Abstract
The aim of this study was to investigate the potential of pioglitazone hydrochloride as a promising anticancer agent and then to design and evaluate the colon-targeted delivery system. The role of pioglitazone hydrochloride as a promising anticancer agent was evaluated by in vitro cell line studies and in vivo 1,2-dimethylhydrazine-induced colon carcinogenesis in rats. In order to deliver the drug at site of action, i.e. colon, drug embedded in matrices containing a release retarding polymer (HPMC K4M) and a polysaccharide (locust bean gum) were prepared. These matrix systems were further enteric coated with Eudragit®S100 to minimize the premature drug release in the upper segments of the GIT. In vitro dissolution studies were performed in absence and presence of rat caecal contents on selected batches and samples were analyzed using a validated RP-HPLC method. Hence, the studies led to the conclusion that successful site-specific delivery systems of pioglitazone hydrochloride were developed to improve its therapeutic efficacy in the management of colorectal cancer.
Introduction
Colorectal cancer is the third most commonly diagnosed cancer in males (663 000 cases, 10.0% of the total) and the second in females (570 000 cases, 9.4% of the total) over the globe. Over 1.2 million new cancer cases and 608 700 deaths have been estimated to have occurred in 2008 (Jemal et al., Citation2000; Ferlay et al., Citation2010). Currently available therapeutic approaches (cytotoxic chemotherapy, biologic response modifiers and radiation therapy) for advanced colorectal cancer (CRC) are only palliative. Thus, the use of chemopreventive agents is potentially important to suppress the progression of colon cancer (Krishnan & Ruffin, Citation2000).
Peroxisome proliferator-activated receptors (PPARs) are members of the nuclear hormone receptor superfamily of ligand-dependent transcription factors, and the three major PPAR isoforms are PPARα, PPARβ/δ and PPARγ (Kota et al., Citation2005). PPARγ plays an important role in adipocyte differentiation, lipid storage and energy dissipation in adipose tissue, and it is also involved in the control of inflammatory reactions and in glucose metabolism through the improvement of insulin sensitivity. The most widely used synthetic agents are thiazolidinediones (TZDs) including ciglitazone, troglitazone, pioglitazone and rosiglitazone, which have been used clinically to treat type 2 diabetes. Recently, PPARγ was shown to be expressed in many cancers such as prostate cancer, breast cancer, bladder cancer, thyroid cancer, colon cancer. A variety of PPARγ agonists have been described as potent inductors of growth arrest and apoptotic cell death in cancers.
Pioglitazone hydrochloride is an orally administered, insulin sensitizing TZD. A pilot study conducted by Takahashi et al. (Citation2010) suggested that pioglitazone (15 mg daily) may have a preventive potential for human aberrant crypt foci and was found to have a good safety profile in the study patient population. Pioglitazone is a selective agonist for nuclear PPARγ. Activation of PPARγ nuclear receptors modulates the transcription of a number of insulin responsive genes involved in the control of glucose and lipid metabolism. PPARγ activation promotes anti-tumor actions through apoptosis induction, differentiation and growth (proliferation) arrest. Furthermore, there has been no evidence of occurrence of hepatotoxicity with this analogue as compared to other drugs of this category, namely troglitazone and rosiglitazone; therefore, its use in adults is increasing enormously (Tan et al., Citation2004). Hence, it is a candidate of choice among the thiazolidinones for the development of colon-targeted drug delivery systems.
Various approaches have been used for the development of colon-targeted delivery systems including use of pH sensitive systems, microbially triggered systems, pressure-dependent release systems and timed release systems including osmotically controlled drug delivery systems. The polysaccharide-based delivery systems effectively shield the drug from the hostile environment of the GIT and aid in colon-targeted drug delivery. The naturally occurring polysaccharides are highly stable, safe, nontoxic, hydrophilic, gel forming and in addition biodegradable. They fall into the category of generally regarded as safe (GRAS) agents. After arrival to the colon, the glycosidic linkages within the polysaccharides get hydrolyzed releasing the drug candidate at the site of action. These natural polysaccharides can be utilized to develop the microbially triggered systems. The pH of the GIT is low in the stomach but increases when passing to the small and large intestine. For the purpose of targeting drugs to the colon, it is plausible, therefore, to coat tablets, capsules or pellets with a pH-dependent polymer that is insoluble at low pH but soluble at neutral or slightly alkaline pH. This pH differential in different segments of the GIT is the basic target for pH-sensitive polymers. These are the most preferred pharmaceutical dosage forms due to their relatively lower cost of production and the ease of manufacture as compared to the other more complex delivery systems (Friend, Citation2005).
The objective of this study was to design and evaluate the colon-targeted delivery system for pioglitazone hydrochloride. The chemopreventive potential of pioglitazone hydrochloride in CRC was evaluated by in vitro cell line studies and in vivo 1,2-dimethylhydrazine (DMH)-induced colon carcinogenesis in rats. An endeavor was made to prepare a suitable matrix system composed of a release retarding polymer (HPMC K4M; Ranbaxy Laboratories Ltd, Gurgaon, India) and a polysaccharide (locust bean gum; Himedia Laboratories Pvt. Ltd, Mumbai, India) which would provide adequate delay in commencement of drug release from the proposed matrix system. These matrix systems were further enteric coated with Eudragit®S100 (Corel Pharma Chem, Ahmedabad, India) to minimize the premature drug release in the upper segments of the GIT.
Material and methods
Materials
Methyl thiazolyl tetrazolium (MTT; Himedia Laboratories Pvt. Ltd, Mumbai, India), dimethyl sulfoxide (DMSO; Himedia Laboratories Pvt. Ltd, Mumbai, India), 5-fluorouracil (Ranbaxy Laboratories Ltd, Gurgaon, India), pioglitazone (Panacea Biotech, Lalru, India), Human colon cancer HCT-116 cells (ATCC), pioglitazone hydrochloride, locust bean gum, HPMC K4M and Eudragit®S100.
Methods
Chemopreventive potential of pioglitazone hydrochloride
In vitro cell line studies: MTT Assay
HCT-116 cells were plated (5000 per well) in 96-well plates and incubated for 72 h. The cells were treated with different concentrations of pioglitazone hydrochloride (1, 5, 10, 25, 50, 75, 100 μM/L). After 72 h, 20 μL of 5 mg/mL MTT was added to each well. The cells were then incubated at 37 °C for 4 h, after which the MTT medium was removed and 100 μL of DMSO was added. A subsequent color reaction was measured using a spectrometer at wavelength of 540 nm. The proliferation inhibition rate (IR) was calculated according to the following formula:
(1)
Also, 50 μmol/L pioglitazone hydrochloride was co-administered with 1, 5, 10, 25, 50, 75, 100 μmol/L of 5-fluorouracil, respectively, to HCT-116 cells for 72 h and same procedure was followed.
In vivo animal studies: DMH-induced colon carcinogenesis in rats
Animals
Male Wistar rats were acquired from the Central Animal House of Panjab University. All experiments were conducted after approval from Institutional Animal Ethics Committee, Panjab University, Chandigarh, India. The animals were housed in plastic cages embedded with rice husk and maintained on standard animal feed and had free access to water.
Experimental design
Animals were segregated into four groups, namely normal control, DMH treated, DMH + pioglitazone 1 (low dose) and DMH + pioglitazone 2 (high dose) (). Colon carcinogenesis was induced through weekly subcutaneous injections of DMH (Sigma-Aldrich, USA; 30 mg/kg body weight) dissolved in 1 mM EDTA–normal saline (pH 6.5), for 16 weeks (Dani et al., Citation2007). Pioglitazone (in the form of pioglitazone hydrochloride) was daily administered perorally suspended in 0.5% carboxy-methylcellulose sodium salt at low-dose level (1.5 mg/kg body weight) and at high-dose level (4.5 mg/kg body weight). At the end of 16-week duration, the animals of each group were over anaesthetized with ether and sacrificed.
Table 1. Treatment groups.
Colon tumor analysis
The rats were fasted overnight prior to the day of termination of the experiment. Subsequent to 16 weeks of DMH treatment and after the terminal sacrifice, colon was removed, flushed with physiological saline and opened longitudinally. The inner surface of colon was meticulously examined macroscopically for the presence of tumors. Different regions of colon were also examined under hand lens for counting the tumors. Chemopreventive response was assessed on the basis of tumor incidence and multiplicity, which were calculated as follows:
Tumor incidence (percentage of animals having tumors)
Tumor multiplicity (total number of tumors counted/number of tumors bearing rats)
Body weight changes
The animals were weighed at the commencement of the experiment and twice a week, and finally before sacrificing them to observe the body weight changes of control, DMH and drug-treated animals throughout the study.
Preparation of colon homogenate
The animals were sacrificed by giving ample ether anesthesia. Without delay the colons were removed, rinsed with ice-chilled saline and weighed (Dani et al., Citation2007). For histological examination at the light microscopic level, a small portion of colon was immersion fixed in 10% phosphate-buffered formalin. Colon homogenates (10%) were prepared in ice-cold Tris-Mannitol buffer (2 mM Tris, 50 mM Mannitol, pH 7.2) using mechanically driven tissue homogenizer for few minutes till entire disruption of cells. Homogenates were centrifuged at 10 000 × g for 10 min at 4 °C and pellets were discarded. Supernatant was stored at −20 °C and used for various biochemical estimations.
Biochemical estimations
To signify the antioxidant levels of animals, total lipid peroxidation (LPO) levels and several key enzymes were analyzed. Various established biochemical procedures were used as follows: LPO (Wills, Citation1966), catalase (Luck, Citation1954), superoxide dismutase (SOD) (Kono, Citation1978) and reduced glutathione (GSH) (Ellman, Citation1959).
Histopathological studies
For the histopathological interpretation at light microscopic level, fresh tissue pieces of colon were immersion fixed in buffered formalin. Following an overnight fixation, the specimens were dehydrated in ascending grades of alcohol, cleared in benzene and embedded in paraffin wax. Blocks were made and 5- to 7 -µm-thick sections were double stained with hematoxylin and eosin (H/E) and observed under light microscope.
Preparation of colon-targeted drug delivery systems: matrix tablets
Preparation of core tablets
Matrix tablets of pioglitazone hydrochloride containing locust bean gum, HPMC K4M, microcrystalline cellulose as the diluent, magnesium stearate (1%) as the lubricant and talc (2%) as the glidant were prepared. Initially, three batches of locust bean gum in the proportion of 20%, 30% and 50% (LB20, LB30 and LB50) were prepared. Also varying proportions of HPMC K4M (20, 30, 40% w/w) were formulated with 30% w/w locust bean gum (LBH1, LBH2 and LBH3, respectively). Tablets were made by first mixing all the excipients along with the drug (16.54 mg) thoroughly followed by sieving to obtain a uniform blend. Finally, the lubricant and glidant were added and the blend was further mixed for 15 min and then compressed into tablets on Cadmach 16 station rotary tablet machine using 8.2 mm standard concave punches. The powder blend equivalent to 200 mg was weighed and compressed individually.
Enteric-coated matrices
Preparation of coating solution
The coating solution (6.0% w/v) containing Eudragit®S100 was prepared in mixture of ethanol and isopropyl alcohol (1:2) using dibutyl phthalate as the plasticizer. The solution was stirred for sufficient period of time in order to obtain a clear solution.
Coating of core tablets
The tablets of batch LBH2 were coated at different levels of coating using pan coating method and dried with the help of dryer with an inlet air temperature of 35–45 °C. Batches LBH2A, LBH2B, LBH2C, LBH2D and LBH2E coated with 1.915%, 4.119%, 5.99%, 7.91% and 10.08% w/w layer of Eudragit®S100 (6.0% w/v), respectively, were prepared. The coating process was continued till the desired level of coating thickness was achieved. The coating thickness was measured on the basis of coat weight with respect to the weight of matrix tablets.
shows the composition of core of matrix tablets prepared with different ratios of locust bean gum and HPMC K4M.
Table 2. Composition of core of matrix tablets containing different ratios of locust bean gum and HPMC K4M.
Evaluation of pioglitazone hydrochloride tablets
Preparation of rat caecal content medium
Wistar rats, 150–200 g, fed on a normal diet were killed by spinal traction 45 min before the in vitro studies. The abdomens of the rats were opened and the caecal were located, which was ligated at both the ends. The caecal contents were removed, weighed and immediately transferred into pH 6.8 buffer under carbon dioxide bubbling. The suspension was filtered through a muslin cloth and added to the dissolution media to give a final caecal content concentration of 2% w/v. All the above procedures were carried out under carbon dioxide to maintain anaerobic conditions.
Release experiments
To study the in vitro release profiles, the dissolution test was carried out according to USP 30 method for delayed release tablets (Method A). Dissolution study was carried out using USP apparatus type II, i.e. paddle type at 50 rpm. The temperature of the media was maintained at 37 ± 0.5 °C. Initial studies were carried out in 750 mL of 0.1 N HCl (pH 1.2) for 2 h, after which 250 mL of a 0.2 M trisodium orthophosphate solution was added into the dissolution media and pH was adjusted to 6.8. To maintain the sink conditions, sodium lauryl sulphate (1% w/v) was added to the dissolution medium. Samples were collected at various time intervals and analyzed for pioglitazone hydrochloride content using a UV-spectrophotometer at λmax 269 nm (Laxmi et al., Citation2010). The withdrawn volume was replaced with the equal volume of fresh dissolution medium maintained at 37 ± 0.5 °C.
Drug release studies in the presence of rat caecal contents were carried out to assess the degradability of the polysaccharides (used in preparation of tablets) to the colonic enzymes (Sinha et al., Citation2004). Samples were filtered through a 0.45 µm membrane filter and were analyzed by a validated RP-HPLC method aided with a UV-spectrophotometer (λmax = 225 nm).
Results and discussion
Chemopreventive potential of pioglitazone hydrochloride
There is need to identify potentially new therapeutic agents for alleviation of CRC. Investigators have been weighing upon the role of PPARγ in CRC as PPARγ is highly expressed in human colon and CRC (Sarraf et al., Citation1998; Brockman et al., Citation1998). Anti-diabetic TZDs are potent PPARγ agonists that induce suppression, differentiation and reversal of malignant changes in colon cancer in vitro (Shimada et al., Citation2002; Kato et al., Citation2004). Whereas, reports have showed that TZDs enhance colon polyp development in APCmin mice in vivo (Lefebvre et al., Citation1998). These conflicting results have not yet been thoroughly explained. To have a clear picture of chemopreventive potential of pioglitazone hydrochloride, PPARγ ligand, in CRC in vitro cell line studies and in vivo animal studies were performed.
In vitro cell line studies: MTT Assay
To evaluate the effect of specific PPARγ ligand on the cell growth of human CRC cells, HCT-116 cells were treated with pioglitazone hydrochloride (1, 5, 10, 25, 50, 75, 100 μmol/L) for 72 h. The % IR of the drug increased from 2.96 ± 0.477 to 15.374 ± 0.552% with increase in concentration from 1 to 100 µmol/L as shown in .
Figure 1. (a) Effect of pioglitazone hydrochloride on proliferation and growth of HCT-116 exposed to pioglitazone HCl only for 72 h. (b) Effect of pioglitazone hydrochloride and/or 5-FU on proliferation and growth of HCT-116 exposed to 5-FU with or without 50 µmol/L pioglitazone HCl for 72 h. (Data are expressed in mean ± SEM. mp < 0.001, sp < 0.010 and np < 0.050 by one-way ANOVA followed by Tukey’s test when compared with pioglitazone hydrochloride group).
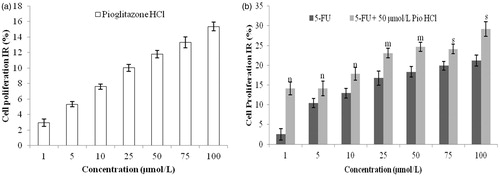
Since the IR value for the drug at 50 μmol/L was 11.797%, 50 μmol/L pioglitazone hydrochloride was co-administered with 1, 5, 10, 25, 50, 75, 100 μmol/L of 5-fluorouracil, respectively, to HCT-116 cells for 72 h. As shown in , 5-fluorouracil inhibited proliferation of HCT-116 cells in a dose-dependent manner and pioglitazone hydrochloride significantly enhanced the proliferation inhibition of HCT-116 induced by 5-fluorouracil. The findings were in agreement with the results of Shen et al. (Citation2007), which demonstrated that PPARγ agonists inhibited the growth and induced differentiation of human colon cancer cells. Thus, a significant therapeutic synergism was also obtained between both the drugs. Hence, the above study indicates the potential of the PPARγ ligand in chemoprevention of colorectal carcinoma.
