Abstract
Abstract: Retention time, which is analogous to transit time, is an index for bacterial stability in the intestine. Its consideration is of particular importance to optimize the delivery of probiotic bacteria in order to improve treatment efficacy. This study aims to investigate the effect of retention time on Lactobacilli and Bifidobacteria stability using an established in vitro human colon model. Three retention times were used: 72, 96, and 144 h. The effect of retention time on cell viability of different bacterial populations was analyzed with bacterial plate counts and PCR. The proportions of intestinal Bifidobacteria, Lactobacilli, Enterococci, Staphylococci and Clostridia populations, analyzed by plate counts, were found to be the same as that in human colonic microbiota. Retention time in the human colon affected the stability of Lactobacilli and Bifidobacteria communities, with maximum stability observed at 144 h. Therefore, retention time is an important parameter that influences bacterial stability in the colonic microbiota. Future clinical studies on probiotic bacteria formulations should take into consideration gastrointestinal transit parameters to improve treatment efficacy.
INTRODUCTION
The human gut microbiota is a tremendous microbial ecosystem whose homeostasis is vital to the maintenance of human health. Intestinal bacterial communities carry structural, protective, and metabolic functions including intestinal barrier fortification, activation, and development of the immune system and fermentation of non-digestible dietary residues [Citation1,Citation2]. However, an alteration in the composition of the gut microbiota, a state called dysbiosis, is linked with the development of a plethora of human diseases such as inflammatory bowel diseases [Citation3,Citation4], the irritable bowel syndrome [Citation5,Citation6] and colorectal cancer [Citation7,Citation8].
The intimate link between the gut microbiota and the overall health status of its host explain the growing interest for probiotic bacteria, essentially Lactobacillus sp. and Bifidobacterium sp., which aim to modulate the gut microbiota in favor of health-promoting bacterial populations [Citation9,Citation10]. However, the dynamics over time of bacterial communities, either autochthonous (resident bacteria) or allochthonous (bacteria in transit), in human intestinal compartments is poorly understood.
Effect of retention time (Rt), which is analogous to transit time, is one of the major parameters that can be of interest to understand the variations in the composition of the gut bacterial ecosystem. Earlier studies have been done to evaluate its effect on human bacterial communities [Citation11,Citation12]. However, Rt effect studies specific to bacteria species such as Lactobacilli and Bifidobacteria are yet to be investigated, which is the major goal of this study.
Since the in vivo study of the colonic flora presents considerable methodological issues, in vitro models can be of immense importance [Citation11–14]. Batch culture fermentor models of the human gut microbiota offer a remarkable opportunity to perform ecological studies [Citation13–15]. They provide reproducible results and allow for long-term mechanistic studies with controlled parameters.
In this study, the effect of Rt on the quantitative stability of Lactobacilli and Bifidobacteria populations was investigated in a human colonic in vitro model. For this, three fermentations were carried out in a well-established gastrointestinal model under controlled clinical colonic conditions. Lactobacilli and Bifidobacteria community stability were assessed over 35 days with bacterial plate counts and semi-quantitative bacterial DNA analysis using PCR.
MATERIALS AND METHODS
In Vitro Colon Model
A semi-continuous colon model fermentation system () was set up to represent the human colonic microbiota based on our well-established computer-controlled human dynamic gastrointestinal model [Citation16,Citation17]. The colon model temperature was kept constant at 37°C by the flow of hot water in the double jacketed vessel. A microbial solution was continuously stirred at 150 rpm. pH was computer-controlled at 6.4 using 0.5 M NaOH and 0.2 M HCl solutions. The anaerobic atmosphere condition was maintained by flushing nitrogen gas for 20 min every day. The nutritive solution consisted of a carbohydrate-based solution. The formulation was: 1 g/L arabinogalactan, 4 g/L mucin, 1 g/L xylan, 1 g/L pepton, 3 g/L yeast extract, 3 g/L starch (Sigma-Aldrich), 2 g/L pectin, 0.4 g/L glucose (Acros Organics), and 0.5 g/L cystein (Fisher Scientific-Bioreagents) diluted into sterile water and adjusted to pH 6.4 [Citation13].
