Abstract
Catalase was immobilized on chitosan and modified chitosan. Studies were carried out on free-immobilized catalase concerning the determination of optimum temperature, pH, thermal, storage stability, reusability, and kinetic parameters. Optimum temperature and pH for free catalase and catalase immobilized were found as 35°C and 7.0, respectively. After 100 times of repeated tests, the immobilized catalases on chitosan-clay and magnetic chitosan maintain over 50% and 60% of the original activity, respectively. The ease of catalase immobilization on low-cost matrices and good stability upon immobilization in the present study make it a suitable product for further use in the food industry.
Introduction
The application of enzymes in their native form in biochemical, biomedical, biotechnological, and food industrial fields is not always suitable and optimal. Binding of enzymes on a solid support is an advantageous modification for their application. Enzymes are immobilized onto or into a solid matrix to increase their thermostability, operational stability, and recovery (Çetinus and Öztop Citation2003). In addition to these, enzyme inactivation can be reduced and its economic value is increased by immobilization (Chang and Juang Citation2005).
Catalase (EC 1.11.1.6) is a heme containing metalloenzyme and regarded as one of the most common enzymes naturally found in almost all living organisms. Catalase consists of four subunits, each of them involving ferriporphyrin as a prosthetic group. Catalase catalyzes the decomposition of hydrogen peroxide to water and oxygen. Catalase has potential industrial applications in free and immobilized forms. In food industry, catalase removes hydrogen peroxide from milk thus aiding in cheese production. Catalase prevents food from oxidizing therefore can be used in food wrapping. Also it is a strong oxidizing and bleaching agent (Çetinus and Öztop Citation2003).
Chitosan is well known as an ideal support for enzyme immobilization because of its features such as hydrophilicity, biocompatibility, biodegradability, and antibacterial properties (Juang et al. Citation2001). Chitosan was utilized for immobilization by covalent coupling of the enzyme catalase to glutaraldehyde-activated chitosan (Albarghouthi et al. Citation2000). Glutaraldehyde activation of supports is one of the most popular techniques to immobilize enzymes. The methodology is quite simple and efficient. Furthermore, in some instances, it allows to improve enzyme stability by multipoint immobilization. Multipoint covalent attachment promotes a rigidification of the molecular structure of the immobilized enzyme, which can reduce the conformational change induced by any distorting agent (heat, organic solvents, and extreme pH values), thus greatly increases the enzyme stability (Pan et al. Citation2009)
In recent decades, physical or chemical modification of chitosan has been frequently conducted to broaden its applications. The physical modification could increase the adsorption properties of chitosan; the chemical modification, on the other hand, can not only increase its adsorption properties and prevent its dissolution in strong acids, but also improve the mechanical strength and superficial area (Pan et al. Citation2009, Xiao and Zhou Citation2008). In recent years, many studies have focused on seeking cheap, locally available and effective carriers, for example waste biopolymers, clays and clay minerals, etc. Among these materials, clay and chitosan are relatively cheap and exhibit higher carrier capacities. Chitosan beads can be improved in conjunction with other powders such as clays and activated carbons to increase its density and mechanical strengthen to extend its application (Chang and Juang Citation2005).
In this paper, we aim to synthesize a chitosan resin modified by magnetic-Fe3O4 nanoparticles and clay. When chitosan is mixed with some solid powders such as clays and activated carbons, it can increase chitosan's density and mechanical strength, thereby extending application possibilities (Wu et al. Citation2002). On the other hand, magnetic Fe3O4 nanoparticles have large specific surface and magnetite-chitosan beads can be easily separated from reaction mixture by magnetic field (Pan et al. Citation2009). We prepared modify chitosan beads with clay and magnetite nanoparticles. Temperature, pH, storage, and operational stability of immobilized enzymes were studied and activities of catalase immobilized on chitosan, chitosan-clay, and chitosan-Fe3O4 beads were compared. This is of practical importance for further applications.
Materials and methods
Materials
Chitosan, catalase (hydrogen peroxide oxidoreductase; EC.1.11.1.6, bovine liver), glutaraldehyde, sodium triphosphate pentabasic (TPP), nanoclay (hydrophilic bentonite, average particle size: ≤ 25 μm, H2Al2O6Si, 180.1 g/mol) were obtained from Sigma Chemical Co. (St. Louis, MO, USA). Hydrogen peroxide and all other chemicals were obtained from Merck AG (Darmstadt, Germany).
