Abstract
Tamibarotene (Am80), a poorly water-soluble drug for the treatment of acute promyelocytic leukemia (APL), loaded nanostructured lipid carrier (Am80-NLC) was developed and characterized previously. The purpose of the present work was to develop PEGylated nanostructured lipid carrier (PEG-NLC) for intravenous delivery of Am80, with the aim to further extend the circulation in blood and decrease the adverse events. Am80-loaded PEG-NLC (Am80-PEG-NLC) modified with PEG-40 stearate (PEG40-SA, molecular weight 2000 Da) was formulated by the method of melt-emulsification and low temperature-solidification technique. Am80-NLC was developed as well as control. Based on the optimized results of single-factor screening experiment, the average drug entrapment efficiency, the mean particle size, and zeta potential of Am80-NLC and Am80-PEG-NLC were found to be 89.8–94.3%, 178.9–201.6 nm, and −37.74 to −20.1 mV, respectively. In vitro drug release of Am80-NLC and Am80-PEG-NLC possessed a sustained release characteristic and their release behavior was in accordance with the Ritger–Peppas equation. In vivo, after intravenous (i.v.) injection to rats, the mean residence time (MRT) of Am80-PEG-NLC group was significantly prolonged and the AUC value was improved as well compared with the Am80-NLC group. Furthermore, the biodistribution in mice showed that Am80-PEG-NLC preferentially decreased the accumulation of Am80 in kidney and increased the drug concentration in brain after i.v. injection. In conclusion, Am80-PEG-NLC may be a potential delivery system for Am80 in the treatment of APL.
Introduction
Am80 (molecular structure shown in ), a retinoic acid receptor (RAR) α/β agonist, has been licensed the right for relapsed or refractory acute promyelocytic leukemia (APL) in Japan in 2005. It has drawn growing attentions in clinic due to high pharmacological activities and low toxicity in the treatment of APL (Takeuchi et al., Citation1997). In vitro, Am80 showed potent ability to induce of differentiation in human promyelocytic leukemia cells, such as NB-4 and HL-60 cells (Ohnishi, Citation2007). Besides, Am80 has been reported to have the potential for the treatment of rheumatoid arthritis, diabetic retinopathy, psoriasis, and solid tumors (Oikawa et al., Citation1993). At present, Am80 is commercially available in a 2 mg tablet (Amnolake®) for oral administration.
However, the bioavailability of Am80 was reported to be very limited (about 50–67%) due to its poor solubility of 2 µg/mL in water and first pass effect (Sugitani, Citation2009). Unfortunately, no appropriate coating process was applied to maintain a favorable oral absorption of the tablet (Kimura & Oyashiki, Citation2005). Besides, the mean biological half-time of a single dose of 2 mg Am80 was only 3.4 h, which suggested that frequent administration was required (Miwako & Kagechika, Citation2007). What’s worse, typical retinoid toxicities, such as hypertriglyceridemia and hypercholesterolemia, were diagnosed to be the most common adverse events associated with Am80 therapy (Tobita et al., Citation1997). Therefore, the development of Am80 dosage forms was limited as a result of the poor solubility in water, short elimination half-life, possible adverse events, and physiologically acceptable organic solvents.
Nanoparticle drug delivery systems are well known to be able to parenterally deliver of the hydrophobic drugs with higher efficiency and fewer adverse side effects (Joshi & Müller, Citation2009). Nanostructured lipid carrier (NLC), a type of lipid nanoparticle drug delivery system developed from solid lipid nanoparticles (SLN), can be prepared by mixing of solid lipids with spatially incompatible liquid lipids, which offer the advantages of improved drug loading and controlled release properties (Liu et al., Citation2011). Furthermore, NLC has been considered as an alternative to liposomes and SLN due to improved properties, such as good stability, high drug loading capacity, controlled drug release, and ease of manufacture. So NLC may be an ideal lipid-based drug delivery system for Am80 due to its attractive ability to increase the amount of drug penetrating into mucosa and prolong the circulation time of the encapsulated drugs (Luo et al., Citation2011; Yang et al., Citation2012).
However, it was reported that most of the intravenously applied delivery systems, such as liposomes, micelles, and nanoparticles, were identified as foreign products and quickly removed from the systemic circulation via recognition mainly by the cells of the mononuclear phagocyte system (MPS) and the polymorphonuclear leukocytes (Gref et al., Citation1995). So the design of a drug carrier that could avoid the recognition by the MPS or the reticuloendothelial system (RES) would be desirable for the short half-life drugs, such as Am80. It is reported that the nanoparticles modified by PEG can oppose uptake by the RES and increase the half-life in the circulation (Stolnik et al., Citation1995).
