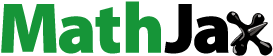
Abstract
Context: The study was aimed to develop a polymeric nanoparticle formulation of anticancer drug carboplatin using biodegradable polymer polycaprolactone (PCL). The formulation is intended for intranasal administration to treat glioma anticipating improved brain delivery as nasal route possess direct access to brain and nanoparticles have small size to overcome the mucosal and blood–brain barrier.
Objective: Development and evaluation of carboplatin-PCL nanoparticles for brain delivery by nasal route.
Methodology: Carboplatin-loaded PCL nanoparticles (CPCs) were prepared by double emulsion-solvent evaporation technique and characterized by particle size, zeta potential, entrapment efficiency, scanning electron microscopy and differential scanning calorimetry. The CPCs were assessed for in vitro release kinetics, ex vivo permeation and in situ nasal perfusion. Cytotoxic potential of CPCs in vitro was evaluated on LN229 human glioblastoma cells.
Results and discussion: The optimized formulation of carboplatin-PCL nanoparticle CPC-08 with particle size of 311.6 ± 4.7 nm and zeta potential −16.3 ± 3.7 mV exhibited percentage entrapment efficiency of 27.95 ± 4.21. In vitro drug release showed initial burst release followed by slow and continues release indicating biphasic pattern. The ex vivo permeation pattern through sheep nasal mucosa also exhibited a similar release pattern as for in vitro release studies. In situ nasal perfusion studies in Wistar rats demonstrate that CPCs show better nasal absorption than carboplatin solution. In vitro cytotoxicity studies on LN229 cells showed an enhancement in cytotoxicity by CPCs compared to carboplatin alone.
Conclusion: CPC-08 effectively improves nasal absorption of carboplatin and can be used for intranasal administration of carboplatin for improved brain delivery.
Introduction
Delivery of therapeutics to the central nervous system (CNS) has always been a challenge due to the presence of blood–brain barrier (BBB) and blood–cerebrospinal fluid barrier. Many novel drug molecules have failed to reach CNS at therapeutically effective dose due to their high molecular weight and low lipophilicity. Development of a formulation whose particle size is in nanometer range and use of biodegradable polymers which are lipophilic in nature can solve this issue. Apart from this, intranasal route is an attractive non-invasive route that can offer benefits such as ease of administration, rapid absorption, avoidance of liver and gut first-pass metabolism, and self-medication (Behl et al., Citation1998). It is also well-established that there exists a direct anatomical connection between the nasal cavity and the CNS by paracellular and transcellular pathway as well as through trigeminal neurons which suggest the scope of the development of CNS therapeutics for intranasal administration. Drugs such as sulfonamides (Sakane et al., Citation1991a), cephalexin (Sakane et al., Citation1991b), progesterone (Anand Kumar et al., Citation1982) and zidovudine (Seki et al., Citation1994) are reported to be successfully delivered to brain by intranasal route. Drugs presently in market such as estradiol for hormone replacement therapy, nicotine for smoking cessation, cyanocobalamin to treat vitamin B12 deficiency, zolmitriptan and sumatriptan for migraine and cluster headache, buserelin for the treatment of prostate cancer, are other examples of intranasally administered drugs for systemic effect. Intranasal route as a successful strategy to deliver drugs to CNS for the treatment of various diseases such as Alzheimer's disease, epilepsy, pain, sleep disorders, are also reported (Deepti et al., Citation2014; Pires et al., Citation2009). Hence, this route could be convincing for the treatment of brain tumor where the nose to brain pathway might provide a faster and specific therapeutic effect (Illum, Citation2002).
Carboplatin is a documented anti-neoplastic drug with proven anticancer activity. Many anti-neoplastic drugs with established therapeutic efficacy toward a particular cancer cause objectionable toxicological concerns when introduced systemically due to non-specific biodistribution, the absence of site specific affinity of the drug toward a pathological site which necessitates high dose of the drug to attain desired concentration at the target site, resulting in non-specific toxicity and other side effects. A novel approach to overcome these limitations is to develop new dosage forms which are capable of delivering the therapeutic agent to target site and sustains the release of drug for prolonged effect at a therapeutically optimum rate and dose schedule.
Nanoparticles are designed for their benefit of possessing nano-size range of particles which can infiltrate through the sites of inflammation and cancer cells and are mostly taken up by the cells leading to effective drug accumulation at the target sites (Panyam & Labhasetwar, Citation2003). Enhanced permeation and retention (EPR) effect also permits the passive targeting of drugs encapsulated in nanoparticles to the tumor site.