Preparation of chitosan beads
Fe3O4 nanoparticles were prepared by coprecipitating of Fe2+ (FeSO4•7H2O) and Fe3+ (FeCl3•6H2O) ions in ammonia solution (Yuanbi et al. Citation2008).
To prepare the chitosan-clay and magnetite-chitosan beads, 1 g chitosan flake was dissolved in acetic acid (2%, 100 ml), 1 g clay or 200 mg magnetic Fe3O4 was added into chitosan solution and then were agitated by magnetic string at room temperature. After 10 min, the viscous solution was placed in an ultrasonic bath to remove air bubbles. The chitosan-clay or magnetite-chitosan solution was dropped through a syringe into cold TPP solution (pH: 8.2) and was stirred for 4 h. Then, the beads were washed with deionized water until they became neutral and were stored in deionized water for use afterwards.
Preparation of crosslinking solution
Glutaraldehyde was utilized as a crosslinking solution. Adequate composite (0, 1 g) was placed in 3% (v/v) of glutaraldehyde (100 ml) and crosslinked at 150 rpm and 30°C (for chiosan-clay beads 4 h and for Fe3O4-chitosan beads 3 h). Then the beads were washed several times with deionized water to remove excess of glutaraldehyde.
Immobilization of catalase on chitosan and modified chitosan beads
An amount of cross-linked bead (0.05 g) was added to 2 ml of the catalase solution (1 mg/ml) and the immobilization reaction was accomplished in a rotary shaker for 4 h at 4°C. The beads were washed with 0.05 M phosphate buffer and were stored in phosphate buffer at 4°C until use.
Activity assays of catalase
The activity of catalase was determined spectrophotometrically at 240 nm by measurement of decrease in the absorbance of hydrogen peroxide owing to its decomposition by the enzyme (Aebi Citation1984). Catalase-immobilized chitosan beads (25 mg) were mixed with 3 ml of hydrogen peroxide solution (10 mM). After 3 min, the reaction was stopped by removal of the beads from the reaction mixture. The absorbance of the reaction mixture was measured and activity of immobilized catalase was calculated. One unit of activity is defined as the decomposition of 1 μmol hydrogen peroxide per min at 25°C and pH 7.0. Activity of free catalase was given as U and immobilized catalase activities were expressed as U/g carrier.
The effect of substrate concentration on the activity was tested by using increasing the concentrations of H2O2; and Vmax and KM values of immobilized and free catalase were determined.
Effect of pH and temperature of free and immobilized catalase
The effect of pH on activities of free and immobilized catalase were determined by using 10 mM H2O2 substrate prepared in 0.05 M sodium acetate (pH: 4.5–5.0), 0.05 M potassium phosphate (pH: 6.5–7.0), and 0.05 M Tris-HCl (pH: 8.0–9.0). The effect of temperature on activities of free and immobilized catalase were investigated at 5–60°C at optimal pH and buffer concentration.
pH stabilities of free and immobilized catalase were examined by measuring the activity of enzyme after the enzyme had incubated in the solutions for 60 minutes at pH 4.0–9.0. For determination of thermal stability, either free or immobilized catalase was incubated in phosphate buffer (50 mM, pH 7.0) for 60 minutes at 20–60°C. Activity of samples were performed at optimum conditions.
Storage stabilities of free and immobilized catalase
Storage stability of the free and immobilized catalase was investigated by measuring their activities after being stored at 4°C and 25°C for 70 days period, and the remaining activity measurement was performed once a week.
Reusability of the immobilized catalase
In order to show the reusability of the immobilized catalase, activity was examined under batch operation mode at 25°C at intervals of 3 min. After measuring each activity, the immobilized catalase was separated from a reaction mixture and washed twice with phosphate buffer (50 mM, pH 7.0), and then immobilized enzymes were added to the fresh reaction medium again. By this way, the next activity measurement was carried out.