So in the present study, Am80-PEG-NLC modified with PEG40-SA was developed to improve the solubility of Am80 and prolong the circulation time in blood. PEG40-SA, an amphiphilic polymeric derivative of hydrophilic PEG modified by attaching a hydrophobic moiety, was reported to be easily incorporated into the lipid core of colloidal carriers with the hydrophilic PEG chain on their surface (Shen et al., Citation2009). Am80-PEG-NLC was prepared by the method of melt-emulsification and characterized by the morphology, particle size and its distribution, zeta potential, pH value, drug encapsulation efficiency and drug loading, and drug release behavior in vitro. With the control of Am80-NLC, the in vivo pharmacokinetics and biodistribution of Am80-PEG-NLC were also evaluated in detail to elucidate their feasibility as a novel intravenously administered anti-leukemia agent.
Material and methods
Materials
Am80 (99%) was synthesized by the laboratory of School of Pharmaceutical Science, Shandong University, Shandong, China. Soybean lecithin (Injection grade, phosphatidylcholine accounts for 95% pH 5.0–7.0) was provided by Shanghai Taiwei Pharmaceutical Co., Ltd, Shanghai, China. Glycerol monostearate was purchased from Chemical Agent factory of Tianjin Sitong (Tianjin, China). Stearic acid was provided by Tianjin Guangcheng Chemical Agent Co., Ltd, China (Tianjin, China). Oleic acid (Chemical Agent factory of Tianjin Damao, Tianjin, China) was chosen as liquid lipid material. Polyethylene glycol 40 Stearate (PEG40-SA) and Poloxamer188 (F68) were purchased from Sigma (St Louis, MO). Methanol, ethanol, and other chemicals and reagents used were of analytical grade.
Methods
Preparation of Am80-PEG-NLC and Am80-NLC
The formulations of Am80-PEG-NLC and Am80-NLC without PEG modification were presented in . The lipid and aqueous phases were prepared separately. Am80-PEG-NLC and Am80-NLC were prepared by the technique of melt-emulsification and low temperature-solidification. Briefly, Am80 and soybean lecithin, monostearin, oleic acid, and PEG40-SA/ stearic acid were weighted precisely and co-dissolved in 5 mL ethanol in water bath at 75 °C as a lipid phase. The aqueous phase was prepared by dissolving 1.5 wt% F68 in 15 mL distilled water. The aqueous phase was also kept at 75 °C in a water bath. Then the hot organic phase was dispersed with a microinjector (Kd Scientific 781100, Fabrique’ Auxetats-Units Co. Ltd., Santa Clara, CA) into the aqueous phase of the same temperature and was stirred simultaneously at 600 rpm for 0.5 h with an electromagnetic. The resultant warm nanoemulsion was quickly solidified by immersing beaker into icy water (0 °C) under stirring at 600 rpm for 1.5 h. Then the resultant Am80-NLC and Am80-PEG-NLC were stored at 4 °C.
Table 1. Compositions of the two nanoparticle formulations of Am80.
Mannitol (5%, w/v) was used in the freeze-drying process as a cryoprotectant. First the obtained Am80-NLC and Am80-PEG-NLC dispersions were pre-frozen using an ultra-cold freezer (MDF-382E, Sanyo Electric Co., Ltd., Osaka, Japan) for 24 h at −80 °C. Then, the resultant samples were transferred to the lyophilizer (FD5-2.5, GOLD SIM, Issaquah, WA) at −60 °C for 48 h. The lyophilized powder was collected for further experiments.
Measurement of particle size, zeta potential, and pH value
Particle size, polydispersity index (PDI), and zeta potential of Am80-NLC and Am80-PEG-NLC were determined by laser light scattering using a Delsa™ Nano C Particle Analyzer (Beckman Coulter A53878, Otsuka Electronics Co. Ltd., Rockville, MD). All samples were diluted with double-distilled water to achieve an adequate scattering intensity prior to the measurement. Then about 1 mL of the diluted samples was filled in the absorption cell. The pH value of Am80-NLCs and Am80-PEG-NLC was determined with a digital pH meter (FE20, Mettler Toledo, Greifensee, Switzerland). Each measurement was made at least in triplicate at 25 °C.