Hence, in the present study, the advantages of intranasal route as a promising strategy for delivering therapeutics to brain using nanoparticulate drug delivery systems of anticancer drug carboplatin is utilized. This study explains the development of polymeric nanoparticles of carboplatin using polycaprolactone (PCL). PCL is a biodegradable and biocompatible polymer which controls the release of drug by extending mean residence time, thus enhancing anti-tumor activity. The formulation is designed for intranasal administration, which can result in improved brain delivery due to the dual advantage of enhanced nasal absorption because of small particle size as well as a direct route to access brain. It also minimizes the toxicity by reducing random biodistribution of the drug to healthy tissue compared to pure drug. Carboplatin was encapsulated within PCL by double emulsion-solvent evaporation method using polyvinyl alcohol (PVA) as emulsifying agent and mannitol was used as cryoprotectant during freeze drying of nanoparticles. The nanoparticles were characterized based on size, surface charge and in vitro drug release profile. In situ nasal perfusion studies were performed using Wistar rats to demonstrate nasal absorption and ex vivo permeation studies using sheep nasal mucosa to study the mucosal permeation of carboplatin from the optimized formulation. In vitro anti-tumor activity of carboplatin-loaded nanoparticles were assessed on human brain tumor cells.
Materials and methods
Materials
Carboplatin was purchased from AK Scientific Inc. (Union City, CA). Polymer poly-ε-caprolactone (PCL) (molecular weight 65 000 g/mol), PVA (molecular weight 30 000–70 000) and MTT reagent were purchased from Sigma Aldrich (St. Louis, MO). LN229 cell line was purchased from National Centre for Cell Science (NCCS) (Pune, India).
Preparation of carboplatin-PCL nanoparticles by double emulsion-solvent evaporation technique
Polymeric nanoparticles of carboplatin with PCL were prepared by double emulsion method followed by solvent evaporation resulting in a water/oil/water (w/o/w) emulsion (Davda et al., Citation2002) using different drug to PCL ratios (1:1, 1:5, 1:10, 1:15, 1:30 and 1:60). In this method, carboplatin (5 mg) dissolved in water was homogenized with polymer dissolved in dichloromethane (DCM) (5 ml) at 15 000 rpm using high-speed homogenizer (Polytron PT-3100; Kinematica AG, Lucerne, Switzerland) for 15 min in an ice-water bath. The resulting primary emulsion was further size reduced to form nanoparticles by adding it dropwise to the aqueous phase containing 2.0% w/v PVA solution (15 ml), while being homogenized using high-speed homogenizer at 20 000 rpm followed by probe sonication at 80% amplitude for 8 min. During this procedure, the sample is placed in an ice bath. Complete evaporation of DCM from the emulsion (w/o/w) was effected by stirring for up to 4 h. Nanoparticles were separated by subjecting the suspension for centrifugation at 20 000 rpm for 30 min, the pellet obtained was re-dispersed in mannitol solution (5% w/v) as cryoprotectant and freeze dried for 48 h at a temperature between −40 and −60 °C and vacuum <50 mTorr to obtain dry free-flowing mass. The effect of various experimental parameters such as polymer-to-drug ratio, type of stabilizer and its concentration, homogenization speed, sonication conditions, type of cryoprotectant and its concentration on physicochemical properties of nanoparticles were also studied.
Physicochemical characterization of nanoparticles
Estimation of carboplatin in nanoparticles by high-performance liquid chromatography
Carboplatin in nanoparticles was estimated by a specific and sensitive reverse-phase chromatographic method. The analysis was performed using integrated high-performance liquid chromatography (HPLC) system, Shimadzu LC-2010 model, with dual wavelength UV spectrophotometer detector (Shimadzu Corporation, Kyoto, Japan). LC solution version1.24 SP1 (Shimadzu Corporation, Kyoto, Japan) was used to process the data obtained from HPLC. The mobile phase consists of a mixture of sulfuric acid 0.001 N and sodium sulfate 0.02 M as aqueous phase and methanol as the organic phase (90:10). Hypersil C18 ODS column (250 × 4.6 mm, 5 μm) was stationary phase, maintained at 25 °C with a flow rate of 1 ml/min at a detection wavelength of 229 nm. Briefly, a small quantity (10 mg) of lyophilized nanoparticles was suspended in 1 ml of DCM followed by mixing thoroughly for 10 min, solvent was removed by passing nitrogen gas and the residue so-obtained was re-dispersed in 1 ml of mobile-phase mixture. The sample was vortexed for 5 min, centrifuged at 10 000 rpm for 10 min and supernatant was used for analysis. A representative chromatogram is shown in . Drug entrapment efficiency (%) was calculated (n = 3) according to Equation (1) (Fonseca et al., Citation2002).
