Abstract
A composite electrode was prepared by modifying glassy carbon micro particles with gold nanoparticles and glucose oxidase enzyme for glucose detection. In terms of analytical characteristics, a linearity was obtained in the concentration range between 50.0 and 1000.0 µM with equation of y = 0.0015x + 0.2336 with the correlation coefficient of R2 = 0.9801. R.S.D. value was calculated for 400 µM glucose (n = 6) and found to be 4.89%. LOD and LOQ values were also calculated and revealed to be 52.9 µM and 176.3 µM, respectively. The developed system was also applied for detection of glucose in four types of alcoholic beverages.
Carbon is known as an ideal electrode substrate (Anık-Kırgöz et al. Citation2006a), which is widely used in electroanalysis due to low background current, wide potential, chemical inertness, low cost, and suitability for various sensing and detection applications (Wang et al. Citation2001). In addition, carbon electrodes exhibit fast response and can easily be fabricated in different configuration and sizes (Anık-Kırgöz et al. Citation2004, Citation2006a, Citation2006b, Wang et al. Citation2001, Çubukçu et al. Citation2007, Timur et al. Citation2007, Anık and Çubukçu Citation2008, Anık and Çevik Citation2009, Anik et al. 2010a). There are several carbon electrode forms which are applied in electroanalytical applications, such as glassy carbon electrode (GCE) and carbon paste electrode (CPE). Other newly developed alternative carbon electrodes are the rigid carbon-polymer composite based electrode, graphite epoxy composite electrode (GECE), and composite electrode material based on mixing glassy carbon (GC) micro particles with an oil binder, glassy carbon paste electrode (GCPE) (Anık-Kırgöz et al. 2006a, Wang et al. Citation2001). GECE allows easy adaptation to a variety of electrode configurations because of its flexibility in size and shape (CitationAnık-Kırgöz et al. 2005, Citation2006a, Citation2006b, Citation2007, Tallman and Peterson Citation1990, Cespedes et al. Citation1996, Citation2000, Alegret et al. Citation1999, Saurina et al. Citation1999, Serradell et al. Citation2002, Alegret and Merkoçi Citation2003). Its surface can be smoothed or polished to provide fresh active material ready to be used in a new assay (Anık-Kırgöz et al. 2006b). GCEs provide an attractive electrochemical reactivity, negligible porosity, and good mechanical rigidity (Wang et al. Citation2001, McCreery Citation1991, Van der Linden and Dieker Citation1980), while the CPEs have been popular due to their very low background current, composite nature, and ease of modification, renewal, and miniaturization (Wang et al. Citation2001, Rice et al. Citation1983, Kalcher et al. Citation1995). GCPEs have been utilized by our group since their usage for the preparation of new electrode material, because they offer high electrochemical reactivity, a wide potential window with a low background current and they are inexpensive, easy to prepare, modify, and renew. Moreover it has been demonstrated that this electrode has better electrochemical reactivity towards the oxidation of hydrogen peroxide compared to conventional CPE (Wang et al. Citation2001, Çubukçu et al. 2007).
On the other hand, Au-np is a kind of nanomaterial where it can help proteins to retain their biological activity upon adsorption (Çubukçu et al. 2007, Doron et al. Citation1995, Brown et al. Citation1996). Its large surface area and good electronic properties (Liu et al. Citation2007), and its utilization for the study of direct electron transfer of redox proteins (Liu et al. Citation2007, Ju et al. Citation2002, Xiao et al. Citation2000) increase its usage in biosensor construction (Xiao et al. Citation2000, Liu and Ju Citation2002, Gu et al. Citation2001).
Various kinds of sensors and biosensors have been constructed by using Au-nps by Pingarron and his group. For example, they prepared glucose oxidase (GOx) biosensors by immobilizing GOx onto different tailored Au-nps modified electrode surfaces (Mena et al. Citation2005). The same group also determined hypoxanthine (Hx) via xanthine oxidase (XOD) based CPE modified with electrodeposited Au-nps (Agüi et al. Citation2006).