In vivo animal studies: DMH-induced colon carcinogenesis in rats
The prophylactic effect of pioglitazone hydrochloride was evaluated by studying its role in regulation of key antioxidant enzymes as well as investigations on histological alterations in the colon of rats subjected to DMH (an organo-specific carcinogen) treatment.
Colon tumor analysis
In the carcinogen Group II, tumor incidence was 100%. Administration of PPARγ ligand to DMH-treated animals resulted in reduction in tumor incidence () 87.14% and 75% at dose levels 1.5 and 4.5 mg/kg body weight, respectively, when compared to DMH Group II. The average number of tumors per tumor bearing rat (tumor multiplicity) was also considered in the study. In addition, rats treated with DMH + pioglitazone HCl in Groups III and IV showed a decline in tumor multiplicity compared to rats administered with DMH only ().
Table 3. Chemopreventive efficacy of pioglitazone hydrochloride on the incidence and multiplicity of DMH-induced rat colonic tumors.
Table 4. Effect of pioglitazone hydrochloride on lipid peroxidation and antioxidant defence system enzymes in the colon of rats subjected to DMH treatment.
The higher dose of the drug (4.5 mg/kg) indicated a more significant reduction in tumor multiplicity than the lower dose (1.5 mg/kg). Furthermore, a significant reduction in colon tumor size was also evident in Groups III and IV when compared with those in carcinogen Group II (p < 0.05). In Groups III and IV, no significant difference was observed in tumor size. depicts comparative gross anatomy of the colon of (a) control (Group I), (b) DMH (Group II), (c) DMH + Pio1 (Group III) and (d) DMH + Pio2 (Group IV).
Figure 2. Comparative gross examination showing the effects of pioglitazone hydrochloride on the morphological features of the colonic mucosal surface during DMH-induced colon carcinogenesis. (a) (Group I) Normal colon of control animal, (b) (Group II): colon of DMH-treated animal, (c) (Group III): colon of DMH + Pio1-treated animal, (d) (Group IV): colon of DMH + Pio2-treated animal.
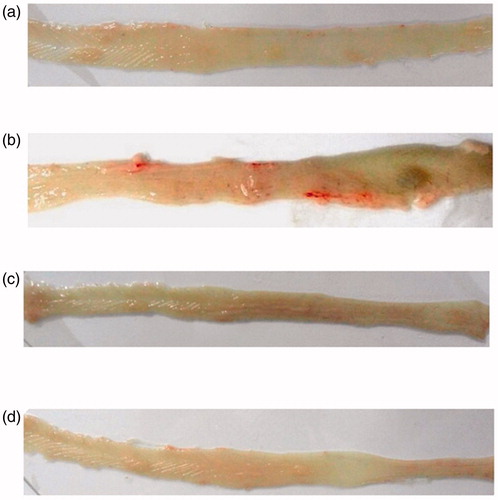
A reduction in the tumor incidence, tumor multiplicity, with concomitant reduction in average tumor size was observed when rats were treated with pioglitazone HCl for 16 weeks. This has put forward the potential of TZD pioglitazone HCl, in inhibiting/slowing tumorogenesis, in the rat colon.
Body weight changes
The body weights of the normal control animals showed gain of 101.98% throughout the study. However, DMH treatment resulted in significant less increase in body weight of 62.34% (p < 0.001), when compared to normal control rats. Pioglitazone hydrochloride treatment to DMH-treated rats tended to improve the body weight growth in comparison to Group II animals (p < 0.05).
Biochemical estimations
Various biochemical estimations were performed to evaluate the effect of pioglitazone HCl on LPO and antioxidant defence system enzymes in the colon of rats subjected to DMH treatment ().
Effect on LPO
Pioglitazone hydrochloride treatment to Group III and IV animals significantly reversed (p < 0.05) the otherwise LPO levels when DMH-treated animals were compared with drug-treated group at the end of the study. The LPO levels of Group III and IV were comparable with no significant difference.
The basis of decreased LPO in the colon following DMH treatment has been the assessment of the levels of reactive molecule malondialdehyde formed during the LPO chain reaction. Numerous studies have shown reduction in rates of LPO in the tumor tissue of various types of cancers (Tanaka et al., Citation1998; Pillai et al., Citation1999). Acquisition of particular characteristics by cancer cells has benefitted their proliferation (Schmelz et al., Citation2000). Also, they tend to proliferate faster when the LPO levels are low. Thus, malignant tissues are less prone and more resistant to free radical attack, and hence LPO is less intense (Nakagami et al., Citation1999). The observed attenuated levels of LPO by pioglitazone hydrochloride could be owed to the fact that it has a role in countering the cell proliferation, thereby, indirectly regulating the levels of LPO.
Effect on catalase and SOD
A statistically significant decrease in the activities of catalase and SOD was observed following DMH treatment (p < 0.05). However, PPARγ ligand, pioglitazone HCl, treatment to DMH-treated animals (Groups III and IV) resulted in significantly increased activity of catalase and SOD enzymes when compared with DMH-treated animals (Group II) (p < 0.05). There was no significant difference in effect of higher and lower dose of the drug on the activities of catalase and SOD.