(1)
(1)
Particle size and zeta potential
The nanoparticles of carboplatin were analyzed for its particle size, polydispersity index (PDI) and zeta potential using Zetasizer (Nano ZS, Malvern Instruments, Malvern, UK). The particle size was measured using dynamic light scattering technique (DLS), which measures the diffusion of particles moving under Brownian motion. These measurements are converted to size and a size distribution by applying the Stokes–Einstein relationship. Simultaneously, PDI which is a measure of particle homogeneity in dispersion was also measured. The zeta potential of a particle is the total charge acquired by it in a specific medium. This technique measures the electrophoretic mobility exhibited by a charged particle as it moves with a velocity, in a dispersion medium connected with a pair of electrodes. When a voltage is applied, this particle moves with a velocity toward the oppositely charged electrodes. Samples were diluted in Milli-Q water (Merck Millipore, Milli-Q Gradient, MA, USA) and the analysis was carried out in triplicates.
Thermogram properties
The thermal behavior of pure drug, pure PCL polymer, PVA, mannitol and freeze-dried optimized CPC formulation was studied by differential scanning calorimetry, using differential scanning calorimetry (DSC)-60 instrument (Shimadzu Corporation, Kyoto, Japan). The samples were heated at a rate of 5 °C/min from 25 to 300 °C under nitrogen flow of 30 ml/min (Musumeci et al., Citation2006).
Scanning electron microscopy
Scanning electron microscopy (SEM) (Zeiss Smart SEM EVO-18, Oberkochen, Germany) was used for surface morphological characterization of carboplatin-loaded PCL nanoparticles. The water suspended nanoparticles were mounted on a glass slide as a thin smear and left to dry. The particles on the dried glass slide were subjected to gold sputtering and the slide was attached on SEM holder using a double-sided carbon tape mounted on aluminum stud. The photomicrographs were captured by operating at an accelerating voltage of 20 kV electron beam at desired magnification.
In vitro drug release studies
In vitro drug release from carboplatin-PCL nanoparticles was performed by dialysis tube method as reported by Jain et al. (Citation2009). The dispersion of carboplatin-PCL nanoparticles in 3 ml of simulated nasal fluid equivalent to 2 mg of carboplatin was placed in dialysis tube (cutoff 12 000 Da; HiMedia Laboratories Pvt Ltd, Mumbai, Maharashtra, India). The dialysis tube was immersed in the receptor compartment containing 100 ml of phosphate buffer pH 6.6, which is within the pH range of nasal cavity. The receptor compartment solution was stirred at 100 rpm and temperature was maintained at 37 ± 2 °C. Samples were collected at regular intervals, and replaced with an equal volume of fresh release medium. The samples withdrawn were centrifuged at 5000 rpm for 2 min, supernatant was passed through 0.45-μm membrane filter and the filtrate was injected into HPLC system with UV detection at 229 nm. The amount of carboplatin released in each sample was estimated from a calibration curve (n = 3). Results of in vitro drug release studies obtained are represented graphically as cumulative percentage drug release versus time.
To understand the drug release kinetics, the data obtained from in vitro drug release study were fitted to various kinetic models such as zero order (cumulative amount of drug released versus time), first order (log cumulative percentage of drug remaining versus time) and Higuchi model (cumulative percentage of drug released versus square root of time).
To evaluate the drug release mechanism from the CPCs, data obtained from the in vitro drug release studies were subjected to the Korsmeyer–Peppas model [Equation (2)]. The release exponent n and K values were calculated from the slope of straight line for the plot of log cumulative percentage of drug released versus log time.
(2)
(2)
where K is the diffusional constant of the drug polymer system, n is an exponent that characterizes the mechanism of drug release, M is the total amount of drug released after an infinite time and Mt represents the amount of the released drug at time t. The drug release mechanism of a delivery system is indicated based on the value of release exponent (n) (Jain et al., Citation2009; Kulkarni et al., Citation2011).
Ex vivo permeation studies of carboplatin-PCL nanoparticles through sheep nasal mucosa
In order to predict the real drug release characteristics, nasal mucosa of sheep was selected for ex vivo permeation studies. Nasal mucosa of sheep was collected from local slaughter house within 1 h of sacrificing the animal and was cleaned thoroughly using isotonic saline solution. A portion of nasal mucosa was carefully cut using a scalpel and mounted on a Franz diffusion chamber with the mucosal side facing the donor and serosal side facing the receptor compartments. The donor compartment containing the dispersion of carboplatin-PCL nanoparticles equivalent to 2 mg of carboplatin in 3 ml of simulated nasal fluid, was kept in contact with the diffusion medium in the receptor chamber containing phosphate buffer pH 6.6, that was within the pH range of nasal cavity and maintained at 37 ± 2 °C (Tas et al., Citation2006). Samples were collected at regular intervals, and replaced with an equal volume of fresh release medium. The analyses of samples were performed in the same manner as in vitro release studies.
Histopathological studies
Histopathological studies were performed on sheep nasal mucosa to assess any damage on integrity of nasal mucosal tissues after permeation experiments. After the permeation experiments, samples of mucosa were removed from diffusion chamber and rinsed with isotonic saline. Mucosa samples were fixed in 10% neutral carbonate-buffered formalin solution routinely processed and mounted in paraffin. Hematoxylin–eosin (HE)-stained paraffin sections were examined under light microscope, to detect any damage to the tissues (Mahajan et al., Citation2012). The non-permeated mucosa, fixated directly after isolation, was used as a control.
Nasal absorption studies of carboplatin-PCL nanoparticles by in situ nasal perfusion in male Wistar rats
The in situ nasal perfusion studies was performed on male Wistar rats (IAEC No.: IAEC/KMC/07/2008-2009) according to the technique developed by Hirai and Yashiki (Citation1981) and Huang et al. (Citation1985). An intraperitoneal injection of ketamine (50 mg/kg body weight) was used to anesthetize male Wistar rats weighing 250–350 g. Trachea and esophagus were exposed and the trachea was cannulated with a polyethylene 200 (PE-200) tube to aid breathing as perfusion procedure used regions between trachea and nostrils and mouth was blocked at the nasopalatine to prevent the loss of the perfusate through mouth. Carboplatin (control) solution or CPCs equivalent to 5 mg of carboplatin in isotonic phosphate-buffered saline pH 6.8 was circulated with the help of peristaltic pump at a flow rate of 2 ml/min. The perfusion sample (20 ml) was kept stirred and was placed in a water jacketed beaker with temperature maintained at 37 °C. Perfusate passing out of the nose was recollected into the same beaker using a funnel. The beaker containing perfusate was periodically sampled to measure the decreasing analyte concentration with time. About 100 μl of the perfusate was withdrawn periodically which was replaced with fresh buffer (100 μl) and was analyzed for the drug concentration in solution. The experiment was run for 75 min and samples were analyzed by HPLC for estimating the drug remaining in the solution at specific time point.
In vitro cytotoxicity studies
In vitro cytotoxicity of optimized CPCs was evaluated by MTT assay. 96-Well plates were seeded with exponentially growing human glioblastoma cells LN229 (104 cells/well, in 100 µl of medium) and incubated in a 5% CO2 atmosphere for 24 h to attain 60–80% confluence. Various concentrations equivalent to 24, 12, 6, 3, 1.5 and 0.75 µg/ml of carboplatin was added in a volume of 100 µl/well. Carboplatin solution was also added at similar concentrations whereas control wells were added with medium alone. After 48/96-h incubation, medium was flicked off, 100 µl of 1 mg/ml MTT reagent was added to each well and incubated for 4 h at 37 °C. The viable cells developed a purple-colored formazan complex which was solubilized by adding 100 µl of DMSO and mixed well with the help of a microvibrator. The absorbance was recorded on BioTek EL × 800's MS microtiter plate reader (BioTek, VT, USA) at 540 nm (Averineni et al., Citation2012). The percentage cell viability was calculated according to Equation (3) where cell survival in control wells was assumed to be 100%.
(3)
(3)
Statistical analysis
The data obtained from multi-replicated determinations were reported as mean ± standard deviation (SD) (n = 3). The data were compared using one-way analysis of variance (ANOVA) followed by Tukey's post hoc test using GraphPad Prism 5.0 (GraphPad Software, Inc, CA, USA), and level of significance was selected as p < 0.05.
Accelerated stability studies
Accelerated stability study was carried out as per the International Conference on Harmonization (ICH) Q1A (R2) guidelines. It was performed at 25 ± 2 °C/60 ± 5% RH for 3 months using a stability chamber (Thermolab Scientific Equipments Pvt Ltd, Mumbai, India). Freshly prepared freeze-dried nanoparticle powder of optimized batch was filled in three different amber-colored glass vials, sealed and placed in stability chamber and maintained at 25 ± 2 °C/60 ± 5% RH for a period of total 3 months. At a sampling interval of 1 month, the samples were re-dispersed in distilled water and analyzed for physical appearance, particle size, zeta potential and percentage entrapment efficiency.