Transmission election microscope (TEM) examination
The morphology of Am80-PEG-NLC was observed by transmission electron microscopy (H-7000, Hitachi, Shiga, Japan). Am80-PEG-NLC was diluted with distilled water before the measurements. Then the diluted formulation was negatively stained with 2% phosphotungstic acid for 30 s and then dropped on a 200-mesh copper grid with films for observation.
Determination of entrapment efficiency and drug loading
The method of dissolution–ultracentrifugation was applied to separate free Am80 and the nanoparticles in both Am80-PEG-NLC and Am80-NLC (Liu et al., Citation2010). To determine the free Am80, 0.1 mL of Am80-PEG-NLC or Am80-NLC suspension was dispersed in 1.9 mL of 0.2 wt% Tween 80 phosphate buffer solution (PBS, pH 7.4) and shaken (XW-80 A, Instruments factory of Shanghai Medical University, Shanghai, China) for 3 min to dissolve the free drugs. Then the dispersion was centrifuged at 15 000 rpm for 1 h (3K30, Sigma, Schnelldorf, Germany). The supernatant was withdrawn and then filtrated through 0.22 µm membrane filters. Then the drug content in the filtrate was determined by the HPLC method using an Agilent G1310A pump and an Agilent G1314A variable wavelength detector set at 290 nm. A Platisil™-C18 column (Agilent Technologies, Palo Alto, CA) (250 mm × 4.6 mm, 5 µm) was used. The mobile phase involved methanol–5% acetic acid aqueous solution mixture at 9:1 (v/v). The flow rate was 1 mL/min at room temperature. The total Am80 concentration in Am80-PEG-NLC or Am80-NLC was measured using the same HPLC method after dissolving 0.1 mL of Am80-PEG-NLC or Am80-NLC in 1.9 mL of methanol. The drug entrapment efficiency (EE) and drug loading (DL) were calculated according to EquationEquations (1)(1) and (Equation2
(2) )
(1)
(2)
where Wtotal, Wfree, and Wlipid are the weights of drug added in the system, analyzed weight of Am80 in supernatant, and the weight of lipid added in system, respectively.
In vitro release assay
Release of Am80 from Am80-PEG-NLC was examined in 0.3 wt% Tween 80 PBS (pH 7.4) using a dialysis technique. Briefly, 2 mL of Am80-PEG-NLC suspension (Am80-PEG-NLC lyophilized powder re-dispersed with normal saline) was transferred to a dialysis bag with a cut-off molecular weight of 8–12 kDa. The dialysis bag was immersed in 30 mL of the release medium which was filled in a well-closed tube. The tubes were placed in a chamber and shaken horizontally (100 strokes/min) at 37 ± 0.5 °C. The dissolution medium was refreshed at scheduled time intervals (0.083, 0.17, 0.5, 1, 2, 4, 6, 8, 10, 12, 24, 36, and 48 h) and its drug content was determined by the HPLC method. The drug release profile of Am80-NLC was examined as a control. Each releasing experiment was performed in triplicate.
Pharmacokinetics studies
Wistar rats (weighed between 200 and 220 g, female or male were provided by the Medical Animal Test Center of Shandong University) were used for in vivo pharmacokinetics studies. These rats were fasted overnight before drug administration. Pharmacokinetics of Am80-PEG-NLC was compared with Am80-NLC. Ten Wistar rats (weighed between 200 and 220 g) were divided at random into two groups and intravenously injected of Am80-NLCs and Am80-PEG-NLC at a single dosage of 4.5 mg/kg, respectively.
After administration, 0.3 mL of blood sample was collected via the sinus jugularis at predetermined time points (0.17, 0.5, 1, 2, 4, 6, 8, 10, 12, and 24 h) and centrifuged for 10 min at 12 000 rpm immediately to obtain plasma. Then the plasma was removed and stored at −20 °C.
Tissue distribution studies
Kunming strain mice (weighed between 18 and 22 g, female or male were provided by the Medical Animal Test Center of Shandong University) were used for in vivo biodistribution studies. And the experimental procedure complied with the ethics and regulations of animal experiments of Pharmaceutical Sciences, Shandong University. One hundred Kunming strain mice were allocated at random to two groups and intravenously administrated of Am80-NLCs and Am80-PEG-NLC via tail vein at a single dose of 12 mg/kg, respectively. At predetermined time points (0.17, 0.5, 1, 2, 4, 6, 8, 10, 12, and 24 h), five mice were sacrificed. Tissues of heart, liver, spleen, lung, kidney, and brain were immediately removed at each time point, weighed, and homogenized with saline. The plasma and tissue samples were frozen at −20 °C until analysis.
