Abstract
Hemoglobin-based oxygen carriers (HBOCs) have been developed as a transfusion alternative and oxygen therapy. The Hb source is usually outdated donated human blood or cow blood obtained from cattle industries because of its abundance. This study examined the feasibility of using swine Hb (SHb) for preparation of cellular-type HBOCs, hemoglobin-vesicles (HbV). Purification of SHb from fresh swine whole blood was conducted with processes including carbonylation (SHbO2 ◊ SHbCO), pasteurization (60 °C, 15 hours) and tangential flow ultrafiltration, with yield of 90%. Actually, differential scanning calorimetric analysis showed a denaturation temperature of SHbCO at 83 °C and assures its stability during pasteurization. Concentrated SHbCO together with pyridoxal 5’-phosphate (PLP) as an allosteric effector was encapsulated in phospholipid vesicles to prepare SHbV. After decarbonylation (SHbCO ◊ SHbO2), the oxygen affinity (P50) of SHb changes mainly by PLP, and the influence of Cl‐ was small, in a manner similar to that of human Hb (HHb). However, after encapsulation, vesicles of SHbV showed much lower oxygen affinity (higher P50) than HHbV did. Autoxidation of SHbV was slightly faster than HHbV. Although some differences are apparent in oxygen affinity and autoxidation rates, results clarified that SHb is useful as a starting material for HbV production.
Introduction
Hemoglobin-based oxygen carriers (HBOCs) have been developed for use as a transfusion alternative (Chang Citation2006) because transfusion presents problems of possible infection, blood-type mismatching, and a short storage period during refrigeration. It is expected that HBOCs can resolve these problems. Cell-free HBOCs are now under clinical trials. Some examples are intramolecularly crosslinked hemoglobin (Hb), polymerized Hb, and polyethylene glycol conjugated Hb (Natanson et al. Citation2008). The major remaining hurdle before clinical approval of this earliest generation of cell-free HBOCs is vasoconstriction and resulting hypertension, which are presumably attributable to the high reactivity of Hb with endothelium-derived nitric oxide (NO) (Olson et al. Citation2004, Yu et al. Citation2010). However, cellular Hb-vesicles (HbVs) that encapsulate concentrated Hb solution in phospholipid vesicles (Sakai et al. Citation2008, Tsuchida et al. Citation2009) induce neither vasoconstriction nor hypertension (Sakai et al. Citation2000).
Most HBOCs are made of human-derived Hb (HHb) that has been purified from outdated donated blood or placenta blood. Because of the limited sources of outdated donated blood, some groups use bovine Hb (BHb), which is purified from blood abundantly obtained from cattle industries. However, the concern of prion contamination remains. In Japan, the usage of biological raw materials derived from ruminant animals including cows is strictly regulated. Moreover, the possibility exists in some countries that religious reasons would hinder the usage of BHb. Another possibility would be use of swine Hb (SHb) (though it would also relate to religious restriction), because swine is not categorized as a ruminant animal. Structural similarity of SHb and human Hb (HHb) were confirmed from X-ray crystallography (Katz et al. Citation1994). In fact, usage of SHb was tested to prepare chemically modified Hb solutions (Lee et al. Citation1992, Chang et al. Citation2004, Barnikol et al. Citation2002, Zhang et al. Citation2011).
In this study, we tested whether SHb is applicable for the preparation of swine Hb-vesicles (SHbV) in terms of purification methods including pasteurization, encapsulation, and the fundamental chemical characteristics of the resulting swine HbV (SHbV) when compared with human Hb-vesicles (HHbV).
Materials and methods
Purification of SHb from swine whole blood
Purification procedures with carbonylation and tangential flow ultrafiltration were almost identical to those presented in our previous report describing preparation of BHb (Sakai et al. Citation2002). Swine fresh whole blood containing EDTA as an anticoagulant was obtained from Innovative Research Inc. (Michigan, USA). A total of 1004 g ([SHb] = 10.78 g/dL) of swine blood was used for centrifugation. The swine blood was separated in equal volumes into four 250 ml centrifuge tubes. Each was spun down at 3000 rpm for 20 min. Supernatants from all four tubes were discarded. The sedimented red blood cells (RBCs) were re-suspended with saline solution. The whole process was repeated for four centrifugation cycles.