Figure 1. Schematic representation of the semi-continuous colon model fermentation system derived from the computer-controlled human dynamic gastrointestinal tract [Citation16]. The fermentor is continuously magnetically stirred. Temperature of the culture system is kept constant at 37 °C by the flow of hot water in the double-jacketed vessel. pH is computer controlled at 6.4. Portholes allow for the addition of food solution and the removal of the culture solution. Anaerobic atmosphere is maintained by the flush of nitrogen.
![Figure 1. Schematic representation of the semi-continuous colon model fermentation system derived from the computer-controlled human dynamic gastrointestinal tract [Citation16]. The fermentor is continuously magnetically stirred. Temperature of the culture system is kept constant at 37 °C by the flow of hot water in the double-jacketed vessel. pH is computer controlled at 6.4. Portholes allow for the addition of food solution and the removal of the culture solution. Anaerobic atmosphere is maintained by the flush of nitrogen.](/cms/asset/980da095-3f0e-44a4-ba90-638051b36277/ianb19_a_622280_f0001_b.jpg)
Fermentation Procedure in the Colon Model
Fecal inoculum was collected from a healthy adult volunteer (age 22) with no antibiotic use within the last 6 months. It was freshly diluted to 15% in sterilized physiological solution (8.5 g/L NaCl). 400 mL of the diluted inoculum was directly transferred to the fermentor culture system. Nutritive solution was added to reach a final working volume of 600 mL.
Three fermentations were carried out using different Rt: 72, 96, and 144 h. Rt, which represents the time allowed for a substance to remain in the system, was set up in order to simulate the human gut microbial populations residency in the colon [Citation18]. It was calculated as the reciprocal of dilution rate ().
Table 1. Retention time during fermentation 1, 2, and 3 as determined by operating parameters.
A start-up period of 12 days allowed the bacterial communities from the fecal inoculum to grow and stabilize in order to be representative of the gut microbiota [Citation14,Citation19].
Bacterial Population Analysis
Plate counts
Isolation media were used to determine bacterial cell viability, calculated as the colony-forming unit (CFU)/mL. Bifidobacteria and Lactobacilli were quantified, respectively, on raffinose bifidobacterium powder and LAMVAB [Citation20,Citation21]. Total aerobic and anaerobic bacteria were enumerated using brain heart infusion agar (Becton Dickinson). Enterococci were counted on Enterococcus agar (Becton Dickinson). Staphylococci and Clostridia were quantified on mannitol salt agar (Sigma-Aldrich) and tryptose sulphite cycloserin agar (Remel). Inoculums were extracted from each fermentation system and freshly serially diluted in physiological solution (8.5 g/L NaCl). Plates were immediately streaked in triplicates with 0.1 mL of three different suitable dilutions. Anaerobic incubation was performed in anaerobic jars, with anaerobe atmosphere generating bags (Oxoid), for 48 h at 37 °C. The cell count of total bacteria was obtained by adding cell counts of total aerobes and total anaerobes bacteria.
PCR analysis
PCR analysis was performed to evaluate any changes in the composition of Lactobacilli and Bifodobacteria. Inoculums were removed from each fermentation system and DNA extraction was immediately performed using the QIAamp DNA stool mini kit (Qiagen) according to the manufacturer's instructions. Total and species-specific bacterial DNA was amplified using specific primers [Citation22–24] () with optimized PCR conditions. Taq DNA polymerase (Invitrogen) was used for the polymerase reaction.
Table 2. PCR primers used to analyze Bifidobacteria, Lactobacilli, and total bacteria.
