechanisms for targeted drug delivery. Adv Drug Deliv Rev 59:748–58
- Bertrand N, Simard P, Leroux JC. (2010). Serum-stable, long-circulating, pH-sensitive PEGylated liposomes. Methods Mol Biol 605:545–58
- Chang JS, Choi MJ, Cheong HS, Kim K. (2001). Development of Th1-mediated CD8_ effector T cells by vaccination with epitope peptides encapsulated in pH-sensitive liposomes. Vaccine 19:3608–14
- Chang JS, Choi MJ, Kim TY, et al. (1999). Immunogenicity of synthetic HIV-1 V3 loop peptides by MPL adjuvanted pH-sensitive liposomes. Vaccine 17:1540–8
- Chen D, Liu W, Shen Y, et al. (2011). Effects of a novel pH-sensitive liposome with cleavable esterase-catalyzed and pH-responsive double smart mPEG lipid derivative on ABC phenomenon. Int J Nanomed 6:2053–61
- Chen H, Zhang H, Thor D, et al. (2012). Novel pH-sensitive cationic lipids with linear ortho ester linkers for gene delivery. Eur J Med Chem 52:159–72
- Chen Y, Sun J, Lu Y, et al. (2013). Complexes containing cationic and anionic pH-sensitive liposomes: comparative study of factors influencing plasmid DNA gene delivery to tumors. Int J Nanomed 8:1573–93
- Collins D. (1995). pH-sensitive liposomes as tools for cytoplasmic delivery. In: Philippot JR, Schuber F, eds. Liposomes as tools in basic research and industry. Boca Raton: CRS Press, 201–14
- Conner J, Huang L. (1986). pH-sensitive Immunoliposomes as an efficient and target-specific carrier for antitumor drugs. Cancer Res 46:3431–5
- Connor J, Yatvin MB, Huang, L. (1984). pH-sensitive liposomes: acid-induced liposome fusion. Proc Natl Acad Sci USA 81:1715–18
- de Barros ALB, Mota LDG, Soares DCF, et al. (2011). Tumor bombesin analog loaded long-circulating and pH-sensitive liposomes as tool for tumor identification. Bioorg Med Chem Lett 21:7373–5
- Di Marzio L, Marianecci C, Cinque B, et al. (2008). pH-sensitive non-phospholipid vesicle and macrophage-like cells: binding, uptake and endocytotic pathway. Biochim Biophys Acta 1778:2749–56
- dos Santos Giuberti C, de Oliveira Reis EC, Ribeiro Rocha TG, et al. (2011). Study of the pilot production process of long-circulating and pH-sensitive liposomes containing cisplatin. J Liposome Res 21:60–9
- Drummond DC, Zignani M, Leroux J. (2000). Current status of pH-sensitive liposomes in drug delivery. Prog Lipid Res 39:409–60
- Ducat E, Deprez J, Gillet A, et al. (2011). Nuclear delivery of a therapeutic peptide by long circulating pH-sensitive liposomes: benefits over classical vesicles. Int J Pharm 420:319–32
- Düzgüneş N, Straubinger RM, Baldwin PA, et al. (1985). Proton-induced fusion of oleic acid-phosphatidylethanolamine liposomes. Biochemistry 24:3091–8
- Fattal E, Couvreur P, Dubernet C. (2004). Smart delivery of antisense oligonucleotides by anionic pH-sensitive liposomes. Adv Drug Deliv Rev 56:931–46
- Ferreira SM, Domingos GP, Ferreira Ddos S, et al. (2012). Technetium-99m-labeled ceftizoxime loaded long-circulating and pH-sensitiveliposomes used to identify osteomyelitis. Bioorg Med Chem Lett 22:4605–8
- Gerasimov OV, Boomer JA, Qualls MM, Thompson DH. (1999). Cytosolic drug delivery using pH- and light-sensitive liposomes. Adv Drug Deliv Rev 38:317–38
- Guo W, Gosselin MA, Lee RJ. (2002). Characterization of novel diolein-based LPDII vector for gene delivery. J Control Rel 83:121–32
- Hong MS, Lim SJ, Oh YK, Kim CK. (2002). pH-sensitive, serum-stable and long-circulating liposomes as a new drug delivery system. J Pharm Pharmacol 54:51–8
- Huang A, Tsao YS, Kennel SJ, Huang L. (1982). Characterization of antibody covalently coupled to liposomes. Biochim Biophys Acta 716:140–50
- Jiang T, Zhang Z, Zhang Y, et al. (2012). Dual functional liposomes based on pH-responsive cell-penetrating peptide and hyaluronic acid for tumor-targeted anticancer drug delivery. Biomaterials 33:9246–58
- Junior AD, Mota LG, Nunan EA, et al. (2007). Tissue distribution evaluation of stealth pH-sensitive liposomal cisplatin versus free cisplatin in Ehrlich tumor-bearing mice. Life Sci 80:659–64
