Abstract
Abstract: The temperature effects on enzyme activity at the conditions with and without inhibition, operation, and storage were investigated for free esterase and immobilized chicken liver esterase with three methods (adsorption, adsorption-microcapsule, and adsorption-crosslinking) used in a calorimetric biosensor. The results indicated that the temperature had significant effects on the enzyme activity by means of catalytic reaction, irreversible denaturation, mass transfer, and structure deformation of resin. Among all the esterase, the adsorption-crosslinking immobilized esterase had the best performances of enzyme activity retention ratio (EARR), above 96% in operation and 90% in storage on condition of maintaining its response to dichlorvos.
INTRODUCTION
Due to its universality and insensibility to the optical, electrochemical, and other inherent interferential factors of crude samples, an enzyme-based calorimetric biosensor has been widely used in many fields such as enzyme activity measurements, multianalyte determination, and environmental monitoring [Citation1–4]. In the previous research [Citation5–6], a flow injection calorimetric biosensor has been developed for the rapid detection of organophosphorus pesticides. For low cost, chicken liver esterase is used as an analytical reagent instead of acetyl cholinesterase (AChE) in the calorimetric biosensor and its inhibition degree of enzyme activity can represent the content of pesticides in samples. As a working parameter and output signal of the calorimetric biosensor, temperature plays an important role in detection. To achieve high detection accuracy and reproducibility, the impacts of temperature on enzyme activity should be kept as small as possible for the calorimetric biosensor. However, enzyme activity of the esterase with or without inhibition inevitably changes with the temperature fluctuation. Furthermore, the esterase can be easily inactivated during the operation and storage in which temperature has fatal effects on enzyme activity.
Enzymes can be more stabilized by immobilization using a variety of chemical and physical techniques, such as adsorption, crosslinking, entrapment, and so on [Citation7–10]. Among these methods, adsorption of enzymes to the ion exchange matrix is less costly and convenient in preparation. Its application has been illustrated by chicken liver esterase immobilized on D113 resin used in the calorimetric biosensor [Citation6,Citation11]. However, the inherent infirmness of adsorption results in irregular loss of enzyme activity, which affects the detection accuracy. This problem can be solved through the further process of the microcapsule with calcium alginate or crosslinking with glutaraldehyde after ion exchange adsorption immobilization without consuming much money and time.
The main objectives of this research were to investigate the effects of temperature on enzyme activity of chicken liver esterase at various conditions of the calorimetric biosensor. Experiments with different parameters of relative enzyme activity, inhibition ratio of enzyme activity, and enzyme activity retention ratio (EARR) were carried out. Performances of free esterase and immobilized esterase with three methods (adsorption, adsorption-microcapsule, and adsorption-crosslinking) were contrasted. The ultimate aim of this research was to improve stability of immobilized esterase and select detection temperature for the calorimetric biosensor.
MATERIALS AND METHODs
Materials
Fresh chicken liver was supplied by Shanghai Dajiang Food Company. D113 ion exchange resin was purchased from Tianjin Nankai Chemical Plant. Glutaraldehyde, a-naphthyl acetate, and fast blue B salt were purchased from Sigma Company; other reagents were of analytical grade.
Extraction of Chicken Liver Esterase
The chicken liver was mixed with citric acid buffer (0.025mol/L, pH 6.4) in the ratio of 1g/ml. The mixture was homogenized by a tissue triturator (Model DS-1, Shanghai Sampling Model Co.) and then separated for 10 minutes at 5000r/min with a centrifuge (Model 90-1, Beijing General Centrifuge Works). The crude chicken liver esterase was obtained from the final supernatant and stored at −18°C before use.
The crude chicken liver esterase was diluted to 6 ± 2U/ml of enzyme activity with citric acid buffer (0.025mol/L, pH 6.4). The dilution solution was obtained as free esterase.
Immobilization of Chicken Liver Esterase
Wet D113 resin between 35 and 40 meshes was dehydrated by centrifugation and then stirred with 0.5M NaOH/HCl solution in sequence of NaOH-HCl-NaOH for 12 hours, respectively. De-ion water washing should be performed between the reagents. The prepared resin was stored in deionized water at 4°C before use.
The adsorption immobilized esterase was obtained by mixing free esterase and pretreated D113 resin in the ratio of 3ml/g after being stirred for 75 minutes.
The adsorption-microcapsule immobilized esterase was prepared as follows: the adsorption immobilized esterase was slowly and evenly added into 0.2% SA (Sodium Alginate) solution, and separated from SA solution for 1 minute at 5000r/min, then transferred into 0.1mol/L CaCl2 (Calcium Chloride) solution and rested for 30 minutes.