RESULTS
This study was performed to investigate the effect of Rt on the community stability of Lactobacilli and Bifidobacteria. Three series of fermentations were carried out using different Rt (72, 96, and 144 h) in a human colonic in vitro model under controlled conditions. Lactobacilli and Bifidobacteria quantitative stability was assessed during 35 days with bacterial plate count and PCR techniques.
In order to ensure that the bacterial ecosystem of the colon model mimicked the human gut flora, the mean concentration of total bacteria, Enterococci, Staphylococci, Bifidobacteria, Lactobacilli, and Clostridia was compared to available data from the literature on the human colonic microbiota () [Citation9]. Mean log viable counts of all intestinal bacterial groups corresponded well with clinical data, although the bacterial populations were somewhat lower. Nevertheless, this is in accordance with previous results [Citation14]. In particular, during fermentation 1 (Rt: 72 h), 2 (Rt: 96 h) and 3 (Rt: 144 h) Bifidobacteria cell counts were respectively 6.24 ± 0.64, 6.01 ± 0.84, and 6.92 ± 0.65 log CFU/mL while Lactobacilli cells counts reached respectively 4.03 ± 0.26, 4.23 ± 0.26, and 3.61 ± 0.3165 log CFU/mL. Thus, the bacterial ecosystem within the fermentor culture system mimicked the human colonic gut flora.
Table 3. Comparison of the cell viability of Lactobacilli and Bifidobacteria in the presence of other intestinal bacterial groups during fermentation 1 (Rt = 72 h), 2 (Rt = 96 h), and 3 (Rt = 144 h) to available human colon data. Mean log viable count and standard deviation were calculated from plate counts between day 0 and day 35. Clinical data are presented as the range of the average composition of the adult ecosystem [Citation9]
To evaluate the effect of Rt on the quantitative stability of Lactobacillus sp. and Bifidobacterium sp., the percentage bacterial cell variability, computed as the variation coefficient of triplicate plate counts between day 0 and day 5, 10, 15, 25 and 35, was calculated (). Fermentation 3 (Rt: 144 h) had the smallest percentage bacterial cell variability of Lactobacilli and Bifidobacteria on day 5, 10, 15, 25, and 35, as compared to fermentation 1 (Rt: 72 h) and 2 (Rt: 96 h). Indeed, the percentage variability of Bifidobacteria during fermentation 3 was only 12 % (day 5), 32 % (day 10), 47 % (day 15), 88 % (day 25), and 28 % (day 35), while it was bigger during fermentation 1 and 2. The same findings were observed for the percentage bacterial cell variability of Lactobacilli during fermentation 3, except on day 10, when it reached 1 % during fermentation 2.
Figure 2. Percentage bacterial cell variability, computed as the variation coefficient calculated from plate counts, of Lactobacilli (A) and Bifidobacteria (B) during fermentation 1 (Rt : 72 h), 2 (Rt : 96 h), and 3 (Rt : 144 h) between day 0 and day 5, 10, 15, 25, and 35.
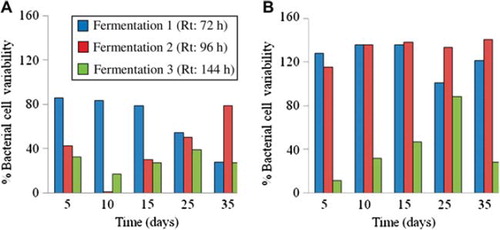
In order to reconfirm the data obtained using bacterial cell counts, PCR was used as a molecular technique to monitor change in the composition of Lactobacilli and Bifodobacteria at the DNA level (). Band intensities of the PCR products were normalized by that of total bacteria. Band intensities remained significantly comparable during fermentation 3 and averaged 1.21 ± 0.03 arbitrary units for Bifidobacterium sp. () and 1.24 ± 0.02 arbitrary units for Lactobacillus sp. (). On the contrary, band intensities of Lactobacilli and Bifodobacteria were significantly different during fermentation 1 (72 h) and 2 (Rt: 96 h).