The reduction in effects of oxidant molecules on tissues have been observed by the antioxidant enzymes, SOD and catalase, which are activated in the defense against oxidative cell injury by means of their being free radical scavengers (Kyle et al., Citation1987). These enzymes are effective to eliminate active oxygen species. The minute deviations in physiological concentrations may have a dramatic effect on the resistance of cellular lipids, proteins and DNA to oxidative damage (Mates & Sanchez-Jimenez, Citation1999). In our study, SOD and catalase activities were found to be significantly decreased following 16 weeks DMH treatment, when compared to the normal control animals. These results are due to a natural cellular response against the decreased levels of reactive oxygen species with regard to hydrogen peroxide and superoxide radicals.
Effect on reduced glutathione
The activity of GSH enzymes significantly increased (p < 0.001) in DMH-treated rats as compared to normal control animals. The PPARγ ligand administration to DMH-treated animals resulted in significant attenuation of GSH levels when compared to DMH-treated group (p < 0.05). The lower dose of the drug (1.5 mg/kg) was as effective as the higher dose (4.5 mg/kg) in reverting the activity of GSH enzymes near normal control levels.
Experimentally, it has been depicted that decreased LPO is associated with enhanced GSH in the colon and intestines (Pillai et al., Citation1999). In this study, enhanced GSH levels following 16 weeks of DMH treatment have been observed. This may be the reason for augmented cell proliferation involved in the pathogenesis of DMH-induced colon cancer (Cao et al., Citation1997). The treatment with pioglitazone hydrochloride to DMH-treated animals modulated the levels of GSH, thus attributing their protective effect in restoring GSH activity.
Histopathological studies
Tissue sections of Group I animals displayed normal colonic architecture with no signs of apparent abnormality (). The normal H/E stained sample of the colon showed clear goblet cells with regular crypts and submucosal layer. In the carcinogen-treated Group II, the outer submucosal and muscular mucosa seemed to be unaffected, however, the inner cells facing the lumen were found to be disrupted (). The histoarchitectural plan due to DMH treatment was well evident of differentiated signs of dysplasia.
Figure 3. Histopathological observations (H/E staining). (a) Normal colonic mucosa of the control (×40) (Group I), (b) normal colonic crypt structure (×100) (Group I), (c) occurrence of dysplasia (×40) in the DMH-treated animals (Group II), (d) disrupted histoarchitecture (×100) in the DMH-treated animals (Group II), (e) colonic epithelium of DMH + Pio1-treated animals showing restoration of normal histology (×100) (Group III), (f) colonic histoarchitecture of (×40) of the colons of the DMH + Pio2-treated animals depicting regressive effect of drug, (g) normal histoarchitecture (×100) of the colons of the DMH + Pio2-treated animals showing normal apical surface of the crypt (Group IV).
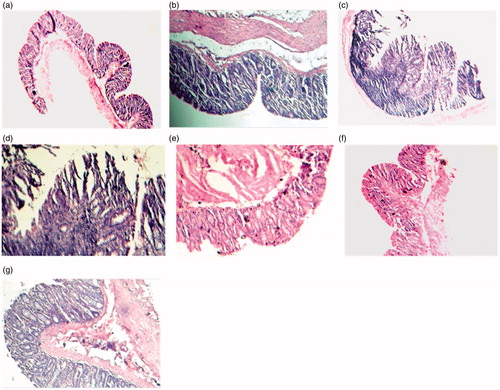
In the combined treatment Groups III and IV, reversal of the histoarchitecture of the colon in terms of epithelia and submucosal structures was indicative (). Since DMH (30 mg/kg body weight/week subcutaneously) and pioglitazone hydrochloride (1.5 and 4.5 mg/kg body weight peroral daily) were given simultaneously for a period of 16 weeks, it revealed the prophylactic effect of PPARγ ligand on the colon. Thus, the histopathological observations imply that pioglitazone hydrochloride is able to restore the histological changes induced by DMH.
This study thus clearly indicates that the administration of pioglitazone HCl in the presence of the procarcinogen DMH appreciably modulates the alterations in the tissue levels of LPO and the overall antioxidant enzymatic status. Furthermore, the histological findings clearly support these biochemical data and suggest that PPARγ ligand may play a promising anticancer role with respect to colon carcinogenesis. Therefore, pioglitazone hydrochloride was selected as the drug for the development of colon-targeted drug delivery system. As there was no added beneficial effect of higher dose of pioglitazone hydrochloride, the formulation development was done with lower dose.
Evaluation of matrix tablets
If a locally acting drug is orally administered using a conventional pharmaceutical dosage form, the latter rapidly disintegrates in the contents of the stomach and the drug is released and likely to be absorbed into the blood stream. This leads to elevated systemic drug concentrations and, thus, to an increased risk of undesired side effects. At the same time, low drug concentrations will be available at the desired site in the colon, resulting in poor therapeutic efficiency. These restrictions can be overcome if drug release is suppressed in the stomach and small intestine. Colon-targeted dosage forms allow the systemic delivery of drugs over a prolonged period, exploiting the reservoir function of the right colon, and enabling the delivery of drugs locally in high concentration for the treatment of diseases of the colon. Therefore, design of delayed-release systems for optimal drug delivery to the colon is beneficial.
