Abstract
Objective: Intra-operative movements due to mechanical ventilation or manipulations are a limiting factor for accurate spinal navigation or robotic-assisted spinal surgery. The purpose of this study was to assess the accuracy of an intra-operative spinal fixation device in an experimental setup.
Materials and Methods: We developed a fixation device, attached to the operating table, that combines soft tissue retraction with spinal process fixation. Using a lumbar spine cadaver, tightness of fixation was evaluated using two measurement systems. Accuracy measurements using changes in spatial co-ordinates of implanted reference markers were performed in three segments, following different manipulations of the spine. In addition, for intra-operative movements of the spine during mechanical ventilation, the range of motion was determined in 10 patients during lumbar interbody fusion.
Results: The spine frame was easy to use and did not restrict screw insertion. Mean deviations of the markers' in all segments were measured at between 0.35 and 0.8 mm, following pedicle screw insertion and lateral traction. Intra-operative range of motion of the spine was measured with a mean value of 8.7 ± 3.3 mm.
Conclusion: Using our spine frame, a rigid fixation following manipulation of the spine was demonstrated. By overcoming the intra-operative movement-dependent inaccuracy, safety in navigated spine surgery and robotic-assisted procedures might be improved.
Introduction
Transpedicle screw insertion may cause neurological, vascular, and mechanical complications. Before the age of spinal navigation, the incidence of incorrect placement of pedicle screws was up to 30% Citation[1–3]. This was improved using computer-assisted techniques Citation[4–8]. Thus, spinal navigation established a new dimension in terms of safety in planning and realization of spinal procedures. However, there are still reports documenting incorrect placement of spinal implants using computer-assisted techniques in up to 4.6% of cases Citation[6]. Despite the development of advanced software, intra-operative difficulties include the registration procedure, identification of characteristic and reproducible anatomical landmarks, and localization of these points in the operating site. However, repeatability and reliability of different navigation systems are also limited by the mobility due to mechanical ventilation and manipulation of the spine during surgery. Tracking systems are used to assess intra-operative movements of the spine, which, however, are often less reliable due to insufficient mechanical fixation at the spinal process and additional restriction within the operating area. In our opinion, intra-operative fixation of the spine might increase the accuracy in spinal computer-assisted procedures and is essential for the application of robotic-assisted spinal surgery. Therefore, we designed a spine frame that minimizes movement-dependent inaccuracy. The aim of our investigation was to prove the rigidity of the spine when fixed in our spine frame in an experimental study. For control measurements, the range of motion during mechanical ventilation was quantified.
Methods
Spine frame
The spine frame described is a newly developed device for intra-operative spinal fixation that prevents intra-operative movement artefacts in computer-assisted spinal surgery. The device combines two methods of intra-operative immobilization: a soft tissue and a bony structure fixation of the operating site. The entire system is directly attached to the operating table by four arms (). The upper and lower arms are linked horizontally by an additional titanium rod, resulting in a frame construction. Moreover, our spine frame is able to offer a platform for navigated instruments during spinal fixation. Previously introduced devices that depend solely upon soft tissue fixation may be integrated into our spine frame. We applied a formerly used wound retractor (Ulrich, Germany) that is immobilized by four adjustable joint arms mounted at the corners of the retractor to connect the other endings to the frame. For bone structure fixation, two titanium bars are rigidly clamped to the spinal processes. Straight titanium arms provide a link between the two clamping bars and the frame, thereby achieving a rigid fixation of the spine (). The entire system, especially the spinal process fixation, is constructed to achieve easy access to the operating site during all surgical procedures for pedicle screw placement. For computer-assisted surgery, a hydraulic arm combined with a navigated instrument holder is attached to the frame and is able to freely adjust, for example, a screwdriver in a pre-planned trajectory.
Figure 1. The spine frame (1) is mounted on the operating table and fixes the bony structures of the spine cadaver in a rigid position via the spinal processes. In front, the Bronze Millenium Arm (2) system (Faro, Stuttgart, Germany) for three-dimensional measurement is shown.
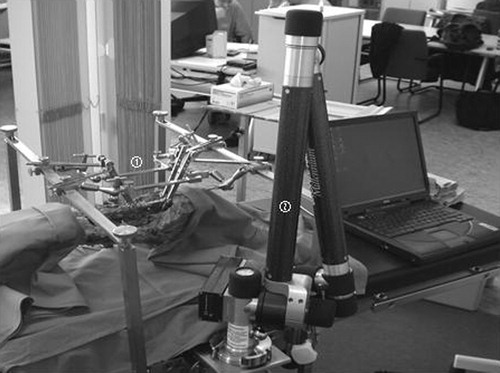
Figure 2. The spine frame includes a soft tissue retractor (1; Ulrich, Germany) that is fixed by four adjustable joint arms (2) to the horizontal rods (3) of the frame. The fixation of the spine is provided by a clamp (4) directly mounted on the spinal processes and linked by titanium arms (5) to the frame.
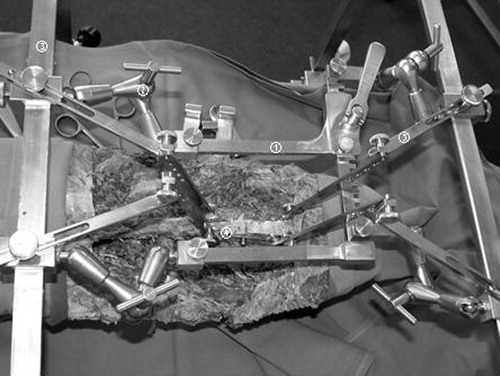
Accuracy measurements
To evaluate the stability of the fixation system, the movement-dependent inaccuracy is determined following different procedures. For this purpose, two lumbar spine cadavers were used. Paravertebral soft tissue was resected to expose the entire dorsal bony structure of the lumbar spine. Three reference markers were placed at the tip of the spinal process and bilaterally at the medial facet joints of each vertebra (L2, L3, and L5). A computer tomography (CT) scan of the lumbar spine was carried out with no gantry tilt and 1 mm slice thickness using a Somatom Plus 4 scanner (Siemens, Erlangen, Germany). The spine frame was mounted on the operating table and the spine cadaver was fixed using the inner spinal process fixation in a position corresponding to that in vivo dorsal spinal surgery. The soft tissue retractor was mounted on the frame to simulate intra-operative conditions and to verify free accessibility for all procedures ().
To determine movement-dependent deviations of the spine position, mean differences were measured by comparing baseline spatial co-ordinates with the marker co-ordinates following different manipulations. For data acquisition, two systems were used. The Bronze Millennium Arm (Faro, Stuttgart, Germany) is a computer-assisted mechanical system that defines spatial co-ordinates. System-related inaccuracy is specified by the manufacturers as 0.168 mm. Baseline co-ordinates were determined before each procedure was carried out. In addition, the navigation pointer system STN 4.0 (Leibinger, Freiburg, Germany) was used by pre-defining virtual co-ordinates in the CT data set. Spatial orientation of the fixed spine was performed by registering the reference markers of the vertebral level L3. Using the navigation system, virtual co-ordinates were set as a baseline. Following registration, the deviation of the marker position from baseline co-ordinates of all segments was determined to measure registration accuracy.
Two different procedures were performed to study movement-dependent inaccuracy. First, two pedicle screws were inserted in L3. Secondly, a controlled lateral traction was applied at L3 with a constant power of 70 N lasting for 30 s. Then, measurements of the reference marker position were performed five times. Distances of the marker positions from their baseline co-ordinates were calculated.
Intra-operative measurements
For control measurements, we quantified intra-operative movements due to mechanical ventilation. For this, the range of motion of the spine was quantified during five ventilation cycles in 10 patients during posterior lumbar interbody fusion procedures with prone positioning. Using the navigation system STN 4.0 (Leibinger, Freiburg, Germany), the tip of a pointer instrument was mounted at the spinal process. The changes in co-ordinate position of the pointer tip were detected during all ventilation cycles. Vector differences of the position during maximal inspiration and expiration were calculated and used as the range of motion.
Statistical analysis
Statistical analysis was performed by analysis of variance, using the software Sigma Stat 3.0 (Jandel Scientific, Erkrath, Germany). All results are given as mean ± standard error of the mean. Differences were significant at p < 0.05.
Results
Installing the entire spine frame system at the operating table lasted ∼6 min. In an experimental setup using the spine cadaver, the device showed good applicability in computer-assisted spinal surgery. Using spinal process and retractor fixation, the working space for instrumentation and screw insertion was not restricted.