- Kale AA, Torchilin VP. (2007). “Smart” drug carriers: PEGylated TATp-modified pH-sensitive liposomes. J Liposome Res 17:197–203
- Karanth H, Murthy RS. (2007). pH-sensitive liposomes–principle and application in cancer therapy. J Pharm Pharmacol 59:469–83
- Khalil IA, Hayashi Y, Mizuno R, Harashima H. (2011). Octaarginine- and pH sensitive fusogenic peptide-modified nanoparticles for livergene delivery. J Control Rel 156:374–80
- Kim IY, Kang YS, Lee DS, et al. (2009). Antitumor activity of EGFR targeted pH-sensitive immunoliposomes encapsulating gemcitabine in A549 xenograft nude mice. J Control Rel 140:55–60
- Kim MJ, Lee HJ, Lee IA, et al. (2008). Preparation of pH-sensitive, long-circulating and EGFR-targeted immunoliposomes. Arch Pharm Res 31:539–46
- Kono K, Zenitani K, Takagishi T. (1994). Novel pH-sensitive liposomes: liposomes bearing a poly(ethylene glycol) derivative with carboxyl groups. Biochim Biophys Acta 1193:1–9
- Koren E, Apte A, Jani A, Torchilin VP. (2012). Multifunctional PEGylated 2C5-immunoliposomes containing pH-sensitive bonds and TAT peptide for enhanced tumor cell internalization and cytotoxicity. J Control Rel 160:264–73
- Koynova R, Wang L, MacDonald RC. (2006). An intracellular lamellarenonlamellar phase transition rationalizes the superior performance of some cationic lipid transfection agents. Proc Natl Acad Sci USA 103:14373–8
- Lai MZ, Duzgunes N, Szoka FC. (1985). Effects of replacement of the hydroxyl group of cholesterol and tocopherol on the thermotropic behavior of phospholipid membranes. Biochemistry 24:1646–53
- Lee KY, Chun E, Seong BL. (2002). Investigation of antigen delivery route in vivo and immune-boosting effects mediated by pH-sensitive liposomes encapsulated with Kb-restricted CTL epitope. Biochem Biophys Res Commun 292:682–8
- Leite EA, Giuberti Cdos S, Wainstein AJ, et al. (2009). Acute toxicity of long-circulating and pH-sensitive liposomes containing cisplatin in mice after intraperitoneal administration. Life Sci 84:641–9
- Leroux J, Roux E, Le Garrec D, et al. (2001). N-Isopropylacrylamide copolymers for the preparation of pH-sensitive liposomes and polymeric micelles. J Control Rel 72:71–84
- Leserman L, Machy P, Leonetti JP, et al. (1990). Targeted liposomes and intracellular delivery of macromolecules. Prog Clin Biol Res 343:95–102
- Li P, Liu D, Miao L, et al. (2012). A pH-sensitive multifunctional gene carrier assembled via layer-by-layer technique for efficient gene delivery. Int J Nanomed 7:925–39
- Litzinger DC, Huang L. (1992). Phosphatidylethanolamine liposomes: drug delivery, gene transfer and immunodiagnostic applications. Biochim Biophys Acta 1113:201–27
- Lutwyche P, Cordeiro C, Wiseman DJ, et al. (1998). Intracellular delivery and antibacterial activity of gentamicin encapsulated in pH-sensitive liposomes. Antimicrob Agents Chemother 42:2511–20
- Mo R, Sun Q, Li N, Zhang C. (2013). Intracellular delivery and antitumor effects of pH-sensitive liposomes based on zwitterionic oligopeptide lipids. Biomaterials 34:2773–86
- Momekova D, Rangelov S, Lambov N. (2010). Long-circulating, pH-sensitive liposomes. Methods Mol Biol 605: 527–44
- Moore NM, Barbour TR, Sakiyama-Elbert SE. (2008). Synthesis and characterization of four-arm poly(ethylene glycol)-based gene delivery vehicles coupled to integrin and DNA-binding peptides. Mol Pharm 5:140–50
- Nakamura K, Kuramoto Y, Mukai H, et al. (2009). Enhanced gene transfection in macrophages by histidine-conjugated mannosylated cationic liposomes. Biol Pharm Bull 32:1628–31
- Nayar R, Schroit AJ. (1985). Generation of pH-sensitive liposomes: use of large unilamellar vesicles containing N-succinyldioleoylphosphatidylethanolamine. Biochemistry 24:5967–71
- Paliwal SR, Paliwal R, Agrawal GP, Vyas SP. (2011). Liposomal nanomedicine for breast cancer therapy. Nanomedicine (London) 6:1085–100