Results and discussion
Formulation development and optimization
Based on the results of solubility studies of drug and polymer in various aqueous and organic solvents, carboplatin was found to be soluble in water and PCL polymer was soluble in DCM. Stabilizers are used in formulation of nanoparticles so as to provide stability of the dispersion in aqueous medium (). Various surfactants like PVA and pluronic F-68 were tried to study the influence of type of surfactant on physicochemical properties of carboplatin nanoparticles. PVA was selected as the stabilizer as it gave lower particle size and maximum entrapment efficiency without particle aggregation. Effect of PVA on particle size was tested at concentrations between 0.25% and 2.5% w/v. An increase in the particle size but decrease in entrapment efficiency was observed at higher concentrations of PVA (2.5% w/v) which may probably be due to an increase in aqueous solubility of the drug (Joshi et al., Citation2010). Inadequate amount of stabilizer failed to stabilize the nanoparticles and resulted in aggregation leading to the formation of large-sized particles while optimum concentration of stabilizer led to the formation of nanoparticles with small size (Feng et al., Citation2001; Movva et al., Citation2008). Hence, 2.0% w/v PVA concentration was considered optimum. The effect of drug:polymer ratio on entrapment efficiency and particle size was studied at different drug:polymer ratios like 1:1, 1:5, 1:10, 1:15, 1:30 and 1:60. An increase in drug:polymer ratio from 1:1 to 1:15 caused an increase in entrapment efficiency and decrease in particle size. Nevertheless, further increase in the drug:polymer ratio increased the particle size (Budhian et al., Citation2007; Shah et al., Citation2009). This may be due to the fact that an increase in the polymer concentration increased the viscosity of the organic phase leading to rapid diffusion of the organic phase into the aqueous phase. Higher polymer concentrations resulted in the formation of coarse dispersions and higher particle size, probably due to the insufficient amount of stabilizer present in the aqueous phase. Decrease in particle size was observed with increase in homogenization speed and sonication amplitude. However, increase in homogenization speed above 20 000 rpm and sonication amplitude >80% does not show any decrease in particle size. Various cryoprotectants such as sucrose, fructose, dextrose and mannitol with suitable concentrations were tested for their effect on freeze drying process of carboplatin polymeric nanoparticles. Among them mannitol yielded completely dry and free-flowing powders with lowest particle size. The concentration of mannitol was varied at different levels such as 2.5, 5 and 10% w/v to study their influence on physicochemical properties of nanoparticles. About 2.5% w/v mannitol produced a particle size of 751 nm with slight broad range of particles. However, lower particle size was observed with 5 and 10% w/v mannitol displaying a particle size distribution in the range of 310–515 nm. Therefore, 5% w/v mannitol was found to be sufficient to obtain nanoparticles of desirable properties. The CPCs prepared using optimized parameters had a percentage entrapment efficiency of 27.95 ± 4.21% and particle size of 311.6 ± 4.7 nm, with a PDI and zeta potential of 0.21 ± 0.03 and −16.3 ± 3.7 mV, respectively.
Table 1. Formulation of carboplatin-PCL nanoparticles.
Entrapment efficiency
To attain optimum entrapment of drug, various batches were prepared with different ratios of polymer to drug. Drug to polymer ratio of 1:15 showed an optimum entrapment efficiency of 27.95 ± 4.21%. The entrapment efficiency of all designed batches of carboplatin-PCL nanoparticles are reported in . As the drug:polymer ratio increased from 1:1 to 1:60, entrapment efficiency increased but particle size also increased beyond drug:polymer ratio of 1:15. But further increase in drug:polymer ratio decreased the drug entrapment in nanoparticle and increased the particle size.
Particle size, PDI and zeta potential
Average particle size, PDI and zeta potential of all batches of CPCs are reported in . The PDI was measured to determine the range of particle size distribution. Particle size distribution of optimized batch CPC-08 is depicted in . The PDI value for CPC-08 was found to be 0.21 ± 0.03. Pattern of size distribution of nanoparticles plays a vital role in defining the mucosal permeation, drug release behavior and their prospect for intranasal administration. Smaller-sized particles are benefited by EPR effect as they tend to accumulate in the tumor sites. Particles <1 μm are capable of passing through the nasal cavities with the inspired air, whereas particles >10 μm gets deposited at the anterior parts of the nose and thus evades ciliated absorption areas (Illum et al., Citation1987). The zeta potential for optimized formulation CPC-08 was −16.3 ± 3.7 mV, which was due to the negative charge of PCL. Zeta potential values in the range −15 to −30 mV are ideal for stabilized nanoparticles (Musumeci et al., Citation2006). The electrostatic repulsion between particles with high negative zeta potential values are more which prevents their aggregation and thereby stabilize the nanoparticulate dispersion (Feng et al., Citation2001). The optimized formulations (CPC-07 and CPC-08) with desirable properties were further used for in situ nasal perfusion studies.
Thermogram properties
DSC thermograms of PCL polymer (PC), free carboplatin (C), PVA (PV), mannitol, (MN) and carboplatin-loaded PCL nanoparticle (CPC-08) were analyzed to study the existence of interactions, if any, between the drug and excipients in the nanoparticles (). DSC thermogram of pure carboplatin exhibited endothermic thermal transitions at 235–245 °C which corresponds to its melting temperature (Tm) (Curve a). DSC thermogram of pure polymer PCL only showed an endothermic peak 71–72 °C which corresponds to its melting temperature (Curve c). The thermogram of carboplatin-loaded nanoparticles (CPC-08) exhibited endotherm at 63.84 °C which corresponds to melting endotherms of PCL polymer (Curve b). This thermogram also showed a broad and very weak endotherm between 235 and 245 °C corresponding to the melting endotherm of carboplatin. This may be due to the conversion of drug from crystalline to amorphous form. Therefore, it may be concluded that the carboplatin in PCL nanoparticles existed in amorphous form of a molecular dispersion or solid solution state in the polymer matrix. Thus, it could lead to increased solubility and finally to an improved biological activity.