Accuracy measurements
The Bronze Arm measured mean deviations of the markers following screw insertion of 0.71 ± 0.16 mm in L2, 0.79 ± 0.06 mm in L3, and 0.74 ± 0.1 mm in L5 (). Following controlled lateral traction, mean deviations were 1.0 ± 0.15 mm in L2, 1.12 ± 0.08 mm in L3, and 1.19 ± 0.1 mm in L5 (). There were no significant differences between the measured values.
Figure 3. The radii of deviation of each segment following pedicle screw insertion at L3 (a) and controlled lateral traction (b) compared with baseline positions are shown as bar diagrams. The deviation of reference markers measured by the Faro arm (light bars) is lower or merely 1 mm in all segments following both procedures. Measurements using the navigation system (dark bars) show adequately low deviations in the registered L3 segment after both manipulations. Deviations in the neighboring segments are significantly higher according to the system- and registration-related inaccuracy (results are given as mean ± standard error of the mean, *p < 0.01).
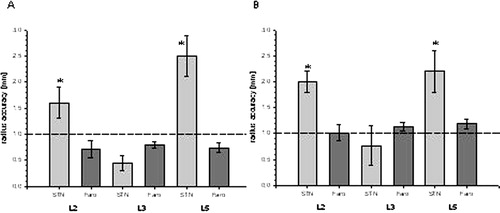
In navigation pointer system measurements, mean deviations in L3 were 0.35 ± 0.16 mm following registration, 0.45 ± 0.15 mm after screw insertion (), and 0.77 ± 0.38 mm following lateral traction (). In the neighboring segments, significantly higher deviations were measured (p < 0.05). In L2, deviations of 1.2 ± 0.3, 1.6 ± 0.3, and 2 ± 0.2 mm were calculated following the aforementioned procedures. In L5, deviations were 2.5 ± 0.3, 2.5 ± 0.4, and 2.2 ± 0.4 mm, respectively ( and ).
Intra-operative spinal movements
During five mechanical ventilation cycles in 10 patients, the motion of the spine was measured. The range of motion was determined to be between 1.3 and 18.1 mm in all patients. Mean range of motion was 8.7 ± 3.3 mm in all patients.
Discussion
Robotic systems are commonly used for orthopaedic hip replacement. In spinal surgery, robotic techniques might be helpful when implanting pedicle screws. Recently, navigation has become widely used in pedicle screw insertion. We present a retractor-based fixation system to prevent unwanted movement artefacts in spinal navigation and robotics.
The fixation system was constructed as a rigid system fixed to the operating table with a spinal process fixation device and soft tissue retractor. A comparable fixation system, such as the SynFrame, is used for soft tissue retraction in ventral and dorsal spine surgeries Citation[9], Citation[10]. This system was suggested for use as a platform for computer-guided procedures Citation[9]. However, owing to the SynFrame's ability to fixate only soft tissue, it does not stabilize the spine itself and hence intra-operative movements are not prevented.
In our opinion, in order to improve the accuracy in spinal navigation and robotics, the highest measure of spinal rigidity must be achieved. The widely used tracking systems for spinal navigation are limited in terms of compensation for all movements and manipulations related to changes in spatial orientation Citation[11], Citation[12]. One type of restriction that these tracking systems demonstrate is insufficient fixation at the spinal process itself. In addition, the camera's view of the dynamic reference frame may not be interrupted at any time during the navigation procedure. Moreover, from our experience, a greater degree of inaccuracy was observed using a tracking system in navigated brain surgery when compared with a procedure using a Mayfield clamp and a rigidly fixed operating table. A tracking system is able to overcome movement artefacts due to mechanical ventilation during a navigated surgical procedure. We quantified a mean range of motion of the lumbar spine during mechanical ventilation of 8.7 mm with maximum deviations of 18.1 mm. A tracking system is able to follow these changes in position of the navigated object. However, for robotic-assisted procedures, a continuous virtually repositioning of a moving object would imply that the robot also needs to follow these movements during the intervention. Even if this can be realized technically, a high risk of inaccuracy might be present. We postulate that an enhanced rigidity of the spine during navigated and robotic-assisted procedures will increase the accuracy of these interventions. As a result, we developed a spine frame as an intra-operative fixation system combining a soft tissue and spinal fixation retractor system that is mounted on the operating table.
In this cadaver study, the complete retractor system was attached to the spine frame in order to simulate clinical conditions while emphasizing the spatial freedom for all surgical procedures (). We could prove that access to the pedicles and different angles for implantation of pedicle screws were possible using the spine frame. However, clinical experience is necessary to evaluate the system. Following a learning curve, the installation of the spine frame on the operating table, the positioning of the retractor system, and the fixation of the spinal process took ∼6 min.