- Paliwal SR, Paliwal R, Pal HC, et al. (2012). Estrogen-anchored pH-sensitive liposomes as nanomodule designed for site-specific delivery of doxorubicin in breast cancer therapy. Mol Pharm 9:176–86
- Paliwal SR, Paliwal R, Vyas SP. (2013). pH-sensitive liposome in drug delivery. In: Alvarez-Lorenzo C, Concheiro A, eds. Smart materials for drug delivery. Vol. 1. UK: Royal Society of Chemistry, 80–93
- Pedroso de Lima MC, Simões S, Pires P, et al. (2001). Cationic lipid-DNA complexes in gene delivery: from biophysics to biological applications. Adv Drug Deliv Rev 47:277–94
- Pichon C, Midoux P. (2013). Mannosylated and histidylated LPR technology for vaccination with tumor antigen mRNA. Methods Mol Biol 969:247–74
- Rodrigues PC, Beyer U, Schumacher P, et al. (1999). Acidsensitive polyethylene glycol conjugates of doxorubicin: preparation, in vitro efficacy and intracellular distribution. Bioorg Med Chem 7:2517–24
- Rosa M, Penacho N, Simöes S, et al. (2008). DNA pre-condensation with an amino acid-based cationic amphiphile. A viable approach for liposome-based gene delivery. Mol Membr Biol 25:23–34
- Roux E, Francis M, Winnik FM, Leroux JC. (2002a). Polymer based pH-sensitive carriers as a means to improve the cytoplasmic delivery of drugs. Int J Pharm 242:25–36
- Roux E, Lafleur M, Lataste E, et al. (2003). On the characterization of pH-sensitive liposome/polymer complexes. Biomacromolecules 4:240–8
- Roux E, Passirani C, Scheffold S, et al. (2004). Serum-stable and long-circulating, PEGylated, pHsensitive liposomes. J Control Rel 94:447–51
- Roux E, Stomp R, Giasson S, et al. (2002b). Steric stabilization of liposomes by pH-responsive N-isopropylacrylamide copolymer. J Pharm Sci 91:1795–802
- Sakaguchi N, Kojima C, Harada A, et al. (2008a). Effect of transferrin as a ligand of pH-sensitive fusogenic liposome-lipoplex hybrid complexes. Bioconjug Chem 19:1588–95
- Sakaguchi N, Kojima C, Harada A, et al. (2008b). The correlation between fusion capability and transfection activity in hybrid complexes of lipoplexes and pH-sensitive liposomes. Biomaterials 29:4029–36
- Sakaguchi N, Kojima C, Harada A, Kono K. (2008c). Preparation of pH-sensitive poly(glycidol) derivatives with varying hydrophobicities: their ability to sensitize stable liposomes to pH. Bioconjug Chem 19:1040–8
- Sawant RM, Hurley JP, Salmaso S, et al. (2006). “SMART” drug delivery systems: double-targeted pH-responsive pharmaceutical nanocarriers. Bioconjug Chem 17:943–9
- Seddon JM, Cevc G, Marsh D. (1983). Calorimetric studies of the gel-fluid (L beta–L alpha) and lamellar-inverted hexagonal (L alpha-HII) phase transitions in dialkyl- and dia- cylophosphatidylethanolamines. Biochemistry 22:1280–9
- Shi G, Guo W, Stephenson SM, Lee RJ. (2002). Efficient intracellular drug and gene delivery using folate receptor-targeted pH-sensitive liposomes composed of cationic/anionic lipid combinations. J Control Rel 80:309–19
- Shigeta K, Kawakami S, Higuchi Y, et al. (2007). Novel histidine-conjugated galactosylated cationic liposomes for efficient hepatocyte-selective gene transfer in human hepatoma HepG2 cells. J Control Rel 118:262–70
- Simard P, Leroux JC. (2009). pH-sensitive immunoliposomes specific to the CD33 cell surface antigen of leukemic cells. Int J Pharm 381:86–96
- Simard P, Leroux JC. (2010). In vivo evaluation of pH-sensitive polymer-based immunoliposomes targeting the CD33 antigen. Mol Pharm 7:1098–107
- Simoes S, Moreira JN, Fonseca C, et al. (2004). On the formulation of pH-sensitive liposomes with long circulation times. Adv Drug Del Rev 56:947–65
- Simoes S, Slepushkin V, Duzgunes N, et al. (2001). On the mechanisms of internalization and intracellular delivery mediated by pH-sensitive liposomes. Biochim Biophys Acta 1515:23–37
- Slepushkin VA, Simoes S, Dazin P, et al. (1997). Sterically stabilized pH-sensitive liposomes. Intracellular delivery of aqueous contents and prolonged circulation in vivo. J Biol Chem 272:2382–8