HPLC analysis of Am80
Each plasma sample was extracted as the following procedure. About 1 mL of plasma was vortex mixed with 2 mL methanol for 3 min. The denatured protein precipitate was separated by centrifugation at 12 000 rpm for 10 min. Then, 2 mL of the supernatant organic layer was dried under nitrogen flow at 40 °C and redissolved in 200 µL mobile phase. About 20 µL of the resulting solution was directly injected into HPLC after filtering through a 0.22 µm filter for analysis.
Organs from mice for each treatment group were pooled and accurately weighed, then homogenized after the addition of 1 mL physiologic saline (except for liver, 2 mL). Tissue homogenates were processed as the plasma sample and analyzed by HPLC.
The plasma and tissue samples were all determined by an Agilent 1200 HPLC system (Agilent Technologies, Palo Alto, CA). An Eclipse XDB-C18 column (250 mm × 4.6 mm, 5 µm) was used. The other HPLC condition was the same as described in Section 2.2.4.
Statistical analysis
Data were expressed as mean ± standard deviation (SD). The statistical analysis of significance among various treatments was performed using unpaired Student’s t-test with p < 0.05 indicating significant difference. An analysis of variance (ANOVA) test was also used if necessary. Pharmacokinetic parameters of Am80-NLC and Am80-PEG-NLC were obtained using DAS2.0 (drug and statistics for windows) program.
Results and discussion
Characterization of Am80-PEG-NLC
The photographs of fresh-prepared Am80-PEG-NLC (A), the freeze-dried powder (B), and the suspension of freeze-dried powder (dispersed with distilled water) (C) are shown in . The result of TEM image of Am80-PEG-NLC is shown in , which displayed that Am80-PEG-NLC possessed a relatively spherical shape and non-adherent among each other. The average particle size, size distribution, zeta potential, and entrapment efficiency of the two formulations were reported in . The mean particle size of the two formulations was between 178.9 and 201.6 nm. Furthermore, the PDI of both Am80-NLC and Am80-PEG-NLC was below 0.3, which revealed that the two formulations had a quite narrow size distribution and uniform size (Zhang et al., Citation2011). The zeta potential values of Am80-NLC and Am80-PEG-NLC were detected about −34.69 mV and −21.14 mV, respectively. The results revealed that the zeta potential of Am80-NLC nanoparticle decreased after PEG modification. This phenomenon could be explained by the fact that the PEG chain scattered on the surface of the nanoparticles reduced the surface charge (Jia et al., Citation2010). The pH values of Am-80-NLC and Am80-PEG-NLC were determined to be 4.5 and 4.4, respectively, which were within the safe pH scope of 4–9 for intravenous injection. The mean drug entrapment efficiency of Am-80-NLC and Am80-PEG-NLC was 90.85% and 92.16%, respectively. It is reported that effective entrapment occurred when the drug solubility in lipids was high (Xu et al., Citation2011). So, high entrapment efficiency of the two formulations may be explained by the good solubility of Am80 in the lipids. And the higher entrapment efficiency of Am80-PEG-NLC may be attributed that PEG increase the affinity of Am80 (Zhang et al., Citation2011) and the increased liquid lipid content of Am80-PEG-NLC (Yuan et al., Citation2007; Jia et al., Citation2012).
Figure 2. Photographs of Am80-PEG-NLC: (A) fresh-prepared, (B) freeze-dried, and (C) the suspension of freeze-dried powder.
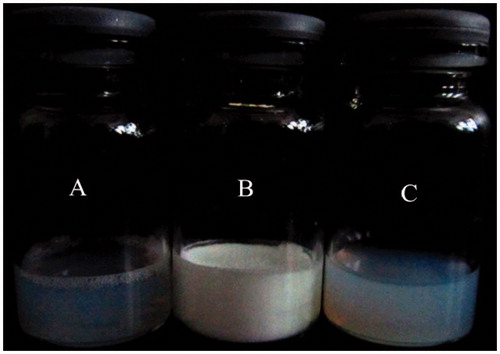
Table 2. The mean particle size, size distribution, zeta potential, and entrapment efficiency of the two Am80 formulations, each data was expressed as mean ± SD (n = 5).