Characterization of chitosan and modified chitosan beads
Fourier transform infrared (FTIR) analysis was accomplished on a Perkin Elmer spectrum BX scanning from 4000 cm−1 to 400 cm− 1 at room temperature. Chitosan, chitosan-clay, chitosan-clay-glutaraldehyde, Fe3O4, chitosan-Fe3O4, and chitosan-Fe3O4-glutaraldehyde were mixed with KBr and pressed to plates for measurements.
The magnetization of magnetic chitosan beads were recorded using a Sherwood Scientific magnetometer.
The weight-loss temperatures of the chitosan and modified chitosan beads were determined using a Mettler Toledo TGA analyzer (TGA/SDTA851e).
Results and discussion
Properties of chitosan and modified chitosan beads
The FTIR spectra corresponding to chitosan, chitosan-clay, and chitosan-clay-glutaraldehyde are shown in . As seen in , the peak around 3400 cm−1 observed to the –OH group. The C–H stretching vibration of the polymer backbone is manifested through strong peak at 2925 cm−1 and 2855 cm−1. The stretch vibrations of C–O are found at 1084 cm−1. The characteristic adsorption bands appeared at 1589 cm−1 which can be assigned to N–H bending vibration, peaks at 1399 cm− 1 appeared to –C–O stretching of primary alcoholic group in chitosan. In , between 600–400 cm−1 can be associated respectively with Si–O stretching, and Al–O stretching, and Si–O bending. In , a new sharp peak at 580 cm−1 relates to Fe–O group. For crosslinked chitosan-clay beads, an additional peak at 1656 cm−1 can be observed in c and c, which corresponds to stretching vibrations of C = N bound. This peak indicated the formation of Schiff's base as a result of the reaction between carbonyl group of glutaraldehyde and amine group of chitosan chains.
Figure 1. FTIR spectra of (A) a) chitosan, b) chitosan-clay, c) chitosan-clay-GA; (B) a) Fe3O4, b) chitosan-Fe3O4, c) chitosan-Fe3O4-GA.
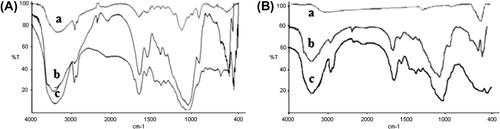
Magnetization of magnetic Fe3O4 nanoparticles and magnetic Fe3O4-chitosan beads were obtained at 25°C. The saturation magnetization of magnetic chitosan beads was about 33.1 emu g−1 while for the magnetic Fe3O4 nanoparticles the magnetization was 80.6 emu g−1. The similar results were indicated by Li et al. (Citation2008). Superparamagnetism is an especially important property needed for magnetic targeting carriers. These results also indicated that the single-domain magnetic nanoparticles remained in these polymer nanoparticles. The magnetic molecules on the surface lack complete coordination and thus increase the surface spin disorientation. This disordered structure in the amorphous materials and at the interface might have caused a decrease in the effective magnetic moment (Li et al. Citation2008).
The thermal stability of the chitosan and its composites has been examined using thermogravimetric analysis and results are shown in . All thermal decomposition profiles exhibit two main stages, with one starting at around 100°C and another at 230°C. The maximum mass loss observed around 210.35°C, 217.12°C and 228.56°C for chitosan, chitosan-clay and chitosan-Fe3O4, respectively. The mass loses for samples chitosan, chitosan-clay, and chitosan-Fe3O4 were 90%, 60%, and 73%, respectively. Chitosan's mass lose was higher than the magnetic chitosan and chitosan-clay at high temperatures. The addition of clay and magnetic Fe3O4 nanoparticles in chitosan usually turn the chitosan more resistant to degradation when compared to the chitosan.
Immobilization of catalase on chitosan and modified chitosan beads
The covalent immobilization of catalase was studied on chitosan and chitosan-composite beads. For this purpose, we modified the amino group of the chitosan and chitosan-composite beads with glutaraldehyde bearing a free aldehyde group. The resulting functional chitosan and chitosan-composite beads were reacted, via Schift's base formation reaction, with the amino groups on the enzyme surface (Bayramoglu et al. Citation2008). It is apparent that activity of immobilized enzyme depends primarily upon the properties of support and immobilization conditions. The high amount of protein loading could cause high activity, however, the increases in the amount of binding enzyme indicated that diffusion limitation, change of enzyme conformation, and its denaturation under more acidic and alkali conditions also affected the ultimate activity in immobilization (Jiang et al. Citation2005). Under the optimal immobilization conditions, immobilization yield and coupling yield were calculated and the results are shown in .