The RBC concentrate was transferred into a 2 L Pyrex bottle, and was topped up to 1.5 L with pure water. A tangential flow ultrafiltration system of a Biomax V-screen (cutoff Mw.:1000 kDa; Millipore Corp.) was set up for the hemolysis and removal of stroma of swine RBCs. Hemolysis and removal of stroma were done simultaneously by adding pure water to the retentate RBC concentrate in the Pyrex bottle while maintaining a total volume of 1.5 L in the bottle. The ultrafiltrated SHb solution was collected.
The SHb solution as oxyhemoglobin (SHbO2) was converted to carbonylhemoglobin (SHbCO) by bubbling CO gas into the SHb solution for approximately 4 hours. SHbCO solution was heated overnight (15 hours) at 60 °C to inactivate viruses and to denature other proteins. After heating overnight, the solution was cooled to around 15 °C using ice/water. Then it was centrifuged at 3000 rpm for 30 minutes for two centrifugation cycles. The supernatant was collected into a new 2 L Pyrex bottle.
Dialysis and concentration of the SHbCO was performed using an ultrafiltration system (Biomax V-screen, Cut off Mw., 8 kDa; Millipore). After confirming that the sodium concentration (as NaCl) decreased below 0.01%, the SHbCO was concentrated to 42 g/dL.
Preparation of SHb-vesicles (SHbV)
With slight modifications, SHbVs were prepared using a method reported previously (Sakai et al. Citation2009). Pyridoxal 5’-phosphate (PLP; Sigma Chemical Co., St Louis, MO) was added to the SHbCO solution as an allosteric effector at a molar ratio of PLP/SHb tetramer = 0, 0.5, 1.0, and 2.5. After filtration of the SHbCO solution through a sterilizable filter (pore size 0.45 µm; Advantec Co.), it was mixed with lipids and encapsulated in vesicles. The lipid bilayer comprised 1,2-dipalmitoyl-sn-glycero-3-phosphatidylcholine (DPPC), cholesterol, 1,5-O-dihexadecyl-N-succinyl-L-glutamate (DHSG; Nippon Fine Chemical Co. Ltd., Osaka, Japan), and 1,2-distearoyl-sn-glycerol-3-phosphatidylethanolamine- N-PEG5000 (DSPE-PEG; NOF Corp., Tokyo, Japan) at the molar composition of 5/4/0.9/0.03. The particle diameter was regulated using a rapid mixing method. The encapsulated SHbCO was converted to SHbO2 by exposing the liquid membranes of HbVs to visible light under an aerobic atmosphere. The SHb concentration was measured using a modified cyanomethemoglobin (metHb-CN) method (Alfresa Pharma Corp., Tokyo). The phospholipid (DPPC) concentration was measured using a choline oxidase-DAOS method (Wako Pure Chemical Industries Ltd., Osaka, Japan). Finally, the Hb concentration of the suspension was adjusted to 10 g/dL. The particle diameter distribution was measured using a dynamic light-scattering method (Nanoparticle analyzer SZ-100; Horiba Ltd., Tokyo).
Analysis of thermal stability of SHb
Thermograms (30–120 °C) of SHb solutions in oxy, deoxy, and carbonyl states were obtained using a differential scanning calorimeter (DSC8500; Perkin Elmer Inc., MA, USA). The solutions (10 g/dl, 60 µl) were sealed with stainless steel capsules (Large volume capsules, LVC; Perkin Elmer Inc.). The LVCs eliminate the interfering effects of heat of vaporization by suppressing the vaporization of water even at 120 °C. The scanning rate was 1.0 °C/min.
Measurements of oxygen equilibrium curves of SHb and SHbV
The SHbCO solution was adjusted to about 2.5 g/dL in saline. It was converted to SHbO2 by photoreaction as described above. A Hemox analyzer (TCS Scientific Corp., PA, USA) was used to obtain the oxygen equilibrium curves. Either phosphate-buffered saline (PBS, pH 7.4; Gibco) or 30 mM phosphate buffer (PB, pH 7.4, without Cl‐) was used to dilute the solutions. SHbVs with different PLP contents were diluted with a Hemox solution. Oxygen equilibrium curves were obtained in the same manner.
Measurements of autoxidation rates of SHb and SHbV
A SHbO2 solution or a SHbV containing SHbO2 was suspended in PBS (pH 7.4) and incubated at 37 °C with gentle shaking. The metHb level of SHb solution was measured periodically using the method of Evelyn–Malloy using CN‐ (Matsubara Citation1987). For SHbV suspension, 3 ml of PBS was added to the Thunberg cuvette together with 10 µL of sample. The solution was subjected to bubbling of N2 gas for 4 minutes, resulting in the presence of deoxHb and metHb. Absorbance reading was then measured at 405 nm (metHb) and 430 nm (deoxyHb) using a spectrophotometer (V-650; Jasco Corp., Tokyo) and recorded. Calculations of two absorptions were then done to ascertain the level of metHb (Hamada et al. Citation1995).
For all the analyses described above, HHb was used for comparison.
Results
Purification of SHb and preparation of SHbV
Within 2 weeks after the withdrawal of swine blood, we were able to start the SHb purification. The yield of Hb from the first rinsing procedure with saline was 98% because of the lesser amount of hemolysis in the fresh blood. Tangential flow ultrafiltration (cut-off Mw. 1000 KDa) effectively removed stroma components in the turbid retentate fraction, and stroma-free SHb was collected in the permeate fraction. The stroma-free SHb was carbonylated and heated at 60 °C for 15 hours. During heating, denatured protein aggregated and floated in the solution. Centrifugation of the solution generated a precipitate of denatured proteins and a clear SHbCO solution with the yield of 90%. Tangential flow ultrafiltration (cut-off Mw. 8 KDa) effectively concentrated the Hb solution to 42 g/dL and removed electrolytes.
After the addition of PLP to the concentrated SHb solution at different molar PLP/SHb ratio (0, 0.5, 1.0, and 2.5), it was encapsulated in phospholipid vesicles in the same manner as that described for HHb. The particle diameter was regulated well at around 250 nm, on average. The weight ratio of SHb to total lipid was 1.0–1.1.
Thermal stability of SHb
DSC thermograms of the SHb solutions showed the denaturation temperatures in the oxy, deoxy, and carbonyl states to be, 71, 81, and 83 °C, respectively (). The deoxy SHb sample showed a smaller endothermal peak at around 70 °C, probably because of the presence of a small amount of SHbO2. The denaturation temperatures of SHbs resemble those of HHbs and BHbs ().
Figure 1. Calorimetric thermograms of SHbs in oxy, deoxy, and carbonyl forms. [Hb] = 10 g/dl, 60 µl, scanning rate = 1.0 °C/min.
![Figure 1. Calorimetric thermograms of SHbs in oxy, deoxy, and carbonyl forms. [Hb] = 10 g/dl, 60 µl, scanning rate = 1.0 °C/min.](/cms/asset/3839ad04-5f69-4b36-8ca9-fea6a3ac1b64/ianb_a_716067_f0001_b.gif)
Table I. Thermal stability of swine Hb (SHb) compared with those of human Hb (HHb) and bovine Hb (BHb).
Oxygen affinities of SHb and SHbV
shows that P50 values for all samples increase as the molar ratio of PLP/SHb increases. It was suspected that Cl‐ ions in PBS might affect oxygen affinity. Therefore, phosphate buffer (PB) without Cl‐ was used as well to plot the oxygen equilibrium curves of HHb and SHb in the various PLP/SHb ratios using the Hemox analyzer. However, there was only a small difference in P50 values. Therefore, Cl‐ ions do not affect the oxygen affinity of SHb solutions. Little difference was apparent in the oxygen affinity of SHb and HHb, for solutions in both PBS and PB. Without co-encapsulation of PLP, both SHbV and HHbV showed similar P50 at around 10 mmHg. With increasing amount of PLP, SHbV has much higher P50 values (lower oxygen affinity) than the HHbV solution. Hill numbers for the SHb solutions tended to decrease as the PLP/SHb ratio was increased from around 2.5 to around 2.0. The trend for Hill number values in SHbV solutions differed: the Hill number increased instead as PLP/SHb ratio increases from 1.4 to 1.9.
Figure 2. Oxygen affinities (P50 values) of SHb and HHb samples containing different amounts of pyridoxal 5’-phosphate (PLP), obtained from oxygen equilibrium curves measured using a Hemox analyzer at 37 °C. Phosphate buffered saline (PBS, pH 7.4) and phosphate buffer (PB, pH 7.4) were used for dissolving Hb to confirm the effect of Cl‐ anion. HbV is dispersed in hemox buffer.
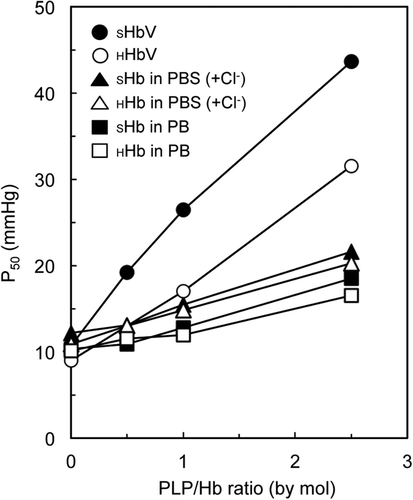
Autoxidation rates of SHb and SHbV
presents the time-dependent increases of metHb levels of SHbO2 dissolved in PBS at 37 °C in an aerobic condition in comparison to HHbO2. In the absence of any metHb reduction enzymatic system, the metHb level invariably increases. No significant difference was found between the two samples.
Figure 3. Autoxidation of SHbO2 in pH 7.4 PBS compared with that of HHbO2 in aerobic conditions at 37 °C.
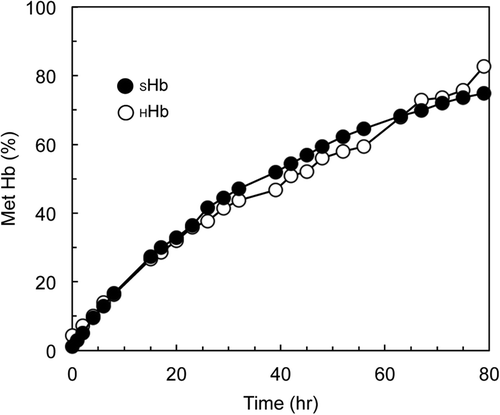
shows time-dependent increases of metHb levels of SHbV dispersed in PBS at 37 °C in an aerobic condition in comparison to HHbV. SHbV showed slightly faster autoxidation rates at all PLP/SHb ratios.
Discussion
This study is our first preparation of Hb-vesicles by using SHb. The purification of SHb from swine whole blood was performed effectively as we always do for human Hb (HHb), through stabilization by carbon monoxide (CO), pasteurization at 60 °C for 15 hours, and tangential flow ultrafiltration. Hb encapsulation was conducted in the same manner as that for HHb. Physicochemical characteristics of SHbV and HHbV are similar. But some differences are apparent in oxygen affinity and autoxidation rate between the two.
Both carbonyl and deoxy SHb showed higher denaturation temperatures than in the oxygen bound state. This tendency is the same with HHb and BHb. Even though oxy SHb shows denaturation temperature above 60 °C, it autoxidizes to form metHb even at a lower temperature as shown in . Actually, metHb is known to induce irreversible transformation of globin helix chains gradually. Both carbonylation and deoxygenation can prevent metHb formation effectively. This thermal stability guarantees that SHbCO can be heated at 60 °C for pasteurization without denaturation.