In our previous work, GCPE was modified with XOD enzyme and Au-nps to obtain xanthine (X) and Hx biosensor and very promising results were obtained. From that result, it was concluded that, especially for Hx detection, a more practical and efficient biosensor has been obtained (Çubukçu et al. 2007). This can be explained by the composite electrode that contains GC micro particles with Au-np. Au-np catalyzes the enzymatic reaction by allowing direct electron transfer between the electrode and enzyme active center by means of the conducting tunnels of gold nanocrystals (Mena et al. Citation2005). On the other hand, the combination of these two materials into a composite structure, rather than usage of Au-np as an immobilizing reagent, may cause the immobilized enzyme to have higher bioactivity that results in fast, stable, and sensitive responses to the concerning substrate (Çubukçu et al. 2007). In the literature there are not many papers corresponding to biosensors for glucose determination, especially in alcoholic beverages. Most of them deal with the alcohol determination while very few contain glucose determinations in alcoholic beverages (Grassino et al. Citation2010, Antiochia and Gorton Citation2007, Tkac et al. Citation1999). In this point of view, we believe that this work will widen the scope of glucose detection in beverages. For this purpose, in the present work, GCPE was modified with Au-nps and then with GOx enzyme and, as a result, a suitable and practical biosensor for glucose detection was obtained. After the optimization of experimental parameters like enzyme amount, Au-np amount, working potential and pH, analytical characteristics were investigated. Then, for examining and testing the performance of a newly developed biosensing system, it was applied for glucose detection in four different types of alcoholic beverages including red and white wine, draft beer, and mint liqueur.
Experimental
Reagents and chemicals
GC spherical powder, 2–12 micron, 99.95% metal basis was purchased from Aldrich, while mineral oil was obtained from Sigma-Aldrich and gold colloid from Sigma (0.75A520 units/mL; 10 nm ∼0.001% as HAuCl4). 0.01 M glucose stock solution was prepared freshly by dissolving the appropriate amount of glucose (D-(+) glucose monohydrate from Merck). GOx, Type II-S: from Aspergillus niger G6641-50KU, 20% protein, balance primarily potassium gluconate, was purchased from Sigma. Phosphate buffer solution KH2PO4 (Merck/purity 99,995%, 0.05 M, pH 7.0) was served as the supporting electrolyte. All solutions were prepared using double distilled water and all other used chemicals were of analytical grade.
Apparatus
Chronoamperometric measurements were carried out with the FRA 2 µ-AUTOLAB Type III electrochemical measurement system from ECO CHEMIE Instruments B.V. (Netherlands) driven by GPES software. The experiments were conducted in a 10 mL voltammetric cell (Metrohm), at room temperature (25°C), using a three-electrode configuration. A platinum electrode served as an auxiliary electrode while an Ag/AgCl electrode as a reference electrode, and Au-np/GOx/GCPE was used as the working electrode. The electrodes were inserted into the cell through the Teflon cover.
Electrode preparation
Au-np/GOx/GCPE was prepared by hand mixing of 80:20 (% w/w) GC spherical powder/mineral oil with 55.7 Unit GOx and 2 µL Au-np. A portion of the resulting paste was then packed firmly into the electrode cavity (3.0 mm diameter and 5.0 mm depth) of a PTFE sieve where electrical contact was established via a copper wire. The resulting electrode surface was then dried at room temperature for about 5 min. The surface of the resulting paste electrodes was smoothed and rinsed carefully with double distilled water.
Procedure
Prior to its use, the surface of the composite electrode was smoothed with a weighing paper. Then three electrodes were immersed into the 10 mL electrochemical cell. Chronoamperometric measurements were carried out in 50.0 mM phosphate buffer (pH 7.5) medium under the operating potential of + 800 mV while the solution was stirred. The duration of each analysis was 400 s and the transient current decayed to a steady-state value after 200 s in the presence of supporting electrolyte. The electrode surface was renewed before the beginning of every measurement
Sample application
The developed biosensor was used for the glucose analysis in four different kinds of alcoholic beverages. These samples were analyzed using the standard addition method. A known amount of glucose (400 µM) was added to the reaction cell from the stock solution that was prepared by dilution of (1:100) beverages including white and red wine, draft beer, and mint liqueur with 50 mM phosphate buffer (pH 7.5). Amperometric measurements in stirred solutions with Au-np/GOx/GCPE were performed after transferring the corresponding analytical solution to the electrochemical cell with a potential of + 800 mV versus Ag/AgCl. Each analysis was performed three times and the results are given in . The recovery values of the analytical signal for the diluted samples were calculated and also demonstrated in the same table (n = 3).
Table I. Recovery data of glucose detection in alcoholic beverages obtained with the Au-np/GOx/GCPE by using chronoamperometric method.
Results and Discussion
Optimization of experimental parameters
Effect of Enzyme Amount
The effect of enzyme amount (39.8, 47.8, 55.7, 71.6, 79.6 unit) on obtained current values was investigated for 400 µM glucose and shown in . As can be seen from , current values increase up to 55.7 unit enzyme and then a sharp decrease was observed for higher enzyme amount, which can be attributed to the diffusion problem (Anik et al. Citation2010b). The excess amount of protein might limit the efficient substrate-enzyme interaction that is required for this reaction. Since the best results were obtained with the 55.7 unit, further experiments were conducted with this amount.