SEM
The SEM photomicrograph of optimized carboplatin-PCL nanoparticles confirmed their spherical shape (). The particles exhibited moderate uniformity and all the particles were discrete entities without any aggregation. They were homogeneous with smooth surface and without any rupture.
In vitro release studies
In vitro release study was performed for all batches prepared. CPC-07 and CPC-08 were found to be the best batches and results are shown in . In vitro release profiles for all the formulations showed biphasic behavior consisting of initial burst release followed by a sustained release phase over a period of 72 h. The initial burst release of drug may be due to the release of carboplatin which was loosely bound or adsorbed on the surface of the nanoparticles. Sustained release may be due to slow diffusion of the drug from the highly lipophilic polymeric matrix. The release of entrapped carboplatin from nanoparticles with lower concentration of polymer was more rapid than those with higher polymer concentration. This could be due to the fact that increase in polymer concentration leads to the formation of thick and less porous polymer matrix.
Figure 5. In vitro percentage cumulative drug release profile of carboplatin-PCL nanoparticle formulations.
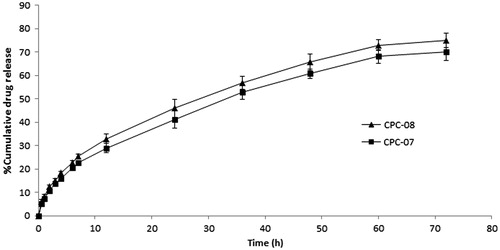
To study the drug release kinetics, the data obtained from in vitro drug release studies of optimized batch CPC-08 was fitted to the different models like, first order and Higuchi's model. Regression coefficients (R2) for first order and Higuchi's model were found to be 0.9854 and 0.9984, respectively. Thus, in vitro drug release of CPC-08 formulation was best explained by Higuchi kinetic diffusion control mechanism, as the plot showed the maximum linearity.
To study the mechanism of drug release, the data obtained from in vitro drug release studies were fitted to the Korsmeyer–Peppas model. The regression coefficient for the plot of log cumulative percentage drug release versus log time of the Korsmeyer–Peppas equation for carboplatin-PCL nanoparticle formulation CPC-08 was found to be 0.9981. The value of release exponent (n) was found to be 0.5321. As the n value is > 0.5, it can be concluded that the release of carboplatin from PCL nanoparticle was by non-Fickian diffusion (diffusion coupled with erosion) (Peppas, Citation1985).
Ex vivo permeation studies of carboplatin-PCL nanoparticles through sheep nasal mucosa
The drug permeation pattern through sheep nasal mucosa reflected a similar release pattern as that of in vitro release studies, but the amount of drug permeated was lower. This resistance to the permeation of drug molecules could be attributed to the complexity of nasal mucosa in terms of anatomy, chemical composition, etc. Between the two formulations (CPC-07 and CPC-08) selected for the study, formulation CPC-08 exhibited better permeation across the sheep nasal mucosa. The cumulative percentage of carboplatin permeated from CPC-08 after 8 h was found to be 22.91 ± 2.08%, upon three determinations. Permeation profile is shown in .
Histopathological studies
Histopathological studies were carried out to examine the histological changes in nasal mucosa caused by carboplatin-PCL nanoparticles. The cross section of the sheep nasal mucosa used for permeation before (untreated control, ) and after the permeation (treated) was stained by HE and observed under light microscope. Cross section of control nasal mucosa showed ciliated respiratory epithelium and normal goblet cells. The effect of carboplatin-PCL nanoparticles (CPC-08) on sheep nasal mucosa, 8 h after permeation studies (treated, ) showed no severe damage on the integrity of nasal mucosa when compared to untreated control. A slight epithelial disruption of nasal mucosa was observed.
Nasal absorption studies of carboplatin-PCL nanoparticles by in situ nasal perfusion in male Wistar rats
Nasal absorption of pure carboplatin solution and optimized carboplatin-loaded nanoparticle formulations (CPC-07 and CPC-08) were studied by in situ nasal perfusion in male Wistar rats. All the samples showed progressive nasal absorption with time. A plot of percentage drug remaining in the perfusing solution versus time is shown in . Carboplatin-loaded PCL nanoparticles exhibited better nasal absorption compared to pure carboplatin solution. Between the two formulations studied for nasal absorption, CPC-08 showed better nasal absorption profile compared to CPC-07. The first-order nasal absorption rate constants for carboplatin solution and optimized formulations CPC-07 and CPC-08 were calculated from the slopes of first-order plots of the percentage drug remaining in the perfusing solution versus time. The first-order nasal absorption rate constants for carboplatin solution, CPC-07 and CPC-08 were found to be 5.067 × 10−3, 6.218 × 10−3 and 6.909 × 10−3 min−1, respectively. There is a statistically significant (p < 0.05) increase in first-order nasal absorption rate constant for optimized carboplatin-PCL nanoparticles (CPC-07 and CPC-08) when compared to that of pure drug solution. Smaller particle size and bioadhesive nature of PCL polymer which enables it to bind to nasal mucosa has increased the residence time and subsequently increased the nasal absorption.