The oxygen affinities of SHb in PBS (with Cl‐) and PB (without Cl‐) are similar. This tendency is similar to that of HHb, but not BHb. Reportedly BHb is affected strongly by the addition of Cl‐ (Fronticelli et al. Citation1984, Perutz et al. Citation1993). Mammalian Hbs are classified into two groups: those with intrinsically high O2 affinity, and those with intrinsically low O2 affinity (Perutz and Imai Citation1980). In fact, both HHb and SHb are examples of the former and BHb of the latter group. Rodents, dogs, pigs, horses, camels, marsupials, and most primates belong to the former category. Cows, sheep, goats, deer, cats, and one primate, the lemur, belong to the latter. The high O2 affinity of the former group is lowered in RBCs by 2,3-diphosphoglyceric acid (2,3-DPG). Therefore, SHb should be mixed with pyridoxal 5’-phosphate (PLP), a substitute of 2,3-DPG.
Addition of PLP to SHb before encapsulation decreases its oxygen affinity (increase P50 value) in the same manner as it does with HHb. However, after encapsulation in vesicles, SHb showed much lower oxygen affinity (higher P50) than HHb, which indicates that SHb interacts with PLP more strongly than HHb in a concentrated condition in the liposomal particle.
According to reports of Sinet et al. (Citation1982) and Condo et al. (Citation1992), SHb has lower O2 affinity (higher P50) than HHb has at a lower temperature, and the P50 of SHb is less affected by temperature than HHb is. These characteristics of SHb are consistent with the well-known cold resistance of pigs. That presents one potential advantage of utilization of SHb for a blood substitute over HHb in terms of larger capacity of oxygen transport in hypothermic conditions.
Autoxidation rates of purified SHbO2 and HHbO2 are similar. However, after encapsulation in vesicles, SHbO2 showed higher rates than HHbO2. The reason remains unclear. In spite of the overall structural similarity of SHb and HHb, small structural differences include (i) a large segmental shift of helix A of the β-subunit, (ii) slight uncoiling of the amino-terminal residues of helix B of the β-subunit and the carboxyl-terminal residue of helix E of the β-subunit, and (iii) small difference in loop regions connecting α-helices, and the eight subunit termini (Katz et al. Citation1994). Such a difference might affect the faster autoxidation rate, especially in a highly concentrated condition within vesicles. Autoxidation would be retarded by non-enzymatic and enzymatic methods which we reported elsewhere (Tsuchida et al. Citation2009).
Conclusion
Swine Hb can be purified effectively from whole blood through carbonylation, pasteurization, and tangential flow ultrafiltration. The concentrated swine Hb was encapsulated in phospholipid vesicles. Accordingly, our routine procedure to prepare HbV using human Hb is applicable to swine Hb. Despite these results, some differences exist in terms of oxygen affinity and autoxidation rate, but sHb can be regarded as an alternative source for the production of Hb-vesicles as an artificial oxygen carrier.
Declaration of interest
Of the authors, H.S. is a holder of patents related to the production and usage of Hb-vesicles. The authors alone are responsible for the content and writing of the article.
This study was supported in part by Health and Labour Sciences Research Grants (Health Science Research Including Drug Innovation) from the Ministry of Health, Labour and Welfare, Japan, and a Supporting Project to Form Strategic Research Platform for Private Universities: Matching Fund Subsidy from Ministry of Education, Culture, Sports, Science and Technology, Japan.
References
- Barnikol WKR, Burkhard O, Poetzschke H, Domack U, Dinkelmann S, Guth S, . 2002. New artificial oxygen carriers made of pegylated polymerized pyridoxalated porcine haemoglobin. Comp Biochem Physiol Part A. 132:185–191.
- Chang TMS. 2006. Evolution of artificial cells using nanobiotechnology of hemoglobin based RBC blood substitute as an example. Artif Cells Blood Substit Immobil Biotechnol. 34:551–566.
- Chang W, Chang Y, Chen Y, Sung H. 2004. Hemoglobin polymerized with a naturally occurring crosslinking agent as a blood substitute: In vivo and in vitro studies. Artif Cells Blood Substitutes Biotechnol. 32:243–262.
- Condo SG, Corda M, Sanna MT, Pellegrini MG, Ruiz MP, Castagnola M, . 1992. Molecular basis of low-temperature sensitivity in pig hemoglobin. Eur J Biochem. 209:773–776.
