Abstract
Abstract: In the present work we have attempted to develop a novel transmucosal patch of diclofenac sodium using electrospun polycaprolactone (PCL) nanofibers. PCL nanofibers were prepared by electrospinning techniques using different polymer concentrations. Nanofibers formulations were characterized by SEM, FTIR, drug loading, and in-vitro release study using Franz diffusion cell. Studies revealed the nanofibers fabricated from 13% PCL were fracture-free, non-beaded, and ultrafine with 120 nm diameter. Release studies demonstrated the sustained release behavior of PCL nanofibers. Nanofibers can be exploited for transmucosal drug delivery of NSAID with improved therapeutic efficacy.
INTRODUCTION
Pain is a situation where the patient needs immediate as well as sustained relief. The available conventional formulations of diclofenac sodium are unsuccessful, particularly in situations like toothache. The oral formulations of diclofenec sodium lead to local GI toxicity, ranging from minor gastric discomfort to ulceration and bleeding of the mucosa, depending upon the dose and duration of treatment. Therefore, in recent years several approaches, such as iontophoresis, microneedles, infusion pumps, transdermal delivery systems, and intramuscular delivery systems, have been conducted to overcome gastric toxicity associated with oral delivery of diclofenac sodium. Irrespective of extensive research in this area, diclofenac-sodium-based delivery systems still suffer from many drawbacks, including the inability to effectively penetrate through skin, leading to poor bioavailability after transdermal administration. Furthermore, intramuscular and pulmonary approaches result in systemic toxicity [Citation1, Citation2]. Therefore, the aim of this study was to develop a transmucosal drug delivery system using electrospun nanofibers, which would prove superior in terms of patient compliance and improved therapeutic efficacy. Nanofibers synthesized from biodegradable polymers have all the desired properties required for a sustained drug delivery system. Moreover, polymeric nanofibers can be easily fabricated into different nanoscales using electrospinning [Citation3, Citation4]. However, their utilization is still limited to biomedical and industrial applications [Citation5]. Electrospun nonwoven nanofibers, because of their high porosity and high surface area per unit mass can be utilized for safe and effective drug delivery [Citation6, Citation7]. In the present work we have studied the potential of polymeric nanofibers as a transmucosal carrier for diclofenac sodium. Nano-architecture of polymeric nanofibers makes the carrier system appropriate for both immediate as well as prolonged therapeutic action. The drug delivery can be terminated at any point without the need of trained personnel. Moreover, we have a long list of safe, biodegradable, biocompatible, bioadhesive polymers, which can be utilized depending upon the required release behavior and physicochemical properties of the drug. Polycaprolactone (PCL) was selected for the preparation of nanofibers [Citation8]. Physical properties of electrospun nanofibers depend on various process parameters, such as voltage, rpm of collector, distance between jet and collector, flow rate, and formulation parameters such as selection of polymer, viscosity, and surface tension [Citation9]. In the present study, the aforesaid parameters were optimized to developed nanofibers of desired characteristics.
MATERIALS AND METHODS
Materials
Polycaprolactone (molecular weight of 80,000 g/mol) was purchased from Sigma-Aldrich Pvt. Ltd., USA. Diclofenac sodium was purchased from Rankem Ltd., New Delhi, India. All other chemicals were of analytical grade.
Preparation of Nanofibers
Nanofibers were prepared by electrospinning technique as reported by Martinová et al., with slight modification depending upon the selected polymer polycaprolactone [Citation9, Citation10]. In brief, different polymeric (PCL) solutions ranging from (8-13%w/v) were prepared using a mixture of acetone and tetrahydrofuran (THF, 1:1). The polymeric solutions were then subjected to ultrasonication for 30 min to achieve a homogeneous dispersion. The dispersion so formed was injected through a 2ml syringe under controlled stirring conditions. The diameter of the ejected fibers from the syringe containing the polymer solution was significantly reduced as they traveled toward the collector placed on the rotating drum. The applied voltage was kept constant (10kV) throughout the study. The nanofibers so formed were collected on an aluminum sheet.
CHARACTERIZATION OF DEVELOPED FORMULATIONS
Morphology
The morphology and surface appearance of the nanofibers were examined by scanning electron microscopy (SEM). The completely dried samples were coated with a thin gold layer and observed with a scanning electron microscope (Jeol JSM- 6100, JEOL BV, Schiphol-Rijk, Netherlands).
Drug Loading
The various nanofiber (1cmX 1cm) formulations were placed in different drug solutions ranging from (0.5-2% w/v) for 30 minutes. After 30 minutes, the nanofibers were dried in a vacuum drier. The dried nanofibers were stored in an airtight container. For the estimation of loading efficiency, nanofibers were sonicated under a bath sonicator for 10 minutes and filtered using a 0.2μm membrane filter. The absorbance of the filtrate was taken using UV-spectroscopy at 284 nm. Loading efficiency (Eq. 1) and loading capacity (Eq. 2) were calculated using the following formulas.