Figure 3. PCR analysis of the inoculum samples per group analyzed (Bifidobacteria, Lactobacilli and total bacteria) and fermentation 1 (Rt: 72 h), 2 (Rt: 96 h), and 3 (Rt: 144 h) on days 0, 15, and 35 (A). Abbreviations: L - Ladder; NTC - Non template control. The graphs (B: Bifidobacteria and C: Lactobacilli) are the semi-quantitative representation of the band intensities PCR products as analyzed by Image J software. The band intensities of the PCR products were normalized by that of total bacteria. The band intensities of Fermentation 1 on day 0 have been taken as 1, and the other band intensity values were represented relative to that. Data represent the means ± SD (n = 3).

DISCUSSION
The importance of a healthy gut microbiota extends to a number of extra-intestinal processes and organ systems [Citation1,Citation25,Citation26]. Focus has been particularly directed towards Lactobacilli and Bifidobacteria. Both are autochthonous members of the human gut microbiota and positively associated with health-promoting effects [Citation27,Citation28]. To maintain and/or favor human health, oral ingestion of probiotic supplements of Lactobacillus and Bifidobacterium have been successively used [Citation10,Citation29–31]. However, the dynamics of Lactobacillus sp. and Bifidobacterium sp. in human intestinal compartments is poorly understood. In particular, there is little data on the effect of Rt, analogous of transit time, on Lactobacilli and Bifidobacteria. Clinical studies have demonstrated that total transit time varies from hours to days in healthy individuals [Citation18]. Most of the variability tends to be associated with the colon [Citation18].
In this study, Rt values (72, 96, and 144h) were selected to simulate the human colonic bacterial transit in the human colon. Both culture-dependent and molecular techniques demonstrated that stability of Lactobacilli and Bifidobacteria populations in the in vitro semi-continuous colon model fermentation system was affected by Rt. Rt operated during fermentation 3 - 144 h - was optimal to maintain quantitatively stable populations of Lactobacilli and Bifidobacteria during a period of 35 days. However, there was surprisingly no trend observed between Rt and stability of Lactobacilli and Bifidobacteria populations. Although it is an approximation, this model allows the experimentation of a complex culture of the gut microbiota under well-standardized conditions. It can easily be operated and demonstrates considerable Lactobacilli and Bifidobacteria population stability with a Rt of 144h. As a consequence, this model offers a reproducible method to appropriately determine the effect of probiotic bacteria on the intestinal ecosystem.
Despite the increased research interest in the development of probiotic formulations, detailed mechanisms of action are still lacking. The effect of ingested Lactobacillus and Bidifobacterium sp. on the gut microbiota ecosystem is poorly understood. In particular, the effect of probiotic bacterial dose frequency and timing on an intestinal ecosystem remains unknown. This study demonstrates that the stability of resident Lactobacilli and Bifidobacteria populations is affected by Rt in an in vitro model of human colonic microbiotia. Therefore, resident Lactobacilli and Bifidobacteria populations might be influenced by colonic transit time.
Extrapolation from those findings suggests that the potential effects of ingested Lactobacilli and Bifidobacteria probiotic bacteria to interact and colonize the gastrointestinal tract might be influenced by colonic transit. Consequently, dosing frequency and timing might be determined to increase ingested probiotic activity within the intestines for an enhanced effect on human health.
CONCLUSION
There has been an increasing interest in probiotic bacteria to modulate the gut microbiota ecosystem in favor of health-promoting bacterial populations. Probiotics such as Lactobacilli and Bifidobacteria are autochnonous inhabitants of the human gastrointestinal tract. This study is the first to demonstrate in an in-vitro model of human colonic microbiotia that the stability of autochthonous Lactobacilli and Bifidobacteria is affected by colonic retention time, which is analogous to transit time. As a consequence, transit time is an important parameter to take into account to deliver probiotic bacterial formulations. To reach maximal treatment efficacy, the dosage of Lactobacilli and Bifidobacteria probiotic bacteria formulations should take into consideration food frequency and gastrointestinal transit parameters. Future clinical studies should aim at defining the effect of dosing frequency and timing on the efficacy of probiotic bacterial formulations.