The adsorption-crosslinking immobilized esterase was obtained by adding the adsorption immobilized esterase into 0.04% glutaraldehyde solution, then the mixture was stirred for 60 minutes at 10°C.
After immobilization, immobilized esterase produced by three methods were separated and washed repeatedly by deionized water until no enzyme was left in the water. The immobilized esterase were stored in deionized water at 4°C before use.
General Esterase (GE) Activity Assay
The analytical procedure of free esterase was as follows. First, 3ml esterase solution properly diluted by citric acid buffer (0.025mol/L, pH 6.4) was mixed with 50μl a-naphthyl acetate of 80mmol/L at a certain reaction temperature for 5 minutes in a test tube. Second, 0.5ml of stop reagent (0.4% fast blue B in 3.6% SDS) was added at 30°C for 5 minutes. Third, absorbance of the reacted solution was read on a spectrophotometer (Model 722S, Shanghai Analytical Instrument Company) at 595 nm with buffer as reference. Last, the GE activity was calculated by the following equation:
where EL is the GE activity of chicken liver esterase solution (U/ml); D dilution times of crude chicken liver esterase; K the slope of the standard curve of a-naphthol solution (μM/OD); A the optical density (OD) of the reacted solution at 595nm; and U the enzyme activity unit (μmol/min).
The analytical procedure of immobilized esterase was as follows. First, 0.25g immobilized enzyme and 50 μl a-naphthy acetate of 80mmol/L were added into 20ml citric acid buffer (0.025mol/L, pH 6.4) in sequence at a certain reaction temperature for 5 minutes. Second, the 3ml reacted solution was mixed with 0.5 ml stop reagent in a test tube for another 5 minutes at 30°C. Third, the absorbance of final solution was measured at 595 nm using buffer as reference solution. Last, the GE activity of immobilized esterase was calculated by the following equation:
where Es is GE activity of immobilized enzyme (U/g); W the weight of immobilized enzyme (g); and other symbols have the same meanings as in Eq. (1). The factor 20 indicates the volume of the reacted solution (ml).
Definition of Parameters
To compare the enzyme activity of free and immobilized esterase at different temperature, the relative enzyme activity was defined as follows:
The inhibition ratio reflecting the degree of inhibition was calculated from the enzyme activity with and without inhibition by the following equation:
In order to evaluate the ability to maintain enzyme activity in operation and storage, the enzyme activity retention ratio (EARR) was defined as follows:
Statistics Analysis
Experimental results are the mean ± SD for three replicates. The means comparison of EARR at different operational temperature is calculated by one-way ANOVA statistical test at the significance level of 0.05 using the software of Origin 8.1 (OriginLab Corporation).
RESULTS AND DISCUSSION
Temperature Effects on Enzyme Activity
Temperature is an important parameter for enzyme-catalyzed reaction. The enzyme activity of chicken liver esterase with free and immobilized forms was measured by GE activity assay in the reaction temperature range from 25°C to 70°C at the interval of 5°C.
shows the temperature effects on the relative enzyme activity of the chicken liver esterase. The result was consistent with the general law of enzymes changing with temperature. According to the Arrhenius Law, the rise of temperature resulted in the increase of enzyme activity by enhancing the molecules kinetic energy and reducing the activation energy of the catalytic reaction. In addition, when substrate and esterase were mixed well, temperature had more impact on the enzyme activity of immobilized esterase than that of free esterase by mass transfer diffusing at the micro inner channel and boundary layer of D113 resin. So, the changing rate of enzyme activity with temperature for free esterase was smaller than that of immobilized esterase. The curves were almost linear with temperature just beyond a certain point defined as optimum temperature, as shown in . After that point, the enzyme as protein was gradually denatured with temperature increasing and enzyme activity started to decrease. The free and immobilized esterase had different optimum temperature, which was around 50°C for adsorption and adsorption-crosslinking immobilized esterase, and 55°C for free esterase and adsorption-microcapsule immobilized esterase. The result was totally different from traditional conclusion that immobilized esterase has higher optimum temperature than free esterase. The reason might be the structure deformation of D113 resin, which further weakened mass transfer of immobilized esterase using D113 resin as carrier. This assumption was confirmed by the DSC (Differential Scanning Calorimeter, Diamond DSC, PerkinElmer Inc.) scan curve in , and the heat flow reached its minimal value and the trend changed at 50°C, which reflected thermal structure deformation. When temperature increased to 50°C, the micro inner channel of D113 resin may disappear or narrow, which weakens mass transfer between substrate and esterase and leads to lower enzyme activity. For adsorption-microcapsule immobilized esterase, protection of gel mesh resulted in higher optimum temperature.
Figure 1. Temperature effects on relative enzyme activity of free and immobilized chicken liver esterase.
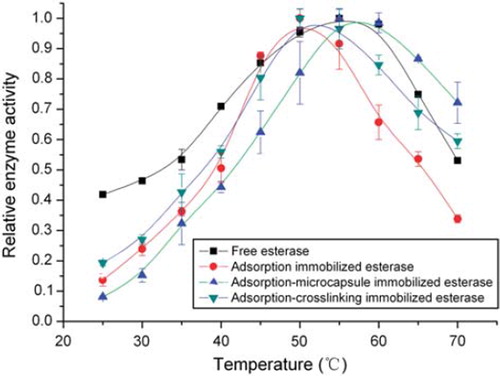
The above results indicated that the effects of temperature on enzyme activity could be described by the following factors: catalytic reaction, irreversible denaturation, mass transfer, and structure deformation of resin. All of them affected the enzyme activity from experiment and functioned differently under different given situations.
Temperature Effects on Enzyme Activity with Inhibition
As is well known, inhibitor and temperature have significant effects on inhibition degree and ability of enzyme, which reflects sensitivity and resolution of detection. In this research, dichlorvos was diluted with a citric acid buffer to different concentration from 0.1 mg/L to 1 mg/L. Substituting dichlorvos solution for citric acid buffer, the enzyme activity of inhibited chicken liver esterase was separately measured at reaction temperatures of 30°C, 40°C, 50°C, 60°C, and 70°C using the GE activity assay method.
showed the temperature effects on the inhibition ratio of free and immobilized chicken liver esterase by dichlorvos with different concentrations. For all of esterase, the changes of inhibition ratio with temperature were small and nearly linear under 50°C of optimum temperature. The linear relation could be used for temperature correction in rapid detection under fluctuant temperature environment.
Figure 3. Temperature effects on inhibition ratio of free and immobilized chicken liver esterase: (a) free esterase; (b) adsorption immobilized esterase; (c) adsorption-microcapsule immobilized esterase; (d) adsorption-crosslinking immobilized esterase.
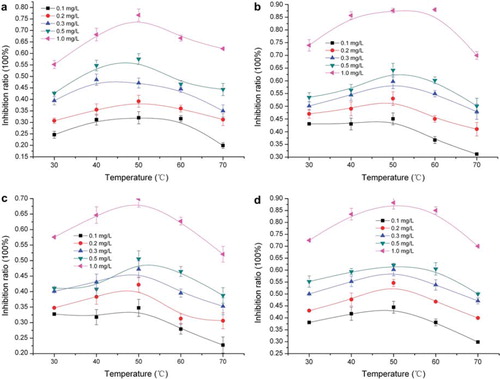
From the inhibition curves, the inhibition ratio of adsorption immobilized esterase was found obviously higher than that of free esterase as a result of the purification effect in the process of immobilization, namely the response of adsorption immobilized esterase to dichlorvos was higher than that of free esterase. First, free esterase and immobilized esterase were in the different distribution status in the inhibition reaction system. Compared with impurity contained by the free esterase, for immobilized esterase enzyme protein was bonded on the surface and the micro inner channel of D113 ion exchange resin, and there was a purification effect in this process of immobilization. On the other hand, the contact area per unit volume of immobilized esterase was far bigger than that of free esterase, which explains why immobilized esterase possessed a higher inhibition ratio. However, the inhibition ratio of adsorption-microcapsule immobilized esterase was similar to that of free esterase. It resulted from the fact that gel mesh formed by the microcapsule process affected the mass transfer of enzyme reaction, and the protection of the gel mesh made the enzyme more difficult to contact substrate. The inhibition ratio of adsorption-crosslinking immobilized esterase was close to that of adsorption immobilized esterase. Therefore the response of immobilized esterase to dichlorvos was still sensitive after the crosslinking process based on adsorption, which had an advantage for the detection of dichlorvos.
Temperature Effects on Enzyme Activity in Operation
The persistence of enzyme activity in operation is indispensable to the detection of reproducibility. However, the calorimetric biosensor was based on flow injection analysis, and the reagent and buffer washed off the immobilized esterase at a certain temperature and flux, which resulted in the loss of enzyme activity. To investigate the operational stability, the immobilized chicken liver esterase produced by three methods were naturally packed into reaction columns and the calorimetric biosensor run with buffer flux of 1ml/min at the temperatures of 30°C, 40°C, 50°C, and 60°C. After 30 minutes, the enzyme activity of immobilized esterase was immediately measured by the GE activity assay method at a reaction temperature of 30°C.
shows the change of the EARR with temperature under the above condition, which indicated that the temperature had little effect on the EARR of immobilized chicken liver esterase in operation. Furthermore, the means comparison of the EARR at different temperature showed that there was no significant difference for all of the immobilized esterase. This demonstrated that the loss of enzyme activity in the operational process was mainly because of mechanical wash and erosion, and the effect of temperature could be neglected as a whole.