- Soares DC, Cardoso VN, de Barros AL, et al. (2012). Antitumoral activity and toxicity of PEG-coated and PEG-folate-coated pH-sensitive liposomes containing 159Gd-DTPA-BMA in Ehrlich tumor bearing mice. Eur J Pharm Sci 45:58–64
- Soenen SJ, Brisson AR, De Cuyper M. (2009). Addressing the problem of cationic lipid-mediated toxicity: the magnetoliposome model. Biomaterials 30:3691–701
- Subbarao NK, Parente RA, Szoka FC, et al. (1987). pH-dependent bilayer destabilization by an amphipathic peptide. Biochemistry 26:2964–72
- Sudimack JJ, Guo W, Tjarks W, Lee RJ. (2002). A novel pH sensitive liposome formulation containing oleyl alcohol. Biochim Biophys Acta 1564:31–7
- Tachibana R, Harashima H, Shono M, et al. (1998). Intracellular regulation of macromolecules using pH-sensitive liposomes and nuclear localization signal: qualitative and quantitative evaluation of intracellular trafficking. Biochem Biophys Res Commun 251:538–44
- Torchilin VP, Zhou F, Huang L. (1993). pH-sensitive liposomes. J Liposome Res 3:201–55
- Torchilin VP. (1985). Liposomes as targetable drug carriers. CRC Crit Rev Ther Drug Carrier Syst 1:65–115
- Torres E, Mainini F, Napolitano R, et al. (2011). Improved paramagnetic liposomes for MRI visualization of pH triggered release. J Control Rel 154:196–202
- Venugopalan P, Jain S, Sankar S, et al. (2002). pH-sensitive liposomes: mechanism of triggered release to drug and gene delivery prospects. Pharmazie 57:659–71
- Wang CY, Huang L. (1986). pH-sensitive immunoliposomes mediate target-cell-specific delivery and controlled expression of a foreign gene in mouse. Proc Natl Acad Sci USA 84:7851–5
- Wang CY, Huang L. (1987). pH-sensitive immunoliposomes mediate target-cell-specific delivery and controlled expression of a foreign gene in mouse. Proc Natl Acad Sci USA 84:7851–5
- Watabe S, Xin KQ, Ihata A, et al. (2001). Protection against influenza virus challenge by topical application of influenza DNA vaccine. Vaccine 19:4434–44
- Watarai S, Iwase T, Tajima T, et al. (2013). Efficiency of pH-sensitive fusogenic polymer-modified liposomes as a vaccine carrier. Sci World J 2013:1–7
- Webb MS, Wheeler JJ, Bally MB, Mayer LD. (1995). The cationic lipid stearylamine reduces the permeability of the cationic drugs verapamil and prochlorperazine to lipid bilayers: implications for drug delivery. Biochim Biophys Acta 1238:147–55
- Woodle MC, Matthay KK, Newman MS, et al. (1992). Versatility in lipid compositions showing prolonged circulation with sterically stabilized liposomes. Biochim Biophys Acta 1105:193–200
- Xia G, An Z, Wang Y, et al. (2013). Synthesis of a novel polymeric material folate-poly(2-ethyl-2-oxazoline)-distearoyl phosphatidyl ethanolamine tri-block polymer for dual receptor and pH-sensitive targeting liposome. Chem Pharm Bull (Tokyo) 61:390–8
- Yatvin MB, Kreutz W, Horwitz B, Shinitzky M. (1980a). Induced drug release from lipid vesicles in serum by pH-change. Biophys Struct Mech 6:233–4
- Yatvin MB, Kreutz W, Horwitz BA, Shinitzky M. (1980b). pH-sensitive liposomes: possible clinical implications. Science 210:1253–5
- Yoshikawa T, Okada N, Oda A, et al. (2008). Development of amphiphilic g-PGA-nanoparticulate cytosolic protein delivery carrier. Biochem Biophys Res Commun 366:408–13
- Yu B, Tai HC, Xue W, et al. (2010). Receptor-targeted nanocarriers for therapeutic delivery to cancer. Mol Membr Biol 27:286–98
- Yuba E, Kojima C, Harada A, et al. (2010). pH-sensitive fusogenic polymer-modified liposomes as a carrier of antigenic proteins for activation of cellular immunity. Biomaterials 31:943–51
- Yuba E, Harada A, Sakanishi Y, et al. (2013). A liposome-based antigen delivery system using pH-sensitive fusogenic polymers for cancer immunotherapy. Biomaterials 34: 3042–52
- Zhou F, Huang L. (1995). Delivery of protein antigen to the major histocompatibility complex class I-restricted antigen presentation pathway. J Drug Target 3:91–109
- Zignani M, Drummond DC, Meyer O. (2000). In vitro characterization of a novel polymeric-based pH-sensitive liposome system. Biochim Biophys Acta 1463:383–94