Abstract
In this study, the production of ethyl butyrate was investigated by using immobilized lipase enzyme in shake flasks. In order to determine optimum conditions for the production, response surface methodology was used. The model indicated the optimum conditions for maximum conversion (9.1%) at the 0.31 M substrate concentration, acid- alcohol molar ratio of 0.49, immobilized enzyme 25% (w/v) at 35°C, for 3 hours which were in good agreement with the experimental value. At the end of the 55 hours conversion was obtained as 61.3%. When Na2HPO4 was used in reaction medium conversion increased to 90.3% for 55 hours.
Introduction
Short-chain esters are very important in food, beverage, cosmetic, and pharmaceutical industries as flavor and fragrance constituents (Jeager and Reetz Citation1998). Ethyl butyrate is in high demand as a component of pineapple flavors in food industries and is produced by using ethyl alcohol and butyric acid.
Esters are generally produced by chemical reaction of an alcohol with an organic acid in the presence of acid catalyst (Kirk and Othmer Citation1991) or by extraction from natural sources (Welsh et al. Citation1989). Enzymatic reactions for the production of esters were preferred because of several advances (Güvenc et al. Citation2002, Zaidi et al. Citation1995).
Lipases (triacylglycerol lipases E.C. 3.1.1.3) display catalytic activity towards a large variety of alcohols and acids in ester synthesis (Armstrong and Yamazaki Citation1986, Gilles et al. Citation1987, Langrand et al. Citation1988, Dias et al. Citation1991, Manjon et al. Citation1991, Thakar and Madamwar Citation2005, Hamsaveni et al. Citation2001, Krishna et al. Citation2001, Won et al. Citation2005, Chabouni et al. Citation2006, Pires-Cabral et al. Citation2010, Ribeiro et al. Citation2010). Investigators have used immobilized lipases in all of these reactions. Because immobilized lipase have high catalytic activity and operational stability in order to lower the costs of the biocatalyst in the process by reusing it in batch cycles or using it in continuous reactors (Lee Citation1996).
Esters’ synthesis must be carried out in low water environment (e.g. nonpolar organic solvents) water causes the displacement of the reaction equilibrium towards hydrolysis. In this study gelatin immobilization was preferred because of its hydrophilic gel property. A low water content of the gelatin gel support is necessary to maintain the native tertiary structure of lipase (Tanaka and Sonamato Citation1990, Petkar et al. Citation2006).
Gelatin is an inexpensive abundant and safe material largely used as an immobilization matrix (Chen Citation1996). Even though lipase has been immobilized in gelatin membranes (Fadnavis and Koteshwar Citation1999) the lipase immobilized in gelatin pieces have not been fully examined (Hedström et al. Citation1998, Dave and Madamwar Citation2008, Nagayama et al. Citation2008, Pulat and Akalın Citation2013).
Response surface methodology (RSM) has been a popular and effective method to solve multivariate problems and optimize several responses in many types of experimentation (Pires-Cabral et al. Citation2007, Bayraktar Citation2001, Murthy et al. Citation2000). The aim of the present study is to optimize the production of ethyl butyrate by using RSM. The effect of Na2HPO4 on the ethyl butyrate yield was also investigated.
Materials and methods
Materials
The lipase enzyme (Novozym 735 Candida antarctica lipase-A) obtained from Novozymes (Denmark) was used for immobilization. Butyric acid, ethanol, ethyl butyrate, n-hexane, gelatin, and glutaraldehyde were analytical grade and obtained from various chemical sources.
Preparation of immobilized lipase
A modification of the procedure given by Hedström et al. (Citation1998) was used. 700 mg gelatin was dissolved in 3.5 mL of distilled water at 50°C. 1.0 mL of the enzyme was added and stirred gently for 5 min. After 1 hour at 25°C, 2.5 mL of 10% glutaraldehyde solution was added. Following this process the mixture was kept in refrigerator at 4°C after 24 hours the gelatin was cut in to small pieces (3.0 mm3, 4.0 g of immobilized lipase preparation which contains 1.0 mL free lipase). The immobilized lipase was finally washed with distilled water and maintained in 0.5 M phosphate buffer pH 7.0 at 4°C. The filtered phosphate buffer solution and washings were collected for loading efficiency determination (Won et al. Citation2005). Protein concentrations were measured with Bradford Reagent using standard method (Bradford Citation1976).
Loading efficiency (%) = ((CiVi× Cf Vf)/CiVi)× 100(1)
Where Ci is the initial protein concentration, Vi the initial volume of enzyme solution, Cf the protein concentration in the total filtrate, and Vf the total volume of filtrate.