Table 1. Temperature effects on enzyme activity retention ratio (EARR) for immobilized esterase in operation.
Due to weak intermolecules’ force for adsorption, the EARR of adsorption immobilized esterase was the lowest and close to 70%, while the EARR of both adsorption-crosslinking and adsorption-microcapsule immobilized esterase had the same magnitude of 96% after the operational process. The better operational stability benefited from the extra-strong cross-linked force and protection of gel mesh.
Temperature Effects on Enzyme Activity in Storage
Normally, it takes a long storage period for esterase from manufacture to use. During this storage, protein denaturation causes the steady drop of enzyme activity. To preserve esterase, low temperature environment is a usual way to maintain its enzyme activity [Citation12]. During the experiment, the chicken liver esterase was subpacked into small samples and stored at four different temperatures. Under non-frozen states, the samples were stored in a thermostatic water bath at 30°C and a cooling chamber at 4°C separately. While under the frozen states, the samples were plunged into liquid N2 directly, then stored at −18°C of frozen chamber and −178°C of liquid N2 after being entirely frozen. At each end of intervals, the samples were taken out for immediate measurement by the GE activity assay method. During this process, the frozen esterase was thawed in a thermostatic water bath at 30°C first, and the non-frozen esterase did not need this step.
It can be seen from that the EARR of all esterase at 30°C declined deeply within the initial several hours and deceased slowly during the later period. By contrast, the EARR of free and absorption immobilized esterase decreased rather sharply. At the end of 24 hours, the value of EARR reached 20% for free and adsorption immobilized esterase and 50% for adsorption-microcapsule and absorption-crosslinking immobilized esterase. However, the value of 50% was still insufficient to meet the accuracy and reproducibility for detection. The above results indicated that the esterase should not be exposed at an environment of 30°C or higher temperature, even for a few hours.
Figure 4. The enzyme activity retention ratio (EARR) of free and immobilized chicken liver esterase at different temperature in storage: (a) 30°C; (b) 4°C; (c) −18°C; (d) −178°C.
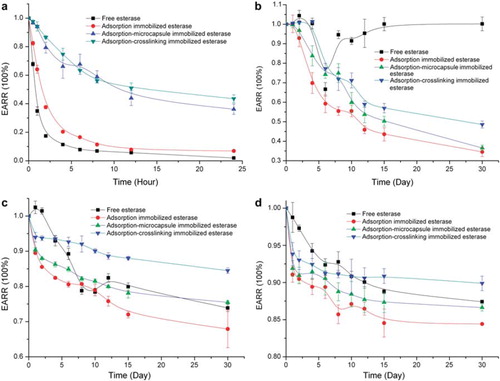
At 4°C of the non-frozen state, as shown in (b), the change of EARR for free esterase decreased until 6 days and increased after 6 days, while the immobilized esterase didn’t show any similar result. Free esterase extracted from chicken liver was crude and impure. It contained several kinds of esterase and impurity and was easily contaminated by a microorganism compared with immobilized esterase. The microorganism might produce enzyme and other substances which showed false general esterase activity. In fact, the free esterase became mildewed and smelled because of microbial contamination after 6 days, whereas the immobilized one kept its normal form and smell. From , it also can be seen that the EARR of three immobilized esterase declined slowly and reached the scope of 30% to 50% after 30 days.
At −18°C and −178°C of the frozen state, as shown in , the EARR of free esterase declined gradually, but the EARR of three immobilized esterase declined by a small margin during the 30 days. In addition, the EARR of three immobilized esterase had a sharp decline without exception every day. The reason was that great mechanical and thermal stress detached the enzyme from the D113 resin in the process of freezing and thawing, which further resulted in the decay of enzyme activity of immobilized esterase. The EARR for all the esterase at −178°C was around 10% higher than that of −18°C. Furthermore, the constant EARR for adsorption-crosslinking immobilized esterase at −178°C indicated that the catalytic ability of esterase could be maintained at a stable level until use.
In summary, all the esterase must be kept under the frozen state in order to prevent the rapid decline of enzyme activity. The stability of immobilized esterase was better than that of free esterase, and became better and better in the following sequence: adsorption, adsorption-microcapsule, and adsorption-crosslinking immobilized esterase.