Moreover, using different systems of accuracy measurement, we demonstrated the high rigidity of the system following implantation of pedicle screws and controlled lateral traction to the spine. Using the navigation tool, higher inaccuracy could be observed in the neighboring segments. Measurements with the Bronze Arm showed rigidity in all segments of the fixed spine following the various procedures. These data prove that the spine is sufficiently stabilized using the spine frame. Following all procedures, deviations of the spatial position of the fixed spine were ≤1 mm. The deviations measured by the navigation system in the neighboring segments were interpreted as system-related, as it was shown that accuracy decreases with increased distance from the reference points Citation[13]. As long as anatomical landmarks are used as reference points and additional surface matching is performed at one segment in a clinical setup, inaccuracy related to the distance from the area of registration will still be present. However, this factor could be eliminated in our experimental setup using the Bronze Arm, which showed equally small deviations in all segments following the different procedures. Thus, movement-dependent inaccuracy is stable in all segments using the intra-operative fixation system. In addition, under clinical conditions, inaccuracy in neighboring segments may also result from differing positions of the patient during image acquisition and during surgery. Thus, a monosegmental registration for navigated procedures is still necessary. In conclusion, our spine frame might improve accuracy and stability in navigated spine surgery and robotics. As compared to dynamic reference-based systems, the intra-operative fixation should prevent movement-dependent inaccuracy during mechanical ventilation and surgical manipulation.
References
- Castro W. H., Halm H., Jerosch J., Malms J., Steinbeck J., Blasius S. Accuracy of pedicle screw placement in lumbar vertebrae. Spine 1996;; 21(11)1320–1324
- Esses S. I., Sachs B. L., Dreyzin V. Complications associated with the technique of pedicle screw fixation. A selected survey of ABS members. Spine 1993; 18(15)2231–2239, discussion 2238–2239.
- Gertzbein S. D., Robbins S. E. Accuracy of pedicular screw placement in vivo. Spine 1990;; 15(1)11–14
- Kalfas I. H., Kormos D. W., Murphy M. A., McKenzie R. L., Barnett G. H., Bell G. R., Steiner C. P., Trimble M. B., Weisenberger J. P. Application of frameless stereotaxy to pedicle screw fixation of the spine. J Neurosurg 1995;; 83(4)641–647
- Laine T., Schlenzka D., Makitalo K., Tallroth K., Nolte L. P., Visarius H. Improved accuracy of pedicle screw insertion with computer-assisted surgery. A prospective clinical trial of 30 patients. Spine 1997;; 22(11)1254–1258
- Laine T., Lund T., Ylikoski M., Lohikoski J., Schlenzka D. Accuracy of pedicle screw insertion with and without computer assistance: a randomised controlled clinical study in 100 consecutive patients. Eur Spine J. 2000;; 9(3)235–240;, discussion 241
- Merloz P., Tonetti J., Pittet L., Coulomb M., Lavallee S., Sautot P. Pedicle screw placement using image guided techniques. Clin Orthop 1998;; 354: 39–48
- Schwarzenbach O., Berlemann U., Jost B., Visarius H., Arm E., Langlotz F., Nolte L. P., Ozdoba C. Accuracy of computer-assisted pedicle screw placement. An in vivo computed tomography analysis. Spine 1997;; 22(4)452–458
- Aebi M., Steffen T. Synframe: a preliminary report. Eur Spine J 2000;; 9(Suppl 1)S44–S50
- Kossmann T., Jacobi D., Trentz O. The use of a retractor system (SynFrame) for open, minimal invasive reconstruction of the anterior column of the thoracic and lumbar spine. Eur Spine J. 2001;; 10(5)396–402
- Braun V., Rath S. A., Antoniadis G., Richter H. P. In vivo experiences with frameless stereotactically guided screw placement in the spine—results from 75 consecutive cases. Neurosurg Rev 2001;; 24(2–3)74–79
- Glossop N. D., Hu R. W., Randle J. A. Computer-aided pedicle screw placement using frameless stereotaxis. Spine 1996;; 21(17)2026–2034
- Fitzpatrick J. M., West J. B., Maurer C. R. Jr. Predicting error in rigid-body point-based registration. IEEE Trans Med Imaging 1998;; 17(5)694–702