In vitro cytotoxicity studies by MTT assay
For in vitro cytotoxicity study, cells were incubated with various concentrations of both pure drug and CPCs for 48 and 96 h, separately. Percentage cell viability (y-axis) was plotted against concentration of the carboplatin (x-axis). Concentration of carboplatin that reduces the viability of cell to 50% (IC50 value) was determined for carboplatin and carboplatin nanoparticles after 48 and 96-h treatment. Results reveal a dose-dependent reduction in the percentage cell viability after treatment with carboplatin nanoparticle or carboplatin (). Pure carboplatin does not show any significant difference in cytotoxicity after 48 and 96 h of incubation. At shorter incubation time (48 h) cytotoxicity of carboplatin nanoparticles CPC-08 and CPC-07 were low at all tested concentrations compared to that of carboplatin solution. The IC50 value for CPC-08 and CPC-07 after 48 h incubation was found to be 10.80 ± 1.03 and 11.974 ± 1.15 μg/ml, respectively, while for pure carboplatin, the IC50 value was 6.738 ± 0.74 μg/ml. This may be due to the fact that polymeric nanoparticles of carboplatin only release ∼60% of drug from polymer matrix as indicated by in vitro release studies on CPCs. For longer incubations (96 h), carboplatin nanoparticle showed better cytotoxicity at all tested concentrations than that of pure carboplatin. The result suggests that polymeric nanoparticles release the drug slowly and have increased retention in the cells as compared to carboplatin alone. Thus, the IC50 value was significantly (p < 0.05) increased with carboplatin-loaded nanoparticles compared to pure carboplatin after 96 h of incubation, indicating enhanced anti-tumor activity of nanoparticles against human glioblastoma cells LN229 ().
Accelerated stability studies
Stability studies of optimized batch of freeze-dried carboplatin nanoparticle CPC-08 was carried out and stability of formulation was evaluated on the basis of particle size, zeta potential and entrapment efficiency as main parameters. Slight increase in average particle size and a slight decrease in zeta potential and percentage entrapment efficiency were observed on 3-month storage. As there is no significant alteration in average particle size, zeta potential and entrapment efficiency, it can be concluded that the carboplatin nanoparticle formulation CPC-08 is stable at 25 ± 2 °C/60 ± 5% RH for a total period of 3 months ().
Table 2. Accelerated stability studies of optimized batch of carboplatin-PCL nanoparticles.
Conclusion
In conclusion, the carboplatin-loaded PCL nanoparticles were successfully prepared with good entrapment, smaller particle size and uniform spherical shape. The optimized carboplatin-loaded PCL nanoparticles demonstrated sustained release and followed non-Fickian diffusion-based release kinetics. The drug permeation pattern through sheep nasal mucosa showed a similar release pattern as shown for in vitro release studies. Histopathological studies on sheep nasal mucosa after 8 h of permeation studies with carboplatin-PCL nanoparticles did not show any severe damage on the integrity of nasal mucosa. Further, the results of in situ nasal perfusion studies revealed that carboplatin-loaded PCL nanoparticles exhibited better nasal absorption compared to that of pure carboplatin solution. The developed nanoparticles of CPC have improved in vitro anti-tumor activity than that of plain drug against human glioblastoma cells LN229. Therefore, formulating carboplatin as polymeric nanoparticles is helpful to effectively improve the nasal absorption of carboplatin and can be used for intranasal administration of carboplatin for improved brain delivery.
Declaration of interest
The authors report no conflicts of interest. This research work is supported by research grant from Department of Biotechnology, Ministry of Science and Technology, Government of India (Project No. BT/PR10830/NNT/28/114/2008).
Acknowledgements
The authors would like to acknowledge the Department of Biotechnology, Ministry of Science and Technology, Government of India for providing the research grant to carry out the studies, Manipal College of Pharmaceutical Sciences, Manipal University, Manipal, India for providing the infrastructure facilities and Central Animal Facilities, KMC, Manipal University, Manipal, India for carrying out the in situ nasal perfusion studies.
