Abstract
Objective: To validate a new non-invasive CT method for measuring segmental translations in lumbar spine in a phantom using plastic vertebrae with tantalum markers and human vertebrae.
Material and Methods: One hundred and four CT volumes were acquired of a phantom incorporating three lumbar vertebrae. Lumbar segmental translation was simulated by altering the position of one vertebra in all three cardinal axes between acquisitions. The CT volumes were combined into 64 case pairs, simulating lumbar segmental movement of up to 3 mm between acquisitions. The relative movement between the vertebrae was evaluated visually and numerically using a volume fusion image post-processing tool. Results were correlated to direct measurements of the phantom.
Results: On visual inspection, translation of at least 1 mm or more could be safely detected and correlated with separation between the vertebrae in three dimensions. There were no significant differences between plastic and human vertebrae. Numerically, the accuracy limit for all the CT measurements of the 3D segmental translations was 0.56 mm (median: 0.12; range: −0.76 to +0.49 mm). The accuracy for the sagittal axis was 0.45 mm (median: 0.10; range: −0.46 to +0.62 mm); the accuracy for the coronal axis was 0.46 mm (median: 0.09; range: −0.66 to +0.69 mm); and the accuracy for the axial axis was 0.45 mm (median: 0.05; range: −0.72 to + 0.62 mm). The repeatability, calculated over 10 cases, was 0.35 mm (median: 0.16; range: −0.26 to +0.30 mm).
Conclusion: The accuracy of this non-invasive method is better than that of current routine methods for detecting segmental movements. The method allows both visual and numerical evaluation of such movements. Further studies are needed to validate this method in patients.
Introduction
Numerous methods for measuring segmental motion in lumbar spine have been described by many authors Citation[1–5]. However, because of the inaccessibility of the spine and the complexity of segmental movements, most of the non-invasive methods in use today have low accuracy or are unable to detect movements in all three cardinal axes. Almost all in vivo methods used for analyzing segmental motion are based on two-dimensional (2D) radiographic examinations. Measurements on conventional radiographs have an accuracy of 1.2–4.0 mm for translation and 1–3° for rotation, depending on which technique is employed Citation[6–8]. In biplanar radiography, orthogonal radiographs with coordinates for anatomical landmarks on the vertebrae in both projections have been used to calculate three-dimensional (3D) movements with higher precision Citation[9]. RSA techniques with implantation of tantalum bullets have also been used. The accuracy with this technique in vivo is 0.3 mm for the vertical axis, 0.6 mm for the transverse axis, and 0.7 mm for the sagittal axis Citation[10]. The aim of this study was to evaluate the accuracy of a new non-invasive method for measuring segmental movement in the spine using Computed Tomography (CT).
Material and methods
A phantom incorporating three lumbar vertebrae (L3-L5) was constructed (). The L4 and L5 vertebrae were rigidly mounted, and L3 was mounted so that it could be translated along its three cardinal axes separately using screws with inclination of 1 mm per revolution, thereby simulating segmental movements along the cardinal axes of the vertebra. The phantom was examined using a LightSpeed QX/i fourth-generation spiral CT unit (General Electric Medical Systems, Milwaukee, WI). Images were acquired with 1.25-mm collimation and a pitch of 3 (0.75 mm/rotation), at 250 mA, 120kV, from L3 to L5. Images were reconstructed with 1.25-mm increments. The phantom underwent a total of 104 CT scans.
Figure 1. The phantom. Three vertebrae are attached to a base plate. L4 and L5 are secured rigidly to the base plate, and L3 is translated using screws.
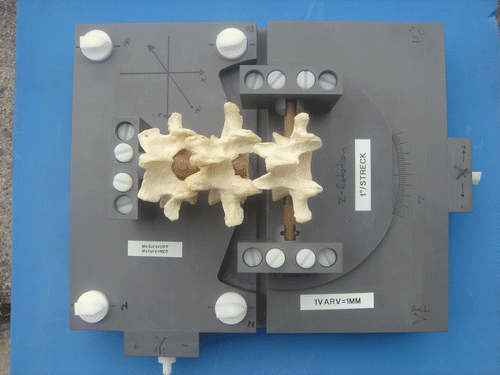
For the first 54 CT scans, plastic vertebrae were used. The plastic vertebrae themselves do not produce any “condensed areas” on CT scans, so we prepared all plastic vertebrae with spherical tantalum markers with a diameter of 0.8 mm, evenly distributed through the volumes of the vertebrae to simulate condensed areas. Also, a disc prosthesis (ProDisc-L, Synthes, Switzerland) was also incorporated between L3 and L4 in the plastic vertebrae to facilitate future studies with this method.