Effect of Au-np Amount
demonstrates the effect of Au-np amounts (0.5, 1, 2, 5, 10 µL) on the current response of 400 µM glucose. As can clearly be seen from the figure, the current increases from 0.5 to 2 µL and then a slight decrease is obtained for volumes greater than 2 µL. This decrease can be attributed to a decrease in GC-µ particle amount in the electrode structure due to the increase in Au-np amount (Çubukçu et al. 2007).
Effect of pH
The effect of pH on the electrode response was investigated by using phosphate buffer systems (50.0 mM) between pH 6.5 and 8.0 with an increment of 0.5 for 400 µM glucose (). As can be seen from (B), the current value of the enzyme electrode increases significantly from pH 6.5 to 7.5, and then a decrease is obtained at pH values higher than 7.5. As a result, pH 7.5 was chosen as optimum pH for further studies.
Figure 3. Chronoamperometric responses of 400 µM glucose on the biosensor response at various pH: (a) background; (b) 6.0; (c) 6.5; (d) 7.0; (e) 7.5; (f) 8.0; conditions: phosphate buffer solution system, applied potential + 0.9 V, 55.7 unit GOx, 2 µL Au-np; Inset: the effect of optimum pH curve of Au-np/GOx/GCPE.
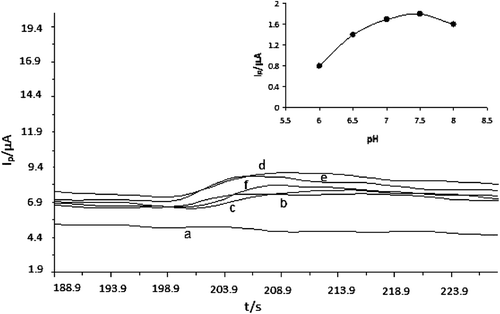
Effect of Working Potential
In order to examine the effect of working potential, potentials between + 500 and + 1000 mV with increment of 100 mV for 400 µM glucose solution was applied to the Au-np/GOx/GCPE. As can be seen from , for the developed electrode, only potentials between + 700 mV to + 1000 mV give the current values. When this region was examined, it can be seen that the current values increase from + 700 mV to + 800 mV, and then a slight decrease is observed for higher potentials. Thus, + 800 mV was taken as optimum working potential and used for further studies.
Analytical characteristics
After the optimization of experimental parameters, a linear relationship between biosensor response and glucose concentration was obtained in the concentration range between 50–1000 µM with the equation y = 0.0024x-0.0651 and correlation coefficient, R2 = 0.9802 (). RSD values were calculated for 400 µM (n = 6) and found to be 4.89%. LOD and LOQ values, on the other hand, were found to be 52.9 µM and 176.3 µM, respectively, based on the formulations of 3s/m (for LOD) and 10s/m (for LOQ), where m is the slope of the calibration curve (at decreased concentration) and s is the standard deviation of the blank current values.
Figure 5. Calibration graph for glucose at Au-np/GOx/GCPE conditions: in 50 mM phosphate buffer solution (pH 7.5) and working potential + 0.8 V, 25°C.
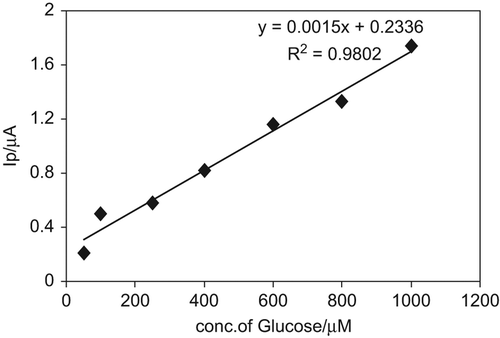
On the other hand, the storage stability of the biosensor is a very important parameter for long-term applications. It was observed that when the newly developed biosensor was stored at + 4°C during a week, activity remained stable. During this stage 27 measurements were carried out and no decrease was detected in the signal response. The effect of ascorbic acid (AA) on the current response was also investigated. The measurements were conducted using 50 μM glucose. It was revealed that up to 100 μM AA, it is possible to detect glucose properly.
Sample application
Four different, commercially available alcoholic beverages were analyzed using the standard addition method. A known amount of glucose (400 μM) was added to the reaction cell from the stock solution that was prepared by diluting the (1:100) alcoholic beverage sample with 50 mM phosphate buffer (pH 7.5). The recovery values of the analytical signal for each of the diluted samples were calculated and are shown in . From the recovery values, it can be concluded that the developed biosensor can be utilized for glucose detection even in complicated matrix systems like beer, wine, and mint liqueur. When examined carefully, apart from the white wine, nearly 100 ± 5.00% recovery values were observed for other beverages. These values are in acceptable limits, demonstrating the applicability of the developed system for detection of glucose in alcoholic beverages. Also, using this biosensor renders the laborious sample treatment step that is commonly applied before the measuring step in alternative methods, such as spectroscopy and chromatography.
Conclusions
In this paper, a glucose nanocomposite biosensor was constructed simply by mixing the proper amount of GOx, Au-np, and GC micro particles and mineral oil. Resulting Au-np/GOx/GCPE provides easy to prepare and useful biosensors for glucose detection in alcoholic beverages. The combination of GOx into a nanocomposite structure with corporation of Au-np enables us to keep the enzyme with higher bioactivity and stability in the operational conditions and results in fast, stable, and sensitive responses in glucose biosensing (Çubukçu et al. 2007). On the other hand, data obtained from the sample application part showed that biosensing responses were not affected by the sample matrix and the nature. Compared to other glucose biosensors in the literature, this system offers practical, precise, and robust detection of glucose in real samples.
In the literature there are not many papers corresponding to biosensors for glucose determination, especially in alcoholic beverages. Most of them deal with the alcohol determination while very few contain glucose determinations in alcoholic beverages. Hence this work will widen the scope of glucose detection in beverages. On the other hand, usage of nanomaterial in the biosensor structure strengthened the effect of the system and, as a result, more robust biosensor was obtained.
Declaration of interest
The authors report no conflicts of interest. The authors alone are responsible for the content and writing of the paper.
References
- Agüi L, Manso J, Yanez-Sedeno P, Pingarron JM. 2006.Amperometric biosensor for hypoxanthine based on immobilized xanthine oxidase on nanocrystal gold–carbon paste electrodes.Sens. Actuators B.113:272–280.
- Alegret S, Fabregas E, Cespedes F, Merkoçi A, Sole S, Albareda M, Pividori MI. 1999.The strategy of renewing biosensor surface: Past, present and future: A review.Química Analitica18:23–29.
- Alegret S, Merkoçi A. 2003.Integrated analytical systems, Elsevier, Amsterdam, 377–412.
- Anık Ü, Çevik S. 2009.Double-walled carbon nanotube based carbon paste electrode as xanthine biosensor.Microchim Acta.66: 209–213.
- Anık Ü, Çevik S, Pumera M. 2010a.Effect of nitric acid ‘‘washing’’ procedure on electrochemical behavior of carbon nanotubes and glassy carbon µ-particles.Nanoscale Res Lett. 5:846–852.
- Anık Ü, Çubukçu M. 2008.Examination of the electroanalytic performance of carbon nanotube (CNT) modified carbon paste electrodes as xanthine biosensor transducers.Turk. J. Chem.32:711–719.
- Anık Ü, Çubukçu M, Çevik S, Timur S. 2010b.Usage of bismuth film electrode as biosensor transducer for alkaline phosphatase assay.Electroanalysis22:1519–1523.
- Anık-Kırgöz Ü, Marín S, Pumera M, Merkoçi A, Alegret S. 2005.Stripping voltammetry with bismuth modified graphite-epoxy composite electrodes.Electroanalysis17:881–886.
- Anık-Kırgöz Ü, Odacı D, Timur S, Merkoçi A, Pazarlıoğlu N, Telefoncu A, Alegret S. 2006a.Graphite epoxy composite electrodes modified with bacterial cells.Bioelectrochemistry69:128–131.
- Anık-Kırgöz Ü, Odacı D, Timur S, Merkoçi A, Alegret S, Besşün N, Telefoncu A. 2006b.A biosensor based on graphite epoxy composite electrode for aspartame and ethanol detection.Analytica Chimica Acta570:165–169.
- Anık-Kırgöz Ü, Timur S, Wang J, Telefoncu A. 2004.Xanthine oxidase modified glassy carbon paste electrode.Electrochemistry Commun6:913–916.
- Anık-Kırgöz Ü, Timur S, Odacı D, Perez B, Alegret S, Merkoçi A. 2007.Carbon nanotube composite as novel platform for microbial biosensor.Electroanalysis19:893–898.
- Antiochia R, Gorton L. 2007.Development of a carbon nanotube paste electrode osmium polymer-mediated biosensor for determination of glucose in alcoholic beverages.Biosensors and Bioelectronics22:2611–2617.