Declaration of interest: The author reports no conflicts of interest in this work. The authors alone are responsible for the content and writing of the paper.
REFERENCES
- Round, J.L., Mazmanian, S.K. (2009). The gut microbiota shapes intestinal immune responses during health and disease. Nat Rev Immunol 9(5):313–23.
- Salzman, N.H., Underwood, M.A., Bevins, C.L. (2007). Paneth cells, defensins, and the commensal microbiota: a hypothesis on intimate interplay at the intestinal mucosa. Semin Immunol 19(2):70–83.
- Macfarlane, S., Steed, H., Macfarlane, G.T. (2009). Intestinal bacteria and inflammatory bowel disease. Crit Rev Clin Lab Sci 46(1):25–54.
- Peterson, D.A., Frank, D.N., Pace, N.R., Gordon, J.I. (2008). Metagenomic approaches for defining the pathogenesis of inflammatory bowel diseases. Cell Host Microbe 3(6):417–27.
- Si, J.M., Yu, Y.C., Fan, Y.J., Chen, S.J. (2004). Intestinal microecology and quality of life in irritable bowel syndrome patients. World J Gastroenterol 10(12):1802–5.
- Kerckhoffs, A.P., Samsom, M., van der Rest, M.E., De, V.J., Knol, J., Ben-Amor, K., Akkermans, L.M. (2009). Lower bifidobacteria counts in both duodenal mucosa-associated and fecal microbiota in irritable bowel syndrome patients. World J Gastroenterol 15(23):2887–92.
- Moore, W.E., Moore, L.H. (1995). Intestinal floras of populations that have a high risk of colon cancer. Appl Environ Microbiol 61(9):3202–7.
- Scanlan, P.D., Shanahan, F., Clune, Y., Collins, J.K., O’Sullivan, G.C., O’Riordan, M., Holmes, E., Wang, Y., Marchesi, J.R. (2008). Culture-independent analysis of the gut microbiota in colorectal cancer and polyposis. Environ Microbiol 10(3):789–98.
- Orrhage, K., Nord, C.E. (2000). Bifidobacteria and lactobacilli in human health. Drugs Exp Clin Res 26(3):95–111.
- Gareau, M.G., Sherman, P.M., Walker, W.A. (2010). Probiotics and the gut microbiota in intestinal health and disease. Nat Rev Gastroenterol Hepatol 7(9):503–14.
- Child, M.W., Kennedy, A., Walker, A.W., Bahrami, B., Macfarlane, S., Macfarlane, G.T. (2006). Studies on the effect of system retention time on bacterial populations colonizing a three-stage continuous culture model of the human large gut using FISH techniques. FEMS Microbiol Ecol 55(2):299–310.
- Macfarlane, G.T., Macfarlane, S., Gibson, G.R. (1998). Validation of a Three-stage compound continuous culture system for investigating the effect of retention time on the ecology and metabolism of bacteria in the human colon. Microb Ecol 35(2):180–7.
- Molly, K., Vande, W.M., Verstraete, W. (1993). Development of a 5-step multi-chamber reactor as a simulation of the human intestinal microbial ecosystem. Appl Microbiol Biotechnol 39(2):254–8.
- Possemiers, S., Verthe, K., Uyttendaele, S., Verstraete, W. (2004). PCR-DGGE-based quantification of stability of the microbial community in a simulator of the human intestinal microbial ecosystem. FEMS Microbiol Ecol 49(3):495–507.
- Macfarlane, G.T., Macfarlane, S., Gibson, G.R. (1998). Validation of a three-stage compound continuous culture system for investigating the effect of retention time on the ecology and metabolism of bacteria in the human colon. Microb Ecol 35(2):180–7.