In vitro release of drug
In vitro drug release behavior of Am80-PEG-NLC was compared with Am80-NLC, which was shown in . The release Am80 from Am80-PEG-NLC was fitted to the Ritger–Peppas equation and the equation was as follows: ln Q = 0.6175 ln t + 1.9416, r = 0.9738. The release behavior of Am80-NLC was previously reported to be in accordance with the Ritger–Peppas equation: ln Q = 0.2881 ln t + 3.4734, r = 0.9810 (Liu et al., Citation2013). The release profile of Am80 from both Am80-NLC and Am80-PEG-NLC was observed to be biphasic. The relative burst release of Am80 from Am80-NLC and Am80-PEG-NLC was at the initial stage, which could be explained by the fact that part of liquid lipids was located at the outer shell of the nanoparticles and the drug loaded in the liquid-lipid-enriched outer layers might be easily released (Jia et al., Citation2012). Subsequently, the two kinds of NLC were observed to exhibit a certain sustained release characteristic. The relative sustained release trend of this phase could be attributed to the fact that lipophilic Am80 was held by the solid-lipid core of NLC and subsequently drug release from the lipid matrices mainly depended on the co-effect of bulk erosion and diffusion (Zhang et al., Citation2008). The drug releasing rate of Am80 increased with the modification of PEG-SA, which could be explained by the fact that amphiphilic PEG chains located on the surface of nanoparticles could decrease the surface tension of nanoparticles and make the nanoparticles surface to be wet more easily (Yuan et al., Citation2007). Besides, the increased liquid-lipid Am80-PEG-NLC may contribute to its faster release as well. Because, compared with Am80-NLC, the increased liquid lipids of Am80-PEG-NLC were located at the outer shell of the nanoparticles besides distributed in solid-lipid core, so the liquid-lipid-enriched outer layers could load a larger amount of drug which might be easily released (Jia et al., Citation2012). However, the in vitro release data only stands for the dissolution processes of Am80 in the artificial body fluid. So the sustained release behavior of Am80-PEG-NLC should be further demonstrated by the pharmacokinetic and biodistribution studies.
Pharmacokinetics studies
At the range of 0.2–20 µg/mL, the standard curve was A = 28.83C + 6.7532 and the standard showed a good linearity with a correlation coefficient of 0.9995. The plasma concentration–time profile after single i.v. injection of Am80-NLC and Am80-PEG-NLC at a dose of 4.5 mg/kg is shown in . After i.v. administration, the plasma Am80 concentration of Am80-PEG-NLC group was much higher than that of the Am80-NLC group at each sampling time. Furthermore, Am80-PEG-NLC was eliminated more slowly than Am80-NLC though Am80-PEG-NLC had a faster drug release rate in vitro. Besides, following injection of Am80-PEG-NLC, Am80 concentration was still measured to be 0.68 mg/L after 24 h, while a relative low concentration was detected in the Am80-NLC group after 8 h.
Figure 5. Mean plasma concentration–time curves of Am80 in rats after i.v. administration of Am80-NLC and Am80-PEG-NLC at a dose of 4.5 mg/kg (n = 5).
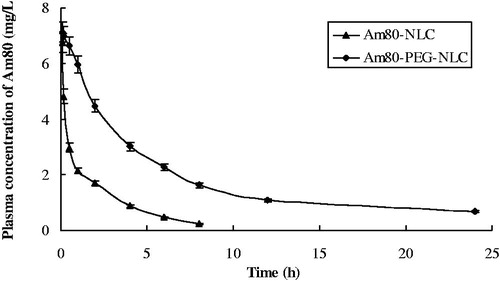
The main corresponding pharmacokinetic parameters AUC and t1/2 for Am80-NLC and Am80-PEG-NLC are summarized in . The results calculated by DAS2.0 showed that Am80-NLC and Am80-PEG-NLC were fitted to a three-compartment model. As displayed in , Am80-PEG-NLC showed a longer circulation time than Am80-NLC, and MRT was 2.73-fold as large as Am80-NLC. Furthermore, the AUC value of Am80-PEG-NLC was 3.02 times higher than that of Am80-NLC.
Table 3. Main pharmacokinetic parameters of Am80 in rats after i.v. administration of Am80-NLC and Am80-PEG-NLC at a single dose of 4.5 mg/kg (mean ± SD, n = 5).
The colloidal drug carriers were taken up by the MPS organs following i.v. injection depending on their physicochemical characteristics, such as the particle size, the surface charge, and the surface hydrophobicity (Storm et al., Citation1995). It was reported that the amphiphilic PEG chains located on the surface of Am80-PEG-NLC nanoparticles forming a water shield on the surface of the nanoparticles, which could reduce adsorption of opsonins and avoid the recognition by the cells of a MPS (Kim et al., Citation2010). That is the main reason why Am80-PEG-NLC could remain in the circulation for a longer period of time than Am80-NLC. However, the influential factor on the adsorption decrease of opsonins by Am80-PEG-NLC was PEG surface density or PEG chain length should be further studied. Besides, a less negative or even neutral zeta potential was reported to contribute to the long-circulating effect (Su et al., Citation2011). As shown in , Am80-PEG-NLC possessed a less negative zeta potential compared with Am80-NLC (p < 0.05).
Biodistribution study
The standard curves of all measured organs were established within 0.1–20 μg/mL Am80 concentration and the correlation coefficients range from 0.9925 to 0.9996. The Am80 concentrations in heart, liver, spleen, lung, kidney, and brain of mice after i.v. administration of Am80-NLC and Am80-PEG-NLC were shown in and (). It can be clearly seen that the drug distribution was notably different in the Am80-PEG-NL group and the Am80-NLC group. Briefly, the concentration of Am80 in kidney, an important excretory organ for i.v. delivery of Am80, was much lower for the Am80-NLC group, which may be expected to reduce or avoid the potential side effects to kidney (Miwako & Kagechika, Citation2007). This phenomenon may be explained by the fact that PEGylation results in a decrease of the renal clearance (Harris et al., Citation2001). Furthermore, Am80-PEG-NLC resulted in a higher drug accumulation in brain compared with Am80-NLC (p < 0.05), which may be explained by the fact that the RES removal of the nanoparticles can be prevented by surface coating with hydrophilic polymers, such as PEG and F68, to increase their availability at brain (Kaur et al., Citation2008). Except for the treatment of APL, Am80 has been reported to have potential for the treatment of some neurological disorders as well, such as Alzheimer’s disease, age-related dementia, and Parkinson’s disease (Shudo et al., Citation2004; Kawahara et al., Citation2009). So the higher Am80 concentration in brain may contribute to the treatment of these neurological disorders.
Figure 6. Am80 concentration in tissue at different time points after i.v. administration of (A) Am80-NLC and (B) Am80-PEG-NLC (n = 5).
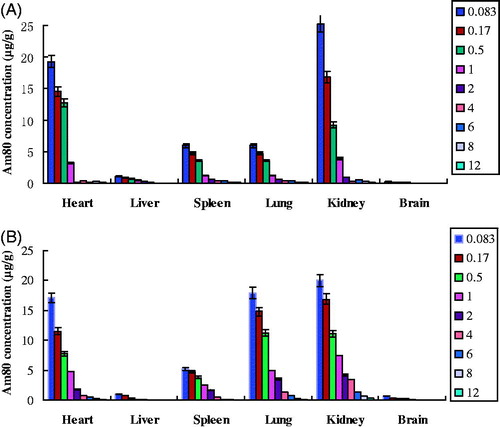
The Am80 concentration percentage in different tissues of mice at 5 min following i.v. administration of Am80-NLC and Am80-PEG-NL is shown in . PEG chains scattered on the surface of nanoparticles were reported to dramatically reduce the uptake of nanoparticles via i.v. injection into the RES cells and subsequently reduced the drug accumulation in liver and spleen (Beletsi et al., Citation2005). Previously, we have reported that Am80-NLC had a much lower drug concentration in liver than Am80 solution (Liu et al., Citation2013). However, in the present work, the drug distribution in liver of the Am80-PEG-NLC group did not significantly reduce following PEG modification compared with the Am80-NLC group (p < 0.01). The low initial drug concentration in liver of the Am80-NLC group may be one of the reasons. In contrast, the spleen drug concentration in the Am80-PEG-NLC group declined slightly compared with the Am80-NLC group.
Figure 7. Am80 concentration percentage of different tissues at 5 min after i.v. administration of Am80-NLC and Am80-PEG-NLC (n = 5).