Matrix tablets are one of the most widely used oral-delayed release systems containing a therapeutic agent, whereby the therapeutic agent is homogeneously dissolved or dispersed in a suitable compressible mixture of release retarding polymers. The design of these systems has been focused on the concept of polymeric hydration to protect the tablet from rapid disintegration and dissolution and to delay the drug release rate.
Release experiments
The goal of this study was to evaluate the effects of hydroxylpropyl methyl cellulose (HPMC) on the release behaviour of pioglitazone hydrochloride from a swellable matrix tablet system containing locust bean gum. In vitro studies were performed with the aim to select a proper combination of release retardant polymer, and to select an appropriate coat for the system. Locust bean gum has been widely used for colon targeting as it aids in degradation of the matrix on enzymatic degradation by colonic microflora, once the tablet reaches the colon. In this study, locust bean gum in the form of matrix former was evaluated as a suitable carrier for colonic drug delivery due to its specific degradation by enzymes secreted by microflora of colon.
Initially, three batches of locust bean gum in the proportion of 20%, 30% and 50% (LB20, LB30 and LB50, respectively) were prepared. The release profiles for batches LB30 and LB20 prepared with only locust bean gum released the whole drug, i.e. 100.42% and 99.57% within 2 h. Hence, these were not suitable for delaying the drug release.
Thus, inclusion of a suitable release retardant was necessary to delay the drug release. HPMC, a semi-synthetic cellulose derivative, is widely used as a matrix former in oral controlled release tablet formulations for targeting the drug release in the intestine. Its prevalent use is mainly due to its GRAS status and biodegradable nature (Ghimire et al., Citation2010). Many formulations containing HPMC as the release retarding agent have recently received FDA approval. It can be compressed directly into matrices, and is available in different viscosity grades. Therefore, HPMC K4M was employed to retard the release in the study. Another key factor affecting drug release from the HPMC matrices is the drug/polymer ratio (Velasco et al., Citation1999). Therefore, relatively lesser proportions of the polymer were used in the study (20–40%) for optimization of drug release owing to the poorly soluble nature of the drug.
In order to add a degradable fraction in the matrix system, higher proportion of locust bean gum will ensure complete drug release in the colonic region due to its degradation specifically in this segment of the GIT. It was observed that a further increase in the concentration of locust bean gum beyond 30% w/w leads to loss in direct compression properties of the blend. Therefore, varying proportions of HPMC K4M (20, 30, 40% w/w) were formulated with 30% w/w locust bean gum.
Dissolution profile of formulations LBH1, LBH2 and LBH3 is as shown in . The tablets of LBH1 containing 20% HPMC K4M showed a cumulative percent release of 34.837 ± 0.721 in first 5 h and was 79.328 ± 0.961% in 24 h. On further increasing the concentration of HPMC K4M in the matrix, initial retardation was further enhanced. The tablets of LBH2 (30% HPMC K4M) and LBH3 (40% HPMC K4M) depicted a cumulative percent release of 23.795 ± 1.209 and 24.535 ± 1.094, respectively, in first 5 h. At 24 h, 72.314 ± 0.955% and 63.531 ± 1.210% drug was released from LBH2 and LBH3, respectively.
Figure 4. Plot of cumulative percentage release profile from matrices containing a mixture of HPMC K4M:locust bean gum.
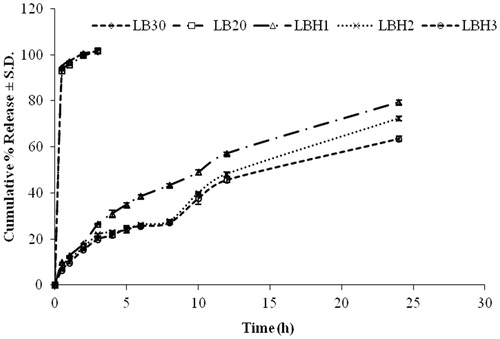
Matrices containing HPMC K4M tend to absorb water rapidly resulting in the formation of a tough gelatinous layer. The thickness and the strength of this gelatinous layer are very crucial parameters in controlling the rate of drug release from the developed matrices. Initial drug release is controlled by the concentration gradient of the drug across this layer. However, on complete hydration, this polymeric gelatinous layer starts eroding due to the disentanglement of the polymeric chains.
Apparently, these matrices formulated in HPMC K4M:locust bean gum could sustain pioglitazone hydrochloride release. Despite the initial retardation, the complete drug could not be released from these formulations. However, complete drug release is expected in the presence of the colonic content due to the presence of locust bean gum in the tablets. The combination of locust bean gum 30% and HPMC K4M 30% (LBH2) was the most apt choice for the development of the desired matrix system but enteric coating is required to prevent initial 5 h drug release to maximize the amount of drug released in the colon.
Enteric coating of matrix tablets
In the ensuing study, the purpose of the enteric coat was to considerably delay the release of pioglitazone hydrochloride in the stomach, and on dissolution of the enteric layer, the drug release will be delayed in the small intestine by HPMC K4M and the gum. The local administration of pioglitazone hydrochloride to the colonic tissue in this fashion aids in prophylactic effect on CRC. Eudragit®S100 was used for enteric coating. Cumulative amount of drug obtained after 24 h from both the uncoated (LBH2) and coated batches (LBH2A, LBH2B, LBH2C, LBH2D and LBH2E) is shown in .
Figure 5. Plot of cumulative percentage release profile from matrices containing a mixture of HPMC K4M:locust bean gum uncoated and coated at various levels with Eudragit®S100 (6% w/w).