Table I. Values of coupling yield, immobilization yield, and kinetic parameters (KM and Vmax) of free and immobilized catalase.
Chitosan can be easily crosslinked by reagents such as glutaraldehyde and epichlorohydrin. Glutaraldehyde is a very reactive crosslinking reagent and the use of increasing concentrations of it as a support may result in matrix with different internal structures (Adriano et al. Citation2005). In this study, chitosan and chitosan composite beads were prepared and treated with glutaraldehyde concentrations ranging from 0.5% to 5% in order to study the effect of cross-linking reagent on the immobilized derivative. As the concentration increased, the activity of immobilized catalase kept rising, and immobilized catalase activity peaked at 3% of glutaraldehyde concentration. This was accepted as the optimal concentration of the crosslinking reagent for the preparation of the chitosan and modified chitosan beads.
Effect of pH and temperature on the catalytic activity
The effect of pH on the activity of the free and immobilized catalase was examined in the pH range of 4.0–9.0 at 25°C and the results are shown in . As can be seen from these curves, both free and immobilized enzymes have the same pH optimum. The optimum pH was found to be 7.0 for both free and immobilized enzymes. Generally, the pH profiles of the immobilized and free catalase are similar, but the immobilized enzymes have a broader pH range of high activity. The stability of immobilized enzymes at low pH could be attributed to the amino/imine groups, because they can be bound to a proton in the matrix, resulting in a decrease of local concentration of proton near the enzyme molecules (Pan et al. Citation2009). Çetinus and Öztop (Citation2003) reported that immobilized catalase on crosslinked chitosan beads showed optimum pH at 7.0.
Figure 3. The effect of pH (A) and pH stability (B) (after 60 min.) on free and immobilized catalase (-▲-: free enzyme, -○-: immobilized catalase on chitosan, -●-: immobilized catalase on chitosan-clay, -◊-: immobilized catalase on chitosan-Fe3O4). Values are means of three replicates.
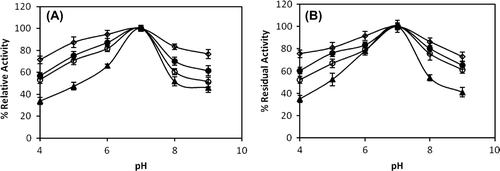
The effect of temperature on the free and immobilized catalase activities was investigated by using H2O2 as substrate as shown in . The temperature profile of the immobilized catalase was broader at higher temperature compared to the free counterpart. The optimum temperature was found to be 35°C for both free and immobilized catalase at pH 7.0. Both of the free and immobilized enzyme activities decreased at high and low temperatures. The loss in the activity of immobilized catalase was lower than that of the free catalase at high temperatures. Similar results were reported by other researchers (Bai et al. Citation2006).
Figure 4. The effect of temperature (A) and temperature stability (B) (after 60 min.) on free catalase and immobilized catalase at pH 7.0 (-▲-: free enzyme, -○-: immobilized catalase on chitosan, -●-: immobilized catalase on chitosan-clay, -◊-: immobilized catalase on chitosan-Fe3O4). Values are means of three replicates.
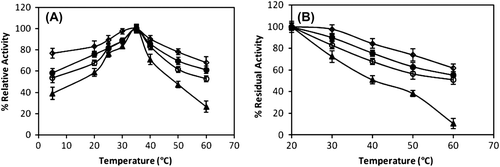
pH, thermal and storage stability of the free and immobilized enzymes
pH stability studies were accomplished for free and immobilized catalase, which were incubated at various pH for 60 min. As seen in , the immobilized enzymes showed more pH stability than the free enzymes. Catalase immobilized on Fe3O4-composite chitosan beads was more stable in the pH range between 4.0 and 9.0 than the other immobilized catalases and free catalase.