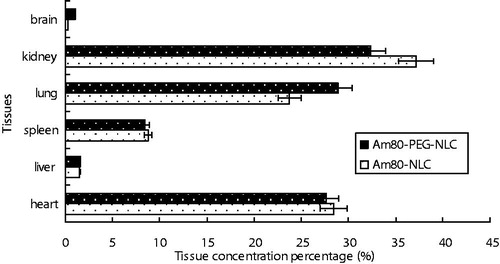
Furthermore, compared with the Am80-NLC group, a higher drug accumulation in the lung was observed when the Am80-PEG-NLC group was intravenously injected. Although effects of PEGylation regarding the translocation across the air–blood–barrier have not been investigated, yet, the fact that PEG surface modification decreases the macrophages uptake and cellular uptake result in an increase of the availability of free NPs on the epithelial cells of lung (Malugin & Ghandehari, Citation2010; Lipka et al., Citation2010). Besides, high drug accumulation in heart was observed in both groups. Fortunately, the detailed data of toxicology studies at the website of pharmaceuticals and medical devices agency (PMDA) of Japan revealed that no obvious cardiotoxicity was observed in rats after orally and subcutaneously administered of Am80.
Conclusions and outlook
In this work, Am80-loaded Am80-PEG-NLC modified with PEG40-SA was successfully developed and parenterally delivered. The in vitro and in vivo physicochemical properties of Am80-PEG-NLC were characterized and evaluated in detail. The in vitro drug release experiments exhibited biphasic drug release characteristic and faster release than Am80-NLC. The pharmacokinetics studies confirmed that the circulation time of Am80 in blood can be extended by Am80-PEG-NLC coated with PEG chains on the surface. The tissue distribution study indicated that Am80-PEG-NLC decreased the drug accumulation in kidney following i.v. injection in mice. We, therefore, conclude that PEG-NLC may serve as a promising carrier for systematic delivery of Am80 in the treatment of APL.
Acknowledgements
The authors would like to express thanks to the laboratory of School of Pharmaceutical Science, Shandong University for providing the bulk drugs of Am80.
Declaration of interest
The authors report no conflicts of interest. The authors alone are responsible for the content and writing of this article.
References
- Beletsi A, Panagi Z, Avgoustakis K. (2005). Biodistribution properties of nanoparticles based on mixtures of PLGA with PLGA–PEG diblock copolymers. Int J Pharm 298:233–41
- Gref R, Domb A, Quellec P, et al. (1995). The controlled intravenous delivery of drugs using PEG-coated sterically stabilized nanospheres. Adv Drug Deliv Rev 16:215–32
- Harris JM, Martin NE, Modi M. (2001). Pegylation: a novel process for modifying pharmacokinetics. Clin Pharmacokinet 40:539–51
- Jia L, Shen J, Zhang D, et al. (2012). In vitro and in vivo evaluation of oridonin-loaded long circulating nanostructured lipid carriers. Int J Biol Macromol 50:523–9
- Jia L, Zhang D, Li Z, et al. (2010). Nanostructured lipid carriers for parenteral delivery of silybin: biodistribution and pharmacokinetic studies. Colloids Surf B Biointerfaces 80:213–8
- Joshi MD, Müller RH. (2009). Lipid nanoparticles for parenteral delivery of actives. Eur J Pharm Biopharm 71:161–72
- Kaur IP, Bhandari R, Bhandari S, Kakkar V. (2008). Potential of solid lipid nanoparticles in brain targeting. J Control Release 127:97–109
- Kawahara K, Nishi K, Suenobu M, et al. (2009). Oral administration of synthetic retinoid Am80 (Tamibarotene) decreases brain β-amyloid peptides in APP23 Mice. Biol Pharm Bull 32:1307–9
- Kim HK, Davaa E, Myung CS, Park JS. (2010). Enhanced siRNA delivery using cationic liposomes with new polyarginine-conjugated PEG-lipid. Int J Pharm 392:141–7
- Kimura Y, Oyashiki K. (2005). Evaluation of the new drug by the clinician. Tamibarotene. Clin Pract 24:991–3
- Lipka J, Semmler-Behnke M, Sperling RA, et al. (2010). Biodistribution of PEG-modified gold nanoparticles following intratracheal instillation and intravenous injection. Biomaterials 31:6574–81
- Liu D, Liu Z, Wang L, et al. (2011). Nanostructured lipid carriers as novel carrier for parenteral delivery of docetaxel. Colloids Surf B Biointerfaces 85:262–9
- Liu D, Wang L, Liu Z, et al. (2010). Preparation, characterization, and in vitro evaluation of docetaxel-loaded poly (lactic acid)-poly (ethyleneglycol) nanoparticles for parenteral drug delivery. J Biomed Nanotechnol 6:675–82
- Liu X, Wang Z, Feng R, et al. (2013). A novel approach for systematic delivery of a hydrophobic anti-leukemia agent Tamibarotene mediated by nanostructured lipid carrier. J Biomed Nanotechnol 9:1586–93
- Luo Q, Zhao J, Zhang X, Pan W. (2011). Nanostructured lipid carrier (NLC) coated with Chitosan oligosaccharides and its potential use in ocular drug delivery system. Int J Pharm 403:185–91
- Malugin A, Ghandehari H. (2010). Cellular uptake and toxicity of gold nanoparticles in prostate cancer cells: a comparative study of rods and spheres. J Appl Toxicol 30:212–7
- Miwako I, Kagechika H. (2007). Tamibarotene. Drugs Today (Barc) 43:563–8
- Ohnishi K. (2007). PML-RARa inhibitors (ATRA, tamibaroten, arsenic troxide) for acute promyelocytic leukemia. Int J Clin Oncol 12:313–7
- Oikawa T, Okayasu I, Ashino H, et al. (1993). Three novel synthetic retinoids, Re 80, Am 580 and Am 80, all exhibit anti-angiogenic activity in vivo. Eur J Pharmacol 249:113–6
- Shen J, Wang Y, Ping Q, et al. (2009). Mucoadhesive effect of thiolated PEG stearate and its modified NLC for ocular drug delivery. J Control Release 137:217–23
- Shudo K, Kagechika H, Yamazaki N, et al. (2004). A synthetic retinoid Am80 (Tamibarotene) rescues the memory deficit caused by scopolamine in a passive avoidance paradigm. Biol Pharm Bull 27:1887–9
- Stolnik S, Illum L, Davis SS. (1995). Long circulating microparticulate drug carriers. Adv Drug Deliv Rev 16:195–214
- Storm G, Belliot SO, Daemen T, Lasic DD. (1995). Surface modification of nanoparticles to oppose uptake by the mononuclear phagocyte system. Adv Drug Deliv Rev 17:31–48
- Sugitani M, Abe R, Ikarashi N, et al. (2009). Disposition of a new tamibarotene prodrug in mice. Biol Pharm Bull 32:1997–2001
- Su Z, Niu J, Xiao Y, et al. (2011). Effect of octreotide-polyethylene glycol(100) monostearate modification on the pharmacokinetics and cellular uptake of nanostructured lipid carrier loaded with hydroxycamptothecine. Mol Pharm 8:1641–51
- Takeuchi M, Yano T, Omoto E, et al. (1997). Re-induction of complete remission with a new synthetic retinoid, Am-80, for relapse of acute promyelocytic leukaemia previously treated with all-trans retinoic acid. Br J Haematol 97:137–40
- Tobita T, Takeshita A, Kitamura K, et al. (1997). Treatment with a new synthetic retinoid, Am80, of acute promyelocytic leukemia relapsed from complete remission induced by all-trans retinoic acid. Blood 90:967–73
- Xu X, Zhao C, Yang H, et al. (2011). Anti-inflammatory activity of injectable dexamethasone acetate-loaded nanostructured lipid carriers. Drug Deliv 18:485–92
- Yang X, Li Y, Li M, et al. (2012). Hyaluronic acid-coated nanostructured lipid carriers for targeting paclitaxel to cancer. Cancer Lett 334:338–45
- Yuan H, Wang L, Du Y, et al. (2007). Preparation and characteristics of nanostructured lipid carriers for control-releasing progesterone by melt-emulsification. Colloids Surf B Biointerfaces 60:174–9
- Zhang T, Chen J, Zhang Y, et al. (2011). Characterization and evaluation of nanostructured lipid carrier as a vehicle for oral delivery of etoposide. Eur J Pharm Sci 43:174–9
- Zhang X, Pan W, Gan L, et al. (2008). Preparation of a dispersible PEGylate nanostructured lipid carriers (NLC) loaded with 10-hydroxycamptothecin by spray-drying. Chem Pharm Bull 56: 1645–50