In-vitro Release Study
The drug releases from different formulations were determined at pH 7.4 using a Franz Diffusion Cell. The drug-loaded formulation was placed in a donor compartment and the receptor compartment was filled with release medium. Samples were collected from the sampling port at different time intervals and replenished again with phosphate buffer after every sampling. The cell was placed in a water bath to maintain at 37°C throughout the release study.
Statistical Analysis
All data were expressed as mean ± standard deviation. Significance was tested using a Dunnett test, i.e. comparison of all the formulations vs. the blank. A p value less than 0.05 was considered to indicate statistical significance for all the comparisons.
RESULTS AND DISCUSSION
Characterization of Developed Formulations
The physical properties and fiber diameter were strongly dependent on spinning conditions and in turn influenced the loading efficiency and release behavior of the developed nanofibers formulations. Similar results were also reported by Reneker et al. [Citation9]. The nanofibers obtained from 13% polymer concentration with a flow rate of 0.5 ml/hr and 1800 rpm of collector speed were found to have an optimized spinning condition for nanofibers’ fabrication (). SEM studies () revealed that the nanofibers developed with 13% PCL were ultrafine, fracture-free, uniform, and nonwoven fibers of 120nm diameter. However, 8% and 10% polymer concentration with specified electrospinning conditions produced non-uniform, beaded, and thick fibers. This consequence attributed an anomalous relationship between the rate of extrusion and rate of evaporation during the nanofibers’ formation. Except for the spinning conditions, the physical properties of polymeric solutions like viscosity, surface tension, and conductivity also play an important role, which determines the rate of extrusion under applied electrical field of 10Kv [Citation10–13]. In the case of 13% PCL, the applied electrical field was found to be just enough to maneuver the resistance associated with fluidity (surface tension and viscosity). Moreover, 13% PCL seems to produce a validated physical state in terms of viscosity, surface tension, and conductivity, which control the rate of extrusion under the applied electrical field of 10Kv. This makes the polymer solution spin and stretch sufficiently between jet and collector, resulting in the formation of ultrafine nanofibers. Nanofibers’ quality in 13% PCL further indicated that an equilibrium state was established between the rate of extrusion and rate of evaporation. Collector speed of 1800 RPM was found to be optimized with respect to fiber formation and its woven process. 8% PCL with 1.5ml/hr flow rate under specified spinning conditions was unable to produce the desired nanofibers, as it possesses higher electrical conductivity due to higher solvent proportion than 10% and 13% PCL solutions, leading to higher extrusion under the applied electrical field. The extrusion rate was not in equilibrium with spinning conditions, resulting in the formation of beaded and uneven nanofibers. Uneven nanofibers’ fabrication with 10% PCL indicated that the collector speed was not optimized with respect to nanofiber production. Moreover, 8% and 10% PCL with 0.5 ml/hr flow rate resulted in thick nanofibers, showing the amount of solvent present in the extruded material at the ejected point was unable to evaporate properly while approaching the collectors grid, thus leaving a high proportion of liquid core in the developed nanofibers. A further increase in polymer concentration up to 15% was found to result in erratic extrusion under specified spinning conditions and thus this parameter was obsolete for further studies.
Figure 1. Scanning electron microscopic images of the nanofibers using polymer concentrations of: (a) 13% w/v; (b) 10 %w/v; (c) 8 % w/v.
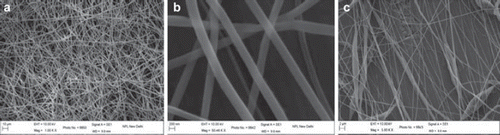
Table 1. Optimized conditions of nanofiber.
Loading Efficiency
The loading capacity of all the developed nanofiber formulations was determined and the results are listed in . As stated earlier, drug loading depends on the physical properties of the nanofibers. Results indicated a higher loading capacity with 13% PCL at a drug concentration of 1mg/ml. The above results were attributed, as 13% PCL with specified spinning conditions yielded uniform, dense, and ultrafine nanofibers with 120nm diameter. Higher drug loading with 13% PCL further demonstrated a higher nanofiber density per unit area and also indicated the presence of a larger void space, which helps to hold a substantial drug fraction. There was a significant decrease in loading efficiency as well as loading capacity on increasing the drug concentration above 1 mg/ml in all the formulations. It may be attributed to the fact that a drug concentration of 1 mg/ml is sufficient to establish equilibrium with the available surface area per unit volume of nanofibers. In contrast, 8 and 10% PCL formulations resulted in uneven, beaded, and non-uniform fiber fabrication. As a consequence, these nanofiber formulations possess a poor drug holding capacity and, in turn, a poor loading capacity. FTIR studies clearly indicated that there was no interaction between drug and polymer. Furthermore, the conditions selected for the loading process were found to be conducive for optimum drug loading and formulation stability ().
Figure 2. FTIR spectra of Diclofenac, showing drug-loaded nanofiber and plain polymer, respectively, from the bottom.
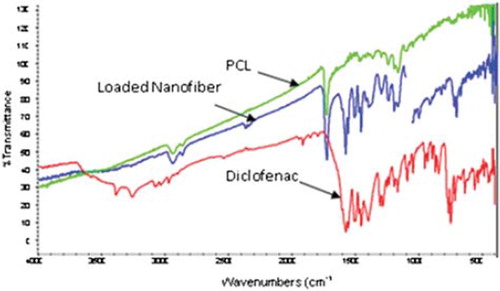
Table 2. Loading capacity of different formulation at different drug concentrations.
Drug Release
The release profile indicated that all the formulations () showed initial burst release followed by a steady state. Initial burst release was attributed to larger surface area associated with developed nanofibers. Afterwards a steady state release behavior was governed by erosion and capillary diffusion mechanism. Release behavior observed in 8% and 10% nanofiber formulations was almost superimposed with minor deviations. However, 13% PCL formulations indicated 38% release after 6 hrs in contrast to 27 and 30 % release from 8 and 10%, respectively. These results were so attributed because 13% PCL formulations have a larger surface area than 8 and 10% PCL formulations. The controlled and steady state release behavior observed in 13% PCL formulation after 8 hrs indicated drug release governed by a diffusion mechanism through the fine capillary from a dense nanofiber network. However, at this stage 8 and 10 % formulations demonstrated second release maxima, which is not only attributed to poor fiber network and fiber density per unit volume of nanofibers but also indicated the dual drug release mechanism such as diffusion and erosion processes. After 24 hrs, results indicated that all the nanofiber formulations showed almost 90% release. The above results clearly indicated that 13% PCL formulations showed an initial burst release followed by steady and controlled release, which is associated with the fiber diameter and complexity in the fiber network.
CONCLUSION
Electrospinning can be successfully used for the fabrication of nanofibers. The quality of nanofibers strongly depends upon spinning conditions and physical properties of the polymer. Nanofiber properties can be further modified into porous, coated, polymeric composites to explore various biomedical applications. Drug loading and release from the nanofiber could be controlled depending upon fiber density and complexity in the fiber network. In the present study, an electrospinning technique was successfully employed to develop a transmucosal patch of diclofenac sodium using biodegradable nanofibers for toothache, which would prove superior in terms of safety and therapeutic efficacy. This technology can be further extended to develop a novel nanocarrier system for pharmaceuticals depending upon their biomedical utility.
Declaration of interest: The authors report no conflicts of interest. The authors alone are responsible for the content and writing of the paper.
REFERENCES
- Rainsford, K.D., Kean, W.F., Ehrlich, G.E. 2008. Review of the pharmaceutical properties and clinical effects of the topical NSAID formulation, diclofenac epolamine. Curr Med Res Opin. 24(10): 2967–92.
- Piao, H, Kamiya, N, Watanabe, J, Yokoyama, H, Hirata, A, Fujii, T, Shimizu, I, Ito, S, Goto, M. 2006. Oral delivery of diclofenac sodium using a novel solid-in-oil suspension. Int J Pharm. 313(1-2): 159–62.
- Baumgarten, P.K. 1971. Electrostatic spinning of acrylic microfibers. J Colloid Interf Sci. 36: 71–79.
- Larronda, L, Manley, R.S. 1981. Electrostatic fiber spinning from polymer melts. I. Experimental observations on fiber formation and properties. J Polym Sci, Part B: Polym Phys. 19: 909–20.
- Hong, K.H., Park, J.L., Sul, H.I., Youk, J.H., Kang, T.J. 2006. Preparation of antimicrobial poly(vinyl alcohol) nanofibers containing silver nanoparticles. J Polymer Sci Part B: Polymer Physics 44: 2468–2474.
- Doshi, J, Reneker, D.H.J. 1995. Electrospinning process and application of electrospinning fibers. Electrostat35: 151–60.
- Gibson, P.W., Lee, C.K.F., Reneker, D. 2007. Application of nanofiber technology to nonwoven thermal insulation. J Eng Fiber Fabric 2: 32–37.
- Nottelet, B, Mandracchia1, D., Pektok, E, Walpoth, B, Gurny, R, Moller, M. 2007. Electrospinning of drug loaded poly (ε caprolactone) nanofibers: In vivo evaluation of novel degradable small-sized vascular grafts. Eur Cell Mater. 13: 1473–2262.
- Reneker, D.H., Chun, I. 1996. Nanometer diameter fibers of polymer produced by electrospinning. Nanotechnology 7: 216–23.
- Fang, X, Reneker, D.H.J. 1997. DNA fibers by electrospinning. Macromol Sci Phys. 36:169–73.
- Fong, H, Chun, I, Reneker, D.H. 1999. Beaded nanofibers formed during electrospinning. Polymer40: 4585–92.
- Jaeger, R, Bergshoef, M, Batlle, C, Schonherr, H, Vancso, J. 1998. Electrospinning of ultra-thin polymer fibres. J Macromol Symp. 127: 141–50.
- Jaeger, R, Schonherr, H, Vancso, J. 1996. Chain packing in electro-spun PEO visualized by AFM. Macromolecules 29: 7634–6.