Selection of Detection Temperature
To ensure sensitivity and reproducibility, detection temperature must be optimized for the calorimetric biosensor. In general, the detection temperature should be set a point at which enzyme activity of esterase is high and stable with temperature fluctuation. Furthermore, great departures of detection temperature from the environment require a higher control precision for thermostat. The enzyme activity and its inhibition degree in responding to dichlorvos reached the maximum at 50°C from and . The reference temperature for enzyme catalytic reaction is modified from 30°C to 37°C recommended by IFCC (International Federation of Clinical Chemistry) [Citation13]. Based on the above information, the detection temperature of 37°C was the optimum for the calorimetric biosensor.
CONCLUSIONS
Temperature was an important factor in enzyme activity of chicken liver esterase in terms of catalytic reaction, irreversible denaturation, mass transfer, and structure deformation of resin. The enzyme activity with and without inhibition by dichlorvos reached the maximum at optimum temperature. The change of inhibition degree with temperature from 30°C to 70°C was within 10%, and had a linear relation before optimum temperature. Compared with temperature, the mechanical damage was the main reason for operational loss of the esterase. To maintain higher catalytic ability in storage, frozen preservation for the esterase was essential, and temperature should be kept as low as possible if not taking cost into consideration.
The performance of immobilized esterase produced by three methods varied dramatically. Among them, the adsorption-crosslinking immobilized esterase had the best stability with enzyme activity retention ratio (EARR) above 96% in the operation and above 90% in the storage without affecting the response to dichlorvos.
The calorimetric biosensor should be operated at an optimum temperature of 37°C according to the results.
Declaration of interest: The authors report no conflicts of interest. The authors alone are responsible for the content and writing of the paper.
REFERENCES
- Danielsson, B., Hedberg, U. and Rankand, B. (1992). Recent investigations on calorimetric biosensors. Sensors and Actuators B: Chemical, 6: 138–142.
- Ramanathan, K. and Danielsson, B. (2001). Principles and applications of thermal biosensors. Biosensors and Bioelectronics, 16: 417–423.
- Zhang, Y. Y. and Tadigadapa, S. (2004). Calorimetric biosensors with integrated microfluidic channels. Biosensors and Bioelectronics, 19: 1733–1743.
- Wang, L., Sipe, D. M., Xu, Y. and Lin, Q. (2008). A MEMS thermal biosensor for metabolic monitoring applications. Journal of Microelectromechanical Systems, 17: 318–328.
- Zhang, H. J., Xu, X. Q., Xu, F. and Hua, T. C. (2004). The comparison of two kinds of enzyme in rapid detection of pesticide residue. Chinese Journal of Analytical Chemistry, 32: 1517–1520.
- Zheng, Y. H., Hua, T. C., Sun, D. W., Xiao, J. J., Xu, F. and Wang, F. F. (2006). Detection of dichlorvos residue by flow injection calorimetric biosensor based on immobilized chicken liver esterase. Journal of Food Engineering, 74: 24–29.
- Guisan, J. (2006). Immobilization of Enzymes and Cells, Humana Pr Inc.
- Gupta, R. and Chaudhury, N. (2007). Entrapment of biomolecules in sol-gel matrix for applications in biosensors: Problems and future prospects. Biosensors and Bioelectronics, 22: 2387–2399.
- Marquez, L., Cabral, B., Freitas, F., Cardoso, V. and Ribeiro, E. (2008). Optimization of invertase immobilization by adsorption in ionic exchange resin for sucrose hydrolysis. Journal of Molecular Catalysis B: Enzymatic, 51: 86–92.
- Alonso, N., Lopez-Gallego, F., Betancor, L., Hidalgo, A., Mateo, C., Guisan, J. and Fernandez-Lafuente, R. (2005). Immobilization and stabilization of glutaryl acylase on aminated sepabeads supports by the glutaraldehyde crosslinking method. Journal of Molecular Catalysis B: Enzymatic, 35: 57–61.
- Xu, F., Xu, X. Q., Zhang, H. J., Jiang, M. and Hua, T. C. (2007). Experimental study on utilization of chicken liver esterase in determination of organophosphate and carbamate pesticides. Chinese Journal of Food Science, 28: 237–241.
- Xu, F., Hua, T. C., Sun, D. W., Xiao, J. J. and Zhao, X. L. (2005). Enzyme activity of wheat esterase as affected by various cryopreservation conditions. Journal of Food Engineering, 69: 17–22.
- Panteghini, M. and Forest, J. (2005). Standardization in laboratory medicine: New challenges. Clinica Chimica Acta, 355: 1–12.