Thermal stability experiments were carried out with free and immobilized enzymes, which were incubated at various temperatures for 60 min. shows the heat inactivation curves between 20 and 60°C for the free and immobilized enzymes. At 30°C, the immobilized enzyme preserved its initial activity 83%–97%, whereas the free enzyme lost about 28% of its initial activity during a 60-min incubation period. The free enzyme lost many of its activity at 60°C after a 60-min heat treatment, but immobilized catalase still showed its activity more than 57%. These results indicated that thermal stability of immobilized catalase on the magnetic chitosan beads was greater than that of the catalase immobilized on chitosan and chitosan-clay beads. The activity of the immobilized enzyme, especially in a covalently bound system, is more resistant than that of the soluble form against heat and denaturing agents. This might arise from the covalently bound enzyme being protected from conformational changes imposed by heat (Tükel and Alptekin Citation2004).
Immobilized catalase was stored in phosphate buffer (50 mM, pH 7.0) at 4 and 25°C for 70 days. Activities of the catalase immobilized on chitosan, chitosan clay, and chitosan-Fe3O4 after 30 days storage at 25°C were observed as 18%, 38%, and 40%, respectively, while activities of the same immobilized catalases within the same period at 4°C were 44%, 73% and 82% of the initial activity, respectively. Under these storage conditions, at 4°C, catalase immobilized on chitosan, chitosan-clay, and chitosan-Fe3O4 beads lost their activities within 56, 63, and 70 days, respectively. At 25°C, immobilized catalase on chitosan lost its activity after 42 days, whereas chitosan-clay and chitosan-Fe3O4 beads lost their activities after 49 days. Free catalase stored at 4°C lost its activity after 42 days and at 25°C lost its activity after 21 days.
Kinetic parameters of free and immobilized enzymes
The kinetic parameters of free and immobilized enzymes are shown in the . The initial reaction rates of the catalytic decomposition of the substrate were measured at different substrate concentrations with the free and the immobilized catalase. The kinetic data for decomposition of H2O2 was fitted to the Lineweaver-Burk plot of 1/v versus 1/S. Michaelis constant (KM) and the maximum reaction velocity (Vmax) of the free and the immobilized enzymes were calculated. For the immobilized catalase on chitosan, chitosan-clay, and chitosan-Fe3O4, KM values were found to be 33.76 mM, 30.33 mM, and 26.66 mM, respectively. KM value of free catalase was found to be 14.28 mM. The Vmax values of immobilized enzyme on chitosan, chitosan-clay, and chitosan-Fe3O4 were calculated as 141.92 μmol/min, 176.6 μmol/min, and 195.04 μmol/min, respectively. KM value of immobilized catalase on different supports was slightly higher than that of free catalase may be due to diffusional resistance of carrier against substrate and/or products. The changes in the kinetics of immobilized enzymes are controlled by four factors such as 1) change in enzyme conformation; 2) steric effects; 3) microenvironmental; and 4) bulk and diffusional effects. The migration of substrate from the solution to the microenvironment of an immobilized enzyme can be a major factor increase in KM values (Bai et al. Citation2006).
Repeated use of the immobilized catalase
Unlike free enzymes, immobilized enzymes can be easily separated from product solutions and reused (Chang and Juang Citation2005). shows the changes of the activity of immobilized catalase after recycling uses. After 100 times of repeated test, the immobilized catalase on chitosan-clay composite beads maintain over 50% of the original activity and the immobilized catalase on magnetic chitosan beads maintain over 60% of the original activity.
Compared to catalase immobilized on composite chitosan beads, after 100 times of repeated use the immobilized catalase on chitosan beads maintain 20% of its original activity. Reuse of chitosan beads led to swell and break apart, than activity loses was observed. In contrast, chitosan-clay composite beads and magnetic chitosan beads maintain good mechanical properties. The activities of immobilized catalase on chitosan-clay and magnetic chitosan beads were higher than that on chitosan beads. The addition of clay and magnetic Fe3O4 nanoparticules in matrix greatly increased density of the beads, making it more rigid and indissoluble (Chang and Juang Citation2005).