Esterification reaction
The reaction was carried out in 50 mL erlenmeyer flask at 35°C in a shaker at 150 rpm. An appropriate amount of immobilized enzyme was added to the reaction media containing different concentration of substrates (butyric acid ethyl alcohol) in 20 mL n-hexane. To determine specific activity and loading activity ester production was performed at 20 mL working volume in the shake flask at 0.31 M butyric acid 0.63 M ethyl alcohol and the enzyme preparation (5.0 g of immobilized enzyme or 1.25 mL of free enzyme). The esterification activity was defined as μmoles of ester formed per minute under reaction conditions.
Sample analysis
At regular intervals of time sample were taken from the hexane phase. The concentration of ester produced was determined by using a gas chromatograph (Carlo Erba Instument GC 8000 Top) equipped with a ZB-FFAP capillary column (50 m length 0.32 mm i.d. 50 μm film thickness) and a flame ionization detector. Nitrogen was used as a carrier gas. Injection port and detector temperatures were maintained at 200°C and 260°C, respectively. Initial column temperature was 50°C for 5 min. Temperature was increased from 50 to 100°C by 25°C/min and maintained at 100°C for 2 min. Then temperature was increased from 100 to 175°C by 25°C/min and maintained at 175°C for 2 min. Internal standard (1-hexanol) was added to each sample (internal standard/sample ratio: 1/10).
Experimental design and statistical analysis
The effects of three variables (enzyme concentration, substrate concentration, and mole ratio alcohol/acid) on the production of ethyl butyrate were studied using RSM. The aim of the RSM is to find out the optimum operating conditions for a given system or the way in which a particular response is affected by the set of variables over some specific region of interest. RSM includes a group of empirical techniques to find the relationship between controlled, experimental, and independent factors and measured responses (Khuri and Cornell Citation1996, Kılıc et al. Citation2002, Sanal et al. Citation2005). 23 full factorial central composite designs for three independent variables were used in this study. The full factorial composite design consists of a complete 2k factorial design where k is the number of test variables n0 centre points (n0 ≥ 1) and two axial points on the axis of each design variable at a distance of α (= 2k/4, α = 1.68 for k = 3) from the design center. The total number of design points is N = 2k + 2k + n0 (20 experiments for 3 independent variables).
The range and the levels of the variables investigated in this study are given in . In the regression equation the test variables were coded according to the following equation (Bayraktar Citation2001, Murthy et al. Citation2000).
Table I. Coded value of the independent variables and experimental ranges.
Where xi is the coded value of the i-th independent variable Xi is the uncoded value of the i-th independent variable Xi* is the uncoded value of the i-th independent variable at the centre point and ∆Xi is the step change value. More specifically coded values of the independent variables were calculated as follows:
The “Design Expert” software (Version 6.06 Stat-Ease Inc. Minneapolis USA) was used for regression and graphical of the data obtained.
Results and discussion
Specific activity and loading efficiency
The esterification activities and free and immobilized enzymes were determined as 3.51 and 2.44 μmol.min−1, respectively. Specific activities were calculated and for free and immobilized enzyme it were 9.75 10−2 and 7.01 10−2 μmol min−1 mg protein−1, respectively. Immobilization yield was defined as the ratio of specific activity of immobilized enzyme to specific activity of free enzyme. Loading efficiency (percent of total enzyme immobilized) was calculated in Eq. (1). Loading efficiency was found as 96.7%. Immobilization yield was 71.9%. This is due to conformational changes in the immobilized enzyme or limitation of substrate transfer in to gelatin.
Optimization of the esterification reaction
RSM experiments were performed at 20 mL working volume 35°C temperature for 3 hours. On the basis of the literature data the effect of enzyme concentration (X1 w/V %), butyric acid (substrate) concentration (X2 M), and molar ratio butyric acid/ethanol (X3 mol/mol) on the conversion into ethyl butyrate was studied using RSM. A second order polynomial model was fitted to the conversion into ester. The experiments were performed and the results obtained under the operational conditions employed were listed in . The application of RSM yielded the followed regression equation which is an empirical relationship between the conversion into ester and the test variables in coded units.
Table II. Experimental matrix and values of observed response.
The analysis of variance was given in . The Fisher F test with a very low probability value (P model > F < 0.0002) demonstrates a very high significance for the regression model and confirms the adequacy of the quadratic model. In addition the model F-value of 12.65 also implies the model is significant. The coefficient of determination (R2) of the model was 0.9193 which indicated that the model adequately represented the real relationship among the selected reaction parameters. In practice R2 should be at least 0.75 or grater; values above 0.90 are considered to be very good (Pires-Cabral et al. Citation2007). Adjusted R2 of this model was 0.8466. Adjusted R2 shows an estimate of the fraction of overall in the data accounted for by the model. It is always smaller than R2.