References
- Anand Kumar TC, David GF, Sankaranarayanan A, et al. (1982). Pharmacokinetics of progesterone after its administration to ovariectomised rhesus monkeys by injection, infusion, or nasal spraying. Proc Natl Acad Sci USA 79:4185–9
- Averineni RK, Shavi GV, Gurram AK, et al. (2012). PLGA 50:50 nanoparticles of paclitaxel: development, in vitro anti-tumor activity in BT-549 cells and in vivo evaluation. Bull Mater Sci 35:319–26
- Behl CR, Pimplaskar HK, Sileno AP. (1998). Effects of physicochemical properties and other factors on systemic nasal drug delivery. Adv Drug Deliv Rev 29:89–116
- Budhian A, Siegel SJ, Winey KI. (2007). Haloperidol-loaded PLGA nanoparticles: systematic study of particle size and drug content. Int J Pharm 336:367–75
- Davda J, Labhasetwar V. (2002). Characterization of nanoparticle uptake by endothelial cells. Int J Pharm 233:51–9
- Deepti M, Asgar A, Shadab M, et al. (2014). Insights into direct nose to brain delivery: current status and future perspective. Drug Deliv 21:75–86
- Feng S, Huang G. (2001). Effects of emulsifiers on the controlled release of paclitaxel (Taxol) from nanospheres of biodegradable polymers. J Control Release 71:53–69
- Fonseca C, Simoes S, Gaspar R (2002). Paclitaxel-loaded PLGA nanoparticles: preparation, physicochemical characterization and in vitro anti-tumoral activity. J Control Release 83:273–86
- Hirai S, Yashiki H. (1981). Absorption of drugs from the nasal mucosa. Int J Pharm 7:317–21
- Huang C, Kimura R, Nassar RB, Hussain A. (1985). Mechanism of nasal absorption of drugs I: physicochemical parameters influencing the rate of in situ nasal absorption of drugs in rats. J Pharm Sci 74:608–12
- Illum L. (2002). Nasal drug delivery: new developments and strategies. Drug Discov Today 7:1184–9
- Illum L, Jorgensen H, Bisgaard H, et al. (1987). Bioadhesive microspheres as a potential nasal drug delivery system. Int J Pharm 39:189–99
- Jain SA, Chauk DS, Mahajan HS, et al. (2009). Formulation and evaluation of nasal mucoadhesive microspheres of Sumatriptan succinate. J Microencapsul 26:711–21
- Joshi SA, Chavhan SS, Sawant KK. (2010). Rivastigmine-loaded PLGA and PBCA nanoparticles: preparation, optimization, characterization, in vitro and pharmacodynamic studies. Eur J Pharm Biopharma 76:189–99
- Kulkarni RV, Wagh YJ, Setty CM, Sa B. (2011). Development and characterization of sodiumalginate-hydroxypropylmethylcellulosepolyester multilayered hydrogel membranes for drug delivery through skin. Polym-Plast Technol Eng 50:490–7
- Mahajan HS, Tatiya BV, Nerkar PP. (2012). Ondansetron loaded pectin based microspheres for nasal administration: in vitro and in vivo studies. Powder Technol 221:168–76
- Movva S, Kolachina V, Ranendra NS. (2008). Etoposide-loaded PLGA and PCL nanoparticles I: preparation and effect of formulation variables. Drug Deliv 15:267–75
- Musumeci T, Ventura CA, Giannone I, et al. (2006). PLA/PLGA nanoparticles for sustained release of docetaxel. Int J Pharm 325:172–9
- Panyam J, Labhasetwar V. (2003). Biodegradable nanoparticles for drug and gene delivery to cells and tissue. Adv Drug Delivery Rev 55:329–47
- Peppas NA. (1985). Analysis of Fickian and non-Fickian drug release from polymers. Pharm Acta Helv 60:110–1
- Pires A, Fortuna A, Alves G, Falcão A. (2009). Intranasal drug delivery: how, why and what for? J Pharm Pharm Sci 12:288–311
- Sakane T, Akizuki M, Yamashita S, et al. (1991a). The transport of a drug to the cerebrospinal fluid directly from the nasal cavity: the relation to the lipophilicity of the drug. Chem Pharm Bull 39:1458–2456
- Sakane T, Akizuki M, Yamashita S, et al. (1991b). Transport of cephalexin to the cerebrospinal fluid directly from the nasal cavity. J Pharm Pharmacol 43:449–51
- Seki T, Sato N, Hasegawa T, et al. (1994). Nasal absorption of zidovudine and its transport to cerebrospinal fluid in rats. Biol Pharm Bull 17:1135–7
- Shah N, Chaudhari K, Dantuluri P, et al. (2009). Paclitaxel-loaded PLGA nanoparticles surface modified with transferrin and Pluronic-P85, an in vitro cell line and in vivo biodistribution studies on rat model. J Drug Targeting 17:533–42
- Tas C, Ozkan CK, Savaser A, et al. (2006). Nasal absorption of metoclopramide from different Carbopol 981 based formulations: in vitro, ex vivo and in vivo evaluation. Eur J Pharm Biopharm 64:246–54