Conclusions
Chitosan and chitosan composite beads were crosslinked by glutaraldehyde and catalase could be immobilized on the surface of beads by formation of the Schiff's base. The immobilized catalase exhibited remarkably improved stability properties to various parameters such as temperature, reuse, and storage time. Optimum pH and temperature did not change. It was found that thermal stability of immobilized catalases was greater than that of the free catalases. Catalase immobilized on magnetic chitosan beads is more stable than catalase immobilized on chitosan and chitosan-clay beads at high temperatures. Magnetic chitosan beads can be stored at 4 and 25°C for long time and can be reused for many times. Compared with nonmagnetic carriers, the magnetic property of the magnetic chitosan beads is a significant advantage for the enzyme immobilization. They can be easily separated from the reaction medium with conventional permanent magnets for the purpose of reuse. This study shows that catalase can be immobilized on composite chitosan beads and can be used for practical applications.
Declaration of interest
The authors report no declarations of interest. The authors alone are responsible for the content and writing of the paper.
This work was supported by Research Grants BAP 2010-106 from Celal Bayar University.
References
- Adriano WS, Filho HC, Silva JA, Giordano RLC, Gonçalves LRB. 2005. Stabilization of penicillin G acylase by immobilization on glutaraldehyde-activated chitosan. Braz J Chem Eng. 22: 529–538.
- Aebi H. 1984. Catalase in vitro. In: Methods in Enzymology. Oxygen Radicals in Biological Systems. Packer L, Ed. Vol. 105. Orlando: Academic Press, pp. 121–126.
- Albarghouthi M, Fara DA, Saleem M, El-Thaher T, Matalka K, Badwan A. 2000. Immobilization of antibodies on alginate-chitosan beads. Int J Pharm. 206:23–34.
- Bai YX, Li YF, Wang MT. 2006. Study on synthesis of a hydrophilic bead carrier containing epoxy groups and its properties for glucoamylase immobilization. Enzyme Microb Technol. 39: 540–547.
- Bayramoglu G, Kiralp S, Yilmaz M, Arica MY. 2008. Covalent immobilization of chloroperoxidase onto magnetic beads: Catalytic properties and stability. Biochem Engin J. 38:180–188.
- Çetinus AŞ, Öztop HN. 2003. Immobilization of catalase into chemically crosslinked chitosan beads. Enzyme Microb Technol. 32:889–894.
- Chang MY, Juang R. 2005. Activities, stabilities, and reaction kinetics of three free and chitosan–clay composite immobilized enzymes. Enzyme Microb Technol. 36:75–82.
- Jiang D, Long S, Huang J, Xiao HY, Zhou JY. 2005. Immobilization of Pycnoporus sanguineus laccase on magnetic chitosan microspheres. Biochem Engin J. 25:15–23.
- Juang R, Wu F, Tseng R. 2001. Solute adsorption and enzyme immobilization on chitosan beads prepared from shrimp shell wastes. Bioresource Technol. 80:187–193.
- Li G, Jiang Y, Huang K, Ding P, Yao LL. 2008. Kinetics of adsorption of saccharomyces cerevisiae mandelated dehydrogenase on magnetic Fe3O4-chitosan nanoparticles. Colloids Surf A Physicochem Eng Aspects. 320:11–18.
- Pan C, Hu B, Li W, Sun YL, Ye H, Zeng X. 2009. Novel and efficient method for immobilization and stabilization of β-d-galactosidase by covalent attachment onto magnetic Fe3O4–chitosan nanoparticles. J Mol Catal B Enzymatic. 61:208–215.
- Tükel S, Alptekin Ö. 2004. Immobilization and kinetics of catalase onto magnesium silicate. Process Biochem. 39:2149–2155.
- Wu FC, Tseng RL, Juang RS. 2002. Adsorption of dyes and humic acid from water using chitosan-encapsulated activated carbon. J Chem Technol Biotec. 77:1269–1279.
- Xiao Y, Zhou X. 2008. Synthesis and properties of a novel crosslinked chitosan resin modified by L-lysine. React Funct Polym. 68: 1281–1289.
- Yuanbi Z, Zumin Q, Jiaying H. 2008. Preparation and analysis of Fe3O4 magnetic nanoparticles used as targeted-drug carriers. Chin J Chem Eng. 163:451–455.