Table III. Analysis of variance (anova) for the quadratic model.
The significance of each coefficient was determined by F-value and Prob> F-value (). Values of “Prob > F” less than 0.0500 indicate model terms are significant. These significant values are shown as bold in . In this study the coefficients of x1 (Prob> F = 0.0003), x2 (Prob> F = 0.0030), x3 (Prob> F = 0.0426), x12 (Prob> F = 0.0105), x22 (Prob> F = 0.0114), and x32 (Prob> F < 0.0001) are significant model terms.
The response surfaces obtained according to the RSM analysis for each conversion value are shown in –3. Response surface plots provide a method to predict the conversion for different values of the test variables and the contour plots help us to identify the type of interactions between test variables. shows the response surface plot to enzyme concentration versus substrate (butyric acid) concentration. The conversion increased with increasing enzyme concentration. At the same time the conversion improved by increasing butyric acid concentration from 0.13 M to 0.30 M at about 25% (w/V) of enzyme concentration. Above 0.30 M of butyric acid concentration it decreased due to inhibition of acid at a certain enzyme concentration. The best result was obtained at high enzyme concentration (25% w/V) and mild substrate concentration (0.29 M).
Figure 1. Contour plot of conversion into ester: the effect of enzyme and substrate concentration and their mutual interaction on ethyl butyrate production. other variable is held at zero level.
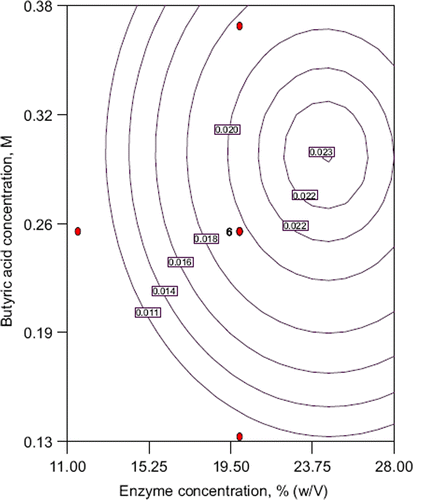
When the response surface is examined it can be said the contours on the are elliptical form. An elliptical or saddle nature of the contour plots indicates the significance of the interactions between the corresponding variables. Therefore the interaction between mole ratio acid/alcohol and enzyme concentration is more effective. Conversion into ester increases with increasing mole ratio to 0.50 after this value it decreases (). It was reported that alcohols have inhibition effects on lipase and the increment of the alcohol concentration (mole ratio decreases) lowered the esterification capacity of lipases (Rodriguez-Nogales et al. Citation2005, Chowdary et al. Citation2000) At the same time the conversion decreases with increasing alcohol concentration due to the accumulation of water during the progress of the reaction and water causes the backward reaction (hydrolysis).
Figure 2. Contour plot of conversion into ester: the effect of mole ratio, enzyme concentration and their mutual interaction on ethyl butyrate production. other variable is held at zero level.
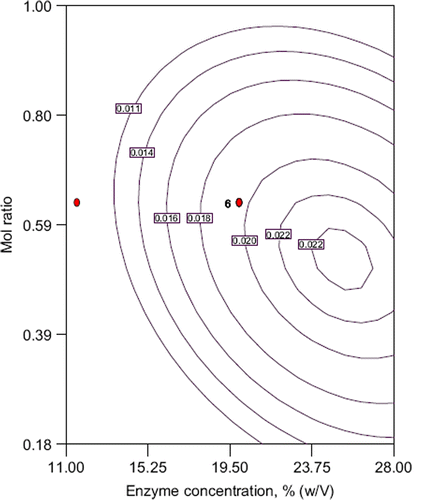
shows that the interaction between butyric acid concentration and mole ratio. The elliptical contour plot was also obtained. There is significant interaction with these parameters. Generally it is known that both acid and alcohol have inhibition effect for an esterification reaction. Also acid inhibition is more dominant than alcohol inhibition (Güvenc et al. Citation2002). The same situation was also observed in this study. Maximum conversion (2.1%) was obtained at about 0.50 acid/alcohol mole ratio for 3 hours ().
Figure 3. Contour plot of conversion into ester: the effect of mole ratio, substrate concentration and their mutual interaction on ethyl butyrate production. other variable is held at zero level.