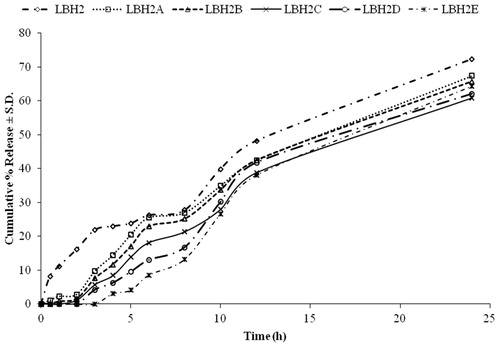
The tablets of batch LBH2 containing 30% HPMC K4M and 30% locust bean gum showed a cumulative percent release of 23.795 ± 1.209 in first 5 h and was 72.314 ± 0.955% in 24 h. Batch LBH2A showed a release of 20.424 ± 0.709% after 5 h and 67.397 ± 1.073% at the completion of 24 h. On further increasing the coat weight on the core, more retardation of drug release was achieved. Batch LBH2E was observed to be the most suitable for delaying the drug release to a sufficient extent in the upper segment of the GIT. It showed drug release of 4.116 ± 0.465% at 5 h and 64.341 ± 0.544% after 24 h. Thus, drug release of 60.225% occurred in the interval of 5–24 h. Although 100% of drug release is not observed in the following study, complete drug release is proposed in the presence of rat caecal content. The presence of colonic microflora would aid in degradation of the matrix once the system reaches the colon, and thus assist in complete drug release.
It was observed that the drug release from the formulations decreased with the increase in the coat weight. The percent release after 5 h followed the following order LBH2 > LBH2A > LBH2B > LBH2C > LBH2D > LBH2E. The release of drug from the drug–polymer matrix takes place after removal of the enteric coat in the basic microenvironment of the colon. However, the time required for the removal of the coat is directly proportional to the coat thickness. The release from the batch LBH2E was considered to be appropriate as it gave the required lag phase of 5 h (<5% of drug release was observed) followed by sufficient drug release after 24 h, i.e. 64.341%. The complete release of drug was further tested in vitro in the presence of rat caecal content.
Release experiments in presence of rat caecal content
The potential use of locust bean gum as a polymer for colon-specific dosage forms, due to its biodegradability with colonic enzymes has been investigated. However, no reports were seen for the study of release of these matrices in the presence of a release retardant like HPMC. In order to study the susceptibility of the prepared enteric-coated matrix system (LBH2E) to enzymatic degradation in the presence of colonic bacteria, drug release studies were performed in a similar kind of biphasic medium in the presence of 2% w/w caecal content for 19 h after 5 h of dissolution in simulated gastric fluid.
All the samples were analyzed using a validated RP-HPLC method for the drug. Cumulative percent release observed after 24 h increased from 67.970 ± 1.012% to 97.318 ± 2.613% in the presence of 2% w/v caecal content (). In vitro studies on locust bean gum tablets in simulated gastric, intestinal and colonic fluids have shown that locust bean gum, in the form of a matrix tablet along with a rate controlling polymer, is capable of protecting the drug from being released completely in the physiological environment of stomach and small intestine and is susceptible to the enzymatic action of the colonic bacteria. Based on these results, it was observed that enteric-coated matrix tablets composed of 30% HPMC K4M and 30% locust bean gum can be successfully used for carrying potent chemopreventive agents like PPARγ ligands, pioglitazone hydrochloride, specifically to the site of action in case of colon cancer. Thus, batch LBH2E was concluded to be the best formulation as it can ensure the delivery of maximum amount of drug to the colon.
Statistical analysis
The cumulative percentage of pioglitazone hydrochloride release from the enteric-coated matrices (LBH2E) n = 3 in the dissolution media at 24 h with and without rat caecal content was compared, and statistical significance was tested using one-way ANOVA followed by Tukey test. A value of p < 0.001 obtained was considered statistically significant.
Conclusions
The role of pioglitazone hydrochloride as a potential anticancer agent was evaluated by in vitro cell line studies and in vivo DMH-induced colon carcinogenesis in rats. The % IR of pioglitazone hydrochloride in HCT-116 cells increased from 2.96 ± 0.477 to 15.374 ± 0.552% with increase in concentration from 1 to 100 µmol/L. The administration of pioglitazone HCl in the presence of the procarcinogen DMH appreciably attenuated the alterations in the tissue levels of LPO and the overall antioxidant enzymatic status well supported by the histological findings. As there was no added beneficial effect of higher dose of pioglitazone hydrochloride, the formulation development was carried out with lower dose.
Batch LBH2E was observed to be the most suitable for delaying the drug release to a sufficient extent in the upper segment of the GIT. It showed drug release of 4.116 ± 0.465% at 5 h and 64.341 ± 0.544% after 24 h. The complete drug could not be released from these formulations. However, in the presence of 2% w/v rat caecal content cumulative percent release observed after 24 h increased significantly from 67.970 ± 1.012% to 97.318 ± 2.613%. Thus, batch LBH2E was found to be appropriate to ensure the delivery of maximum amount of drug to the colon.
The studies led to the conclusion that pioglitazone hydrochloride has the chemopreventive potential in CRC as deciphered by in vitro cell line studies and in vivo DMH-induced colon carcinogenesis in rats. Biodegradable polysaccharide matrices were successfully developed to deliver the drug to colon and improve its therapeutic efficacy in the management of CRC.
Declaration of interest
The authors report no conflicts of interest.
References
- Brockman JA, Gupta RA, Dubois RN. (1998). Activation of PPAR [gamma] leads to inhibition of anchorage-independent growth of human colorectal cancer cells. Gastroenterology 115:1049–55
- Cao G, Sofic E, Prior RL. (1997). Antioxidant and prooxidant behavior of flavonoids: structure-activity relationships. Free Radic Biol Med 22:749–60
- Dani V, Goel A, Vaiphei K, et al. (2007). Chemopreventive potential of zinc in experimentally induced colon carcinogenesis. Toxicol Lett 171:10–18
- Ellman GL. (1959). Tissue sulfhydryl groups. Arch Biochem Biophys 82:70–7
- Ferlay J, Shin HR, Bray F, et al. (2010). Estimates of worldwide burden of cancer in 2008: GLOBOCAN 2008. Int J Cancer 127:2893–917
- Friend DR. (2005). New oral delivery systems for treatment of inflammatory bowel disease. Adv Drug Deliv Rev 57:247–65
- Ghimire M, Hodges LA, Band J, et al. (2010). In-vitro and in-vivo erosion profiles of hydroxypropylmethylcellulose (HPMC) matrix tablets. J Control Release 147:70–5
- Jemal A, Bray F, Center MM, et al. (2000). Global cancer statistics. CA Cancer J Clin 61:69–90
- Kato M, Kusumi T, Tsuchida S, et al. (2004). Induction of differentiation and peroxisome proliferator-activated receptor gamma expression in colon cancer cell lines by troglitazone. J Cancer Res Clin Oncol 130:73–9
- Kono Y. (1978). Generation of superoxide radical during autoxidation of hydroxylamine and an assay for superoxide dismutase. Arch Biochem Biophys 186:189–95
- Kota BP, Huang TH, Roufogalis BD. (2005). An overview on biological mechanisms of PPARs. Pharmacol Res 51:85–94
- Krishnan K, Ruffin MT. (2000). Chemoprevention for colorectal cancer. Crit Rev Oncol Hematol 33:199–219
- Kyle ME, Miccadei S, Nakae D, et al. (1987). Superoxide dismutase and catalase protect cultured hepatocytes from the cytotoxicity of acetaminophen. Biochem Biophys Res Commun 149:889–96
- Laxmi G, Mukhopadhyay S, Durgapal S. (2010). Simultaneous estimation of metformin and pioglitazone by ultraviolet spectrophotometry. Indian J Pharm Sci 72:508–10
- Lefebvre AM, Chen I, Desreumaux P, et al. (1998). Activation of the peroxisome proliferator-activated receptor promotes the development of colon tumors in C57BL/6J-APCMin/+ mice. Nat Med 4:1053–7
- Luck H. (1954). Quantitative determination of catalase activity of biological material]. Enzymologia 17:31–40
- Mates JM, Sanchez-Jimenez F. (1999). Antioxidant enzymes and their implications in pathophysiologic processes. Front Biosci 4:D339–45
- Nakagami K, Uchida T, Ohwada S, et al. (1999). Increased choline kinase activity in 1,2-dimethylhydrazine-induced rat colon cancer. Jpn J Cancer Res 90:1212–17
- Pillai MG, Thampi BS, Menon VP, et al. (1999). Influence of dietary fiber from coconut kernel (Cocos nucifera) on the 1, 2-dimethylhydrazine-induced lipid peroxidation in rats. J Nutr Biochem 10:555–60
- Sarraf P, Mueller E, Jones D, et al. (1998). Differentiation and reversal of malignant changes in colon cancer through PPARγ. Nat Med 4:1046–52
- Schmelz EM, Sullards MC, Dillehay DL, et al. (2000). Colonic cell proliferation and aberrant crypt foci formation are inhibited by dairy glycosphingolipids in 1, 2-dimethylhydrazine-treated CF1 mice. J Nutr 130:522–7
- Shen D, Deng C, Zhang M. (2007). Peroxisome proliferator-activated receptor agonists inhibit the proliferation and invasion of human colon cancer cells. Postgrad Med J 83:414–19
- Shimada T, Kojima K, Yoshiura K, et al. (2002). Characteristics of the peroxisome proliferator activated receptor (PPAR) ligand induced apoptosis in colon cancer cells. Gut 50:658–64
- Sinha VR, Mittal BR, Bhutani KK, et al. (2004). Colonic drug delivery of 5-fluorouracil: an in vitro evaluation. Int J Pharm 269:101–8
- Takahashi H, Hosono K, Uchiyama T, et al. (2010). PPAR ligand as a promising candidate for colorectal cancer chemoprevention: a pilot study. PPAR Res 2010:pii257835
- Tan MH, Johns D, Strand J, et al. (2004). Sustained effects of pioglitazone vs. glibenclamide on insulin sensitivity, glycaemic control, and lipid profiles in patients with Type 2 diabetes. Diabetic Med 21:859–66
- Tanaka T, Kawabata K, Kakumoto M, et al. (1998). Citrus auraptene exerts dose-dependent chemopreventive activity in rat large bowel tumorigenesis: the inhibition correlates with suppression of cell proliferation and lipid peroxidation and with induction of phase II drug-metabolizing enzymes. Cancer Res 58:2550–6
- Velasco M, Ford JL, Rowe P, et al. (1999). Influence of drug: hydroxypropylmethylcellulose ratio, drug and polymer particle size and compression force on the release of diclofenac sodium from HPMC tablets. J Control Release 57:75–85
- Wills ED. (1966). Mechanisms of lipid peroxide formation in animal tissues. Biochem J 99:667–76