- Brown KR, Fox AP, Natan MJ. 1996.Morphology-dependent electrochemistry of cytochrome c at Au colloid-modified SnO2 electrodes.J. Am. Chem. Soc.118:1154–1157.
- Cespedes F, Alegret S. 2000.New materials for electrochemical sensing II. Rigid carbon–polymer biocomposites.Trends Anal Chem. 19:276–285.
- Cespedes F, Fabregas E, Alegret S. 1996.New materials for electrochemical sensing I. Rigid conducting composites.Trends Anal Chem.15:296–304.
- Çubukçu M, Ertaş FN, Anık Ü. 2012.Metal/metal oxide micro/nanostructured modified GCPE for GSH detection.Current Analytical Chemistry (in press).
- Çubukçu M, Timur S, Anık Ü. 2007.Examination of performance of glassy carbon paste electrode modified with gold nanoparticle and xanthine oxidase for xanthine and hypoxanthine detection.Talanta74:434–439.
- Doron A, Katz E, Willner I. 1995.Organization of Au colloids as monolayer films onto ITO glass surfaces: application of the metal colloid films as base interfaces to construct redox-active monolayers.Langmuir11:1313–1317.
- Grassino AN, Milardović S, Grabarić Z, Grabarić BS. 2010.Simple and reliable biosensor for determination of glucose in alcoholic beverages.Food Research International, doi: 10.1016/j.foodres.2011.10.004.
- Gu HY, Yu AM, Chen HY. 2001.Direct electron transfer and characterization of hemoglobin immobilized on an Au colloid–cysteamine-modified gold electrode.J. Electroanal. Chem.516:119–126.
- Ju HX, Liu SQ, Ge B, Lisdat F, Scheller FW. 2002.Electrochemistry of cytochrome c immobilized on colloidal gold modified carbon paste electrodes and its electrocatalytic activity.Electroanalysis14:141–147.
- Kalcher K, Kauffmann J M, Wang J, Svancara I, Vytras K, Neuhold C, Yong Z. 1995.Sensors based on carbon paste in electrochemical analysis: A review with particular emphasis on the period 1990–1993.Electroanalysis7:5–22.
- Liu SQ, Ju HX. 2002.Renewable reagentless hydrogen peroxide sensor based on direct electron transfer of horseradish peroxidase immobilized on colloidal gold-modified electrode.Anal. Biochem.307:110–116.
- Liu S, Wang L, Zhao F. 2007.Influence of gold nanoparticle modified electrode on the mediation reduction of ferricyanide by methylene blue.J. Electroanal. Chem.602:55–60.
- McCreery RL. 1991.Carbon electrodes structural effects on electron-transfer kinetics. Electroanalytical Chemistry, Vol. 18, Bard AJ (ed.), Dekker, New York.
- Mena ML, Ýãnez-Sedeño P, Pingarr ́on JM. 2005.A comparison of different strategies for the construction of amperometric enzyme biosensors using gold nanoparticle-modified electrodes.Anal. Biochem.336:20–27.
- Rice ME, Galus Z, Adams RN. 1983.Graphite paste electrodes: Effects of paste composition and surface states on electron-transfer rates.J. Electroanal. Chem.143:89–102.
- Saurina J, Hernandez-Casou S, Fabregas E, Alegret S. 1999.Determination of tryptophan in feed samples by cyclic voltammetry and multivariate calibration methods.Analyst124:733–737.
- Serradell M, Izquierdo S, Moreno L, Merkoçi A, Alegret S. 2002.Mercury-free PSA of heavy metals using graphite-epoxy composite electrodes.Electroanalysis14:1281–1287.
- Tallman DE, Peterson SL. 1990.Composite electrodes for electroanalysis: Principles and applications.Electroanalysis2:499–510.
- Timur S, Anık Ü, Odacı D, Gorton L. 2007.Development of a microbial biosensor based on carbon nanotube (CNT) modified electrodes.Electrochemistry Commun9:1810–1815.
- Tkac J, Svitel J, Sturdik E. 1999.Determination by biosensors of important substances in beverages.Chemicke Listy93:518–526.
- Van der Linden WE, Dieker JW. 1980.Glassy carbon as electrode material in electro- analytical chemistry.Analytica Chimica Acta119: 1–24.
- Wang J, Anık-Kırgöz Ü, Mo J.-W, Lu J, Kawde A. N, Muck A. 2001.Glassy carbon paste electrodes.Electrochemistry Commun3:203–208.
- Xiao Y, Ju HX, Chen HY. 2000.Direct electrochemistry of horseradish peroxidase immobilized on a colloid/cysteamine-modified gold electrode.Anal. Biochem.278:22–28.