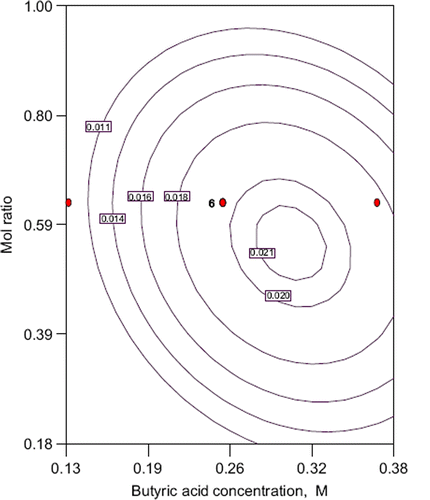
The optimum conditions were determined as a result of RSM application. The optimum values of the test variables for maximum conversion into ester (9.1%) and the ester concentration (0.028 M) for 3 hours were immobilized enzyme concentration 25% (w/V), substrate (butyric acid) concentration 0.31 M, and molar ratio acid/alcohol 0.49. In order to verify these results, esterification reaction was carried out at the optimum conditions and conversion into ester as 7.1% and ester concentration as 0.022 M at 3 hours. This result was in good agreement with the predicted value from RSM.
The time course of ethyl butyrate concentration produced at 35°C by using optimal conditions was shown in . Ethyl butyrate concentration did not change much after 55 hours. Max. ester concentration was obtained 0.19 M. Under these conditions the conversion into ester was 61.3%. When solid Na2HPO4 salt was added in the same reaction medium final concentration was 0.14 M ethyl butyrate and the conversion reached to 90.3%. The phosphate salt is the hygroscopic salt adsorbing water and hence giving higher ester yields (Romero et al. Citation2005).
Conclusions
Enzymatic synthesis of ethyl butyrate was carried out using butyric acid and ethyl alcohol with immobilized Candida antarctica lipase-A. RSM was used to optimize reaction conditions. Maximum ester concentration was found as 0.19 M (61.8% conversion) for 55 hours. When 0.14 M Na2HPO4 solid salt was added into the same reaction medium ester concentration was increased to 0.28 M (90.3% conversion). Carboxylic acids may be a potent inhibitor and cause the enzyme deactivation by acidification microaqueous environment of enzyme. The phosphate salt is the hygroscopic salt adsorbing water and hence giving higher ester yields (Romero et al. Citation2005).
Declaration of interest
The authors report no declarations of interest. The authors alone are responsible for the content and writing of the paper.
References
- Armstrong DW, Yamazaki H. 1986. Natural flavors production: a biotechnological approach. Trends Biotechnol. 4:264–268.
- Bayraktar E. 2001. Response surface optimization of the separation of DL- typtophan using an emulsion liquid membrane. Process Biochem 37:169–175.
- Bradford MM. 1976. A rapid and sensitive method for the quantitation of microgram quantities of protein utilizing the principle of protein-dye binding. Anal Biochem. 72:248–254.
- Chabouni MK, Ghamgui H, Bezine S, Rekik A, Gargouri Y. 2006. Production of flavor esters by immobilized Staphylococus simulanslipase in a solvent-free system. Process Biochem. 41:1692–1698.
- Chen JP. 1996. Production of ethyl butyrate using gel entrapped Candida cylindracealipase. J Ferment Bioeng. 82:404–407.
- Chowdary GV, Ramesh MN, Prapulla SG. 2000. Enzymatic synthesis of isoamyl isovalerate using immobilized lipase from Rhizomucor miehei: a multivariate analysis. Process Biochem. 36:331–339.
- Dave R, Madamwar D. 2008. Candida rugosalipase immobilization in Triton-X100 microemulsion based organels (MBGs) for ester synthesis. Process Biochem. 43:70–75.
- Dias SF, Boas LV, Cabral JMS, Fonseca MMR. 1991. Production of ethyl butyrate by Candida rugosa lipase immobilization in polyurethane Biocatal. 5:21–34.
- Fadnavis NW, Koteshwar K. 1999. An unusual reversible sol-gel transition in organic application for enzyme immobilization in gelatin membranes. Biotechnol Progr. 15:98–104.
- Gilles B, Yamazaki H, Armstrong DW. 1987. Production of flavor esters by immobilized lipase. Biotechnol Lett. 9:709–714.
- Güvenc A, Kapucu N, Mehmetoğlu U. 2002. The production of isoamyl acetate using immobilized lipases in a solvent- free system. Process Biochem. 38:379–386.
- Hamsaveni DR, Prapulla SG, Divakar S. 2001. Response surface methodological approach for the synthesis of isobutyl isobutyrate. Process Biochem. 36:1103–1109.
- Hedström G,Backlund S,Eriksson F,Karlsson S. 1998. Lipase- catalysed stereoselective esterifications using gelatin-based hydrogels. Colloid Sur B 10:379–384.
- Jeager KE, Reetz MT. 1998. Microbial lipases from versatile tools for biotechnology. Tıbtech. 16:396–403.
- Khuri AI, Cornell JA. 1996. Response Surfaces: Design and Analyses. New York: Marcel Dekker.
- Kılıc M, Bayraktar E, Ates S, Mehmetoğlu U. 2002. Investigation of extractive citric acid fermentation using response surface methodology. Process Biochem. 37:759–767.
- Kirk RE, Othmer DF. 1991. Encyclopedia of Chemical Technology, vol. 9. New York: Wiley, pp. 781–812.
- Krishna SH, Sattur AP, Karanth NG. 2001. Lipase-catalysed synthesis of isoamyl isobutyrateProcess Biochem. 37:9–16
- Langrand G, Triantaphylides C, Baratti J. 1988. Lipase catalyzed formation of flavor estersBiotechnol Lett. 10:549–554.
- Lee BH. 1996. Fundamentals of Food Biotechnology. New York: VCH Publisher.
- Manjon A, Iborra JL, Arocas A. 1991. Short-chain flavor ester synthesis by immobilized lipase in organic media. Biotechnol Lett. 13: 339–344.
- Murthy MSRC, Swaminathan SK, Rakshit SK, Kosugi Y. 2000. Statistical optimization of lipase catalyzed hydrolysis of methyloleate by response surface methodology. Bioproc Eng. 22:35–39.
- Nagayama K, Katakura R, Hata T, Imai M. 2008. Reactivity of Candida rugosalipase in CTAB microemulsion-gelatin complex organogels. Biochem Eng J. 38:274–276.
- Petkar M, Lali A, Caimi P, Daminati M. 2006. Immobilization of lipases for non-aqueous synthesis. J Mol Catal B Enzym. 39:83–90.
- Pires-Cabral P, Fonseca MMR, Ferreira-Dias S. 2007. Modelling the production of ethyl butyrate catalysed by Candida rugosalipase immobilized in polyurethane foams. Biochem Eng J. 33: 148–158.
- Pires-Cabral P, Fonseca MMR, Ferreira-Dias S. 2010. Esterification activity and operational stability of Candida rugosalipase immobilized in polyurethane foams in the production of ethyl butyrate. Biochem Eng J. 48:246–252.
- Pulat M, Akalın GO. 2013. Preparation and characterization of gelatin hydrogel support for immobilization of Candida rugosa lipase. Artificial Cells, Nanomedicine, and Biotechnology, 41:145–151.
- Ribeiro AS, Oliveira MV, Rebocho SF, Ferreira O, Vidinha P, Barreiros S,2010. Enzymatic production of decyl acetate. Ind Eng Chem Res. 49:7168–7175.
- Rodriguez-Nogales JA, Roura E, Contreras E. 2005. Biosynthesis of ethyl butyrate using immobilized lipase: a statistical approach. Process Biochem. 40:63–68.
- Romero MD, Calvo L, Alba C, Daneshfar A, Ghaziaskar HS. 2005. Enzymatic synthesis of isoamyl acetate with immobilized Cantarcticalipase in n-hexane. Enzyme Microb Technol. 37:42–48.
- Sanal IS, Bayraktar E, Calımlı A, Mehmetoğlu U. 2005. Determination of optimum conditions for SC-(CO2 + Ethanol) extraction of β- carotene from apricot pomace using response surface methodology. J Supercrit Fluid. 34:331–338.
- Tanaka A, Sonamato K. 1990. Immobilized biocatalyst in organic solvents. Chem Technol. 20:112–117.
- Thakar A, Madamwar D. 2005. Enhanced ethyl butyrate production by surfactant coated lipase immobilized on silica. Process Biochem. 40:3263–3266.
- Welsh FW, Muray WD Williams RE. 1989. Microbiological and enzymatic production of flavor and fragrance chemicals. Crit Rev Biotechnol. 9:105.
- Won K, Kim S, Kim K, Park HW, Moon S. 2005. Optimization of lipase entrapment in Ca-alginate beads. Process Biochem. 40: 2149–2154.
- Zaidi A, Gainer JL, Carta G. 1995. Fatty acid esterification using nylon- immobilized lipase. Biotechnol Bioeng. 48:601–605.