- Fronticelli C, Bucci E, Orth C. 1984. Solvent regulation of oxygen affinity in hemoglobin, Sensitivity of bovine hemoglobin to chloride ions. J Biol Chem. 259:10841–10844.
- Hamada K, Kose T, Ohgushi T, Sakai H, Takeoka S, Nishide H, . 1995. Spectroscopic assay system for the determination of red cell substitutes (NRC). Artif Blood. 3:96–101 (in Japanese).
- Katz DS, White SP, Huang W, Kumar R, Christanson DW. 1994. Structure determination of aquomet porcine hemoglobin at 2.8 A resolution. J Mol Biol. 244:541–553.
- Lee CJ, Kan P, Chen WK. 1992. The purification and comparative analysis of hemoglobin from animal bloods. Biomater Artif Cells Immobilization Biotechnol. 20:477–488.
- Matsubara T. 1987. Spectrophotometric determination of hemoglobin in blood. Tanpakushisu-kakusan-koso (Protein, Nucleic Acid and Enzyme). 32:671–674 (in Japanese).
- Natanson C, Kern SJ, Lurie P, Banks SM, Wolfe SM. 2008. Cell-free hemoglobin-based blood substitutes and risk of myocardial infarction and death: a meta-analysis. JAMA. 299:2304–2312.
- Olson JS, Foley EW, Rogge C, Tsai AL, Doyle MP, Lemon DD, . 2004. No scavenging and the hypertensive effect of hemoglobin-based blood substitutes. Free Radic Biol Med. 36:685–697.
- Perutz MF, Imai K. 1980. Regulation of oxygen affinity of mammalian haemoglobins. J Mol Biol. 136:183–191.
- Perutz MF, Fermi G, Poyart C, Pagnier J, Kister J. 1993. A novel allosteric mechanism in haemoglobin: structure of bovine deoxyhaemoglobin, absence of specific chloride-binding sites and origin of the chloride-linked Bohr effectin bovine and human haemoglobin. J Mol Biol. 233:536–545.
- Sakai H, Hara H, Yuasa M, Tsai AG, Takeoka S, Tsuchida E, Intaglietta M. 2000. Molecular dimensions of Hb-based O2 carriers determine constriction of resistance arteries and hypertension. Am J Physiol Heart Circ Physiol. 279:H908–H915.
- Sakai H, Masada Y, Takeoka S, Tsuchida E. 2002. Characteristics of bovine hemoglobin as a potential source of hemoglobin-vesicles for an artificial oxygen carrier. J Biochem. 131:611–617.
- Sakai H, Sou K, Tsuchida E. 2009. Hemoglobin-vesicles as an artificial oxygen carrier. Methods Enzymol. 465:363–384.
- Sakai H, Sato A, Masuda K, Takeoka S, Tsuchida E. 2008. Encapsulation of concentrated hemoglobin solution in phospholipid vesicles retards the reaction with NO, but not CO, by intracellular diffusion barrier. J Biol Chem. 283:1508–1517.
- Sinet M, Bohn B, Guesnon P, Poyart C. 1982. Temperature independence of the alkaline Bohr effect in pig red cells and pig hemoglobin solutions. Biochim Biophys Acta. 708:105–111.
- Tsuchida E, Sou K, Nakagawa A, Sakai H, Komatsu T, Kobayashi K. 2009. Artificial oxygen carriers, hemoglobin vesicles and albumin-hemes, based on bioconjugate chemistry. Bioconjug Chem. 20:1419–1440.
- Yu B, Shahid M, Egorina EM, Sovershaev MA, Raher MJ, Lei C, . 2010. Endothelial dysfunction enhances vasoconstriction due to scavenging of nitric oxide by a hemoglobin-based oxygen carrier. Anesthesiology. 112:586–594.
- Zhang W, Yan K, Dai P, Tian J, Zhu H, Chen C. 2011. A novel hemoglobin-based oxygen carrier, polymerized porcine hemoglobin, inhibits H2O2-induced cytotoxicity of endothelial cells. Artif Organs. 36:151–160.