- Martoni, C., Bhathena, J., Jones, M.L., Urbanska, A.M., Chen, H., Prakash, S. (2007). Investigation of microencapsulated BSH active lactobacillus in the simulated human GI tract. J Biomed Biotechnol 2007(7):13684.
- Bhathena, J., Kulamarva, A., Martoni, C., Urbanska, A.M., Prakash, S. (2008). Preparation and in vitro analysis of microencapsulated live lactobacillus fermentum 11976 for augmentation of feruloyl esterase in the gastrointestinal tract. Biotechnol Appl Biochem 50(Pt 1):1–9.
- McConnell, E.L., Fadda, H.M., Basit, A.W. (2008). Gut instincts: Explorations in intestinal physiology and drug delivery. Int J Pharm 364(2):213–26.
- Gill, S.R., Pop, M., Deboy, R.T., Eckburg, P.B., Turnbaugh, P.J., Samuel, B.S., Gordon, J.I., Relman, D.A., Fraser-Liggett, C.M., Nelson, K.E. (2006). Metagenomic analysis of the human distal gut microbiome. Science 312(5778): 1355–9.
- Hartemink, R., Kok, B.J., Weenk, G.H., Round, J.L. (1996). Raffinose-Bifidobacterium (RB) agar, a new selective medium for bifidobacteria. J Microbiol Methods 27:33–43.
- Hartemink, R., Domenech, V.R., Rombouts, F.M. (1997). LAMVAB - A new selective medium for the isolation of lactobacilli from faeces. J Microbiol Methods 29:77–84.
- Muyzer, G., de Waal, E.C., Uitterlinden, A.G. (1993). Profiling of complex microbial populations by denaturing gradient gel electrophoresis analysis of polymerase chain reaction-amplified genes coding for 16S rRNA. Appl Environ Microbiol 59(3):695–700.
- Rinttila, T., Kassinen, A., Malinen, E., Krogius, L., Palva, A. (2004). Development of an extensive set of 16S rDNA-targeted primers for quantification of pathogenic and indigenous bacteria in faecal samples by real-time PCR. J Appl Microbiol 97(6):1166–77.
- Satokari, R.M., Vaughan, E.E., Akkermans, A.D., Saarela, M., de Vos, W.M. (2001). Bifidobacterial diversity in human feces detected by genus-specific PCR and denaturing gradient gel electrophoresis. Appl Environ Microbiol 67(2): 504–13.
- Sekirov, I., Russell, S.L., Antunes, L.C., Finlay, B.B. (2010). Gut microbiota in health and disease. Physiol Rev 90(3):859–904.
- O’Hara, A.M., Shanahan, F. (2006). The gut flora as a forgotten organ. EMBO Rep 7(7):688–93.
- FAO/WHO. (2001). Health and nutritional properties of probiotics in food including powder milk with live lactic acid bacteria. Report of a Joint FAO/WHO Expert Consultation on Evaluation of Health and Nutritional Properties of Probiotics in Food Including Powder Milk with Live Lactic Acid Bacteria. Basel, Switzerland: World Heatlh Organization.
- Kleerebezem, M., Vaughan, E.E. (2009). Probiotic and gut lactobacilli and bifidobacteria: Molecular approaches to study diversity and activity. Annu Rev Microbiol 63:269–90.
- Hormannsperger, G., Haller, D. (2010). Molecular crosstalk of probiotic bacteria with the intestinal immune system: Clinical relevance in the context of inflammatory bowel disease. Int J Med Microbiol 300(1):63–73.
- Gill, H., Prasad, J. (2008). Probiotics, immunomodulation, and health benefits. Adv Exp Med Biol 606:423–54.
- Kalliomaki, M., Salminen, S., Isolauri, E. (2008). Positive interactions with the microbiota: Probiotics. Adv Exp Med Biol 635:57–66.