The last 50 CT scans were performed on human vertebrae collected from the Anatomical Institution at the Karolinska Institute. Translations of these vertebrae were carried out by using the inclination screws that made the L3 vertebra translate along different axes. In the vertical and sagittal axes, translation was performed in a series of 0.5-mm increments between the different CT scans. In the transverse direction, the translation increments were 1 mm between scans. Between examinations, the position of the phantom in the CT scanner was altered to simulate different patient positions.
To assess the precision of translations in the phantom, a micrometer (CD-15CR, Mitutoyo Corporation, Japan) with a precision of 0.05 mm was used during the first 32 examinations. The micrometer was used to measure distances relative to the base plate in the phantom that directly correlated to the translation of the L3.
The CT volumes from different scans were combined into 64 cases, each comprising a pair of CT volumes () and characterized as follows:
No segmental movement, plastic vertebrae (12 cases)
No segmental movement, human vertebrae (12 cases)
Segmental movement 0.5–3.0 mm, plastic vertebrae (30 cases)
Segmental movement 1–2 mm, human vertebrae (10 cases)
Table I. Plastic and human vertebrae in different 3D translation positions in the phantom.
Volume registration (the process of bringing information from two separate volumetric data sets into spatial alignment) was carried out on pairs of CT volumes by a single examiner (an orthopedic surgeon) using a 3D volume fusion tool that has been extensively validated previously Citation[9], Citation[11], Citation[12]. This semi-automated tool has a graphic interface and is used for performing landmark-based fusion of two volumes, registering the “target” volume with the “reference” volume. In this case, a rigid-body transformation (i.e., limited to translation and rotation) was used. A technical description of this tool is provided below. The image analysis was performed on a mobile workstation (Precision M60, Dell Computer Corporation, Round Rock, TX).
Image analysis
To speed up the data processing for the plastic vertebrae, we simplified the images by creating 256 * 256 voxel image slices from the original 512 * 512 slices. Using the volume fusion tool, nine corresponding landmarks were placed in the L4 vertebra in each case. In the plastic vertebrae the landmark set corresponded to the tantalum markers. In the human vertebrae there were several condensed areas (the trabecular bone confluences, which create a specific pattern in the vertebra) that could be used for designating landmarks, and as with the plastic vertebrae the landmarks were distributed evenly throughout the vertebrae. The landmarks were placed using a 3D sphere while viewing the data from three orthogonal points of view (i.e., vertical, transverse and sagittal), and checked in the same views (). A transformed volume was created and the degree of overlap of the L4 vertebra in the reference and transformed volumes was visually inspected in 2D and 3D overlays. If the L4 vertebrae were mis-matched, the landmarking was augmented by moving landmarks and a new transformation and visual check were performed. When the L4 vertebra was considered to be well-matched, then a new set of co-homologous landmarks was placed in the L3 vertebra in both the reference volume and the transformed volume. These sets also consisted of nine landmarks.
Figure 2. (a) Simultaneous display of two CT volumes (reference [left] and target [right]) in axial, coronal and sagittal views. A single landmark in the L4 verterbra in each volume is shown (blue diamond) on the orthogonal slices that intersect it. Co-homologous points are designated in both volumes and used to bring the L4 in the target volume into spatial alignment with the L4 in the reference volume. For this study, nine landmarks in L4 where used. (b) Close-up coronal view of human vertebra with co-homologous landmarks shown (orange diamonds) in the reference (top) and target (bottom) volumes. [Color version available online.]
![Figure 2. (a) Simultaneous display of two CT volumes (reference [left] and target [right]) in axial, coronal and sagittal views. A single landmark in the L4 verterbra in each volume is shown (blue diamond) on the orthogonal slices that intersect it. Co-homologous points are designated in both volumes and used to bring the L4 in the target volume into spatial alignment with the L4 in the reference volume. For this study, nine landmarks in L4 where used. (b) Close-up coronal view of human vertebra with co-homologous landmarks shown (orange diamonds) in the reference (top) and target (bottom) volumes. [Color version available online.]](/cms/asset/7660d64d-0f7f-4acd-8116-18187433429b/icsu_a_288423_f0002_b.gif)
Finally, the outcome of the image analysis with respect to both visual and numerical results was compared to the known translations in the model. For numerical evaluation of the measured translation of L3, the differences between corresponding L3 landmarks in the reference and transformed volumes were calculated and expressed as vectors. All nine L3 landmarks were used in the calculations. The average of all nine translations was then calculated, along with the total 3D distance and the distance along the cardinal axes. Three-dimensional images of the two L3 vertebrae were constructed and their relative positions viewed. For comparisons between phantom and CT data, the intended translations in the phantom, as established by using the screws, were accepted as the true values. These were determined to be sufficiently accurate, based on the initial measurements of actual translations in the model using a micrometer. The difference in three dimensions and along the cardinal axes between true translation and the translation as measured on the CT volumes was calculated for all cases. To evaluate any significant difference between the plastic vertebra volumes and the human vertebra volumes, we used the Mann-Whitney U-test, with the significance level set to P < 0.05. The datasets were tested for normality using graphical evaluation. Repeatability (10 plastic cases) and accuracy (all cases) limits for segmental translations were calculated. The repeatability limit was defined as the value less than or equal to which the absolute difference between two test results obtained under repeatability conditions may be expected to have a probability of 95%. The accuracy limit was defined as the value below which 95% of all errors are expected to fall, assuming normal distribution and no bias Citation[13]:
Technical note on volume fusion tool
The user interface of the volume fusion tool presents arbitrarily chosen slices from two volumes simultaneously. Two larger views representing corresponding slices in one of the orthogonal planes, or six smaller views representing corresponding slices in all three planes (vertical, transverse and sagittal) can be displayed (). Two window width/level settings provide a lower window, which can be used for viewing the skeletal structure, and a higher window for simultaneously viewing metal or other high-attenuating structures. A simultaneous 3D isosurface (a 3D shaded surface) can also be displayed (). Landmarks are chosen on concurrently viewed slices which display the same physiological point or structure, or directly on the 3D isosurface. When a landmark is chosen, the corresponding point in the 3D volume is recorded in distance units independent of any voxel location, and a sequence number is generated. The 3D paired landmarks are then used to generate the eigenvalues of the matrix of coefficients for the transformation. A weighted least square linear regression (which limits the effect of mismatched points) followed by a Gauss-Jordan matrix inversion is used to generate transform coefficients for arbitrary volume data sets. Finally, a transformation is performed. For the present study, a rigid body transform was used; this type of transformation preserves the spatial integrity of structures involved. Once the new transformed volume has been created from the original, untransformed data set, this new volume may be re-sliced and evaluated side by side with the reference volume in any of the three planes, or superimposed on the reference slices. An isosurface of the transformed volume may be displayed in three dimensions, superimposed with an isosurface from the reference volume and/or the target volume for comparison (). This may be done in large or small format and the volumes can be rotated and viewed from an arbitrary direction. The transformed volume may also be landmarked together with the reference volume, either to create a better match or, if the match is considered adequate, to generate a numerical correlation of migration. The latter is done by landmarking (with a point of sphere landmark) corresponding structures in the image of the implanted components in the reference and transformed volumes. The sphere landmark superimposes the contours of a 3D sphere and generates the 3D coordinates for the sphere center ().
Figure 3. (a) A 3D display of L4 after registration (case 34). Note the overlapping “zebra-like” pattern between the reference volume isosurface (yellow) and the transformed volume isosurface (green) created when the two surfaces coincide. This pattern indicates that the registration is better than the smallest image element (voxel) in the volumes. (b) A 2D overlay axial view where the gray (left) side of the vertebra is the reference volume and the red (right) side is an overlay of the transformed volume on the reference volume. Note the close match between all the condensed areas of the vertebra (case 34). [Color version available online.]
![Figure 3. (a) A 3D display of L4 after registration (case 34). Note the overlapping “zebra-like” pattern between the reference volume isosurface (yellow) and the transformed volume isosurface (green) created when the two surfaces coincide. This pattern indicates that the registration is better than the smallest image element (voxel) in the volumes. (b) A 2D overlay axial view where the gray (left) side of the vertebra is the reference volume and the red (right) side is an overlay of the transformed volume on the reference volume. Note the close match between all the condensed areas of the vertebra (case 34). [Color version available online.]](/cms/asset/152bc40c-fcef-4c23-9ec4-f85c03fc6a37/icsu_a_288423_f0003_b.gif)
Results
The L4 vertebra could be successfully registered in all cases. This was confirmed with 3D superimposed isosurfaces, where a specific “zebra-like” pattern is obtained when the surfaces coincide (a). This pattern was obtained for the registered L4 vertebrae in all cases, indicating that the L4 was almost perfectly matched. When the 2D slices were superimposed, the registered vertebrae also showed an almost perfect match in all cases (). In all cases with zero translation of the L5 vertebrae, the L5 was also nearly perfectly matched on visual inspection (a). In cases with translation of 0.5 mm, some separation between the L3 vertebrae could be detected, but not enough to be certain of the direction of translation (). In cases with at least 1 mm of translation, we could visually detect both separation and the direction of the translation of the L5 vertebrae in all cases ().
Figure 4. Three-dimensional isosurface displays after registration of L4. (a) A case with no movement of L3, showing “zebra” pattern between the reference (yellow) and transformed vertebrae (green) in both L4 and L3. A disc prosthesis is incorporated between the vertebrae for future studies. (b) A 0.5-mm sagittal translation of L5. (c) A 1.0-mm sagittal translation of L3. (d) A 2.0-mm sagittal translation of L3. [Color version available online.]
![Figure 4. Three-dimensional isosurface displays after registration of L4. (a) A case with no movement of L3, showing “zebra” pattern between the reference (yellow) and transformed vertebrae (green) in both L4 and L3. A disc prosthesis is incorporated between the vertebrae for future studies. (b) A 0.5-mm sagittal translation of L5. (c) A 1.0-mm sagittal translation of L3. (d) A 2.0-mm sagittal translation of L3. [Color version available online.]](/cms/asset/3304e997-10c1-40ef-9bce-23ed1a8ba698/icsu_a_288423_f0004_b.gif)
Therefore, it can be concluded that it was possible to detect segmental movements visually with this method. There was no detectable difference between the plastic vertebrae and human vertebrae with regard to the visual evaluation of translations.
Numerical evaluation
The accuracy of the phantom translation using screw translations, as compared to micrometer measurements, was 0.1 mm. Therefore, it was acceptable to use the intended translation with cardinal screws as the true values. CT measurements are contrasted to phantom measurements in There were no significant differences between plastic and human vertebrae. CT measurements of lumbar segmental translation compared to phantom data showed a near normal distribution when evaluated graphically. The accuracy limit for all the CT measurements of the 3D translations was 0.56 mm (median: 0.12; range: −0.76 to + 0.49 mm). In the clinically important sagittal axis, accuracy was 0.45 mm (median 0.10; range: −0.46 to + 0.62 mm). The accuracy for the coronal axis was 0.46 mm (median: 0.09; range: −0.66 to + 0.69 mm), and the accuracy for the axial axis was 0.45 mm (median: 0.05; range: −0.72 to + 0.62 mm). Repeatability, when 10 plastic cases were analyzed twice, was 0.35 mm (median: 0.16; range: −0.26 to + 0.30 mm) ().
Table II. Repeatability. Difference (in mm) when 10 cases where analyzed twice under repeatability conditions.
Discussion
The relationship between spinal kinematics, degeneration, and segmental movements in low back pain is still not clear. Morphological changes probably cause pathological segmental movements that may be one of the components of low back pain. However, assessment of segmental movement in the lumbar spine on radiographs is difficult. The accuracy when measuring segmental movement is dependent on the method used. The most common methods employ lateral-view radiographs, but there are several sources of errors in such approaches. For example, if the film or vertebrae is laterally tilted or off-center from the central beam, an incorrect image of the vertebrae endplate is induced Citation[14]. Non-invasive biplanar radiography, where landmarks are detected on plain films, is more accurate and enables measurement of segmental movement along all three cardinal axes. This method was introduced in the mid 1980s and, with accuracy of approximately 1.4 mm, can be used to detect translations in all cardinal planes. However the analysis is complicated and special equipment is needed Citation[9]. There are other radiographic methods, such as radiostereometry, that can detect small 3D changes in implant position, but they are invasive, complicated, and costly Citation[10]. Therefore, a more sensitive radiographic technique that could assess 3D segmental movements of the lumbar spine with a higher accuracy than conventional radiography would help provide a better understanding of the role of segmental movements in low back pain. It would also contribute to the understanding of how different implants influence the segmental movement.
The method presented here for measuring segmental movement in the lumbar spine using CT has several advantages. It is truly three-dimensional, and the image-handling toolkit used in this study is highly portable and not dependent on the model of CT scanner used Citation[15]. The accuracy found in this study, 0.56 mm for 3D movement and 0.45 mm in the sagittal axis, is significantly better than that reported for any non-invasive biplanar radiographic method or any lateral-view method. The results of the phantom study indicate that this method may be very promising, and clinical studies should therefore be undertaken. The repeatability was 0.37 mm, which is almost the same as the results obtained in earlier studies on Total Hip Arthroplasty with this method Citation[16]. We have shown previously that inter-observer errors with this method are small, and we assume that the error is the same when applied to the spine Citation[17]. Since the combined error was small and this was a phantom situation, we did not systematically explore how much random error was generated in each step. However, there were no indications of systematic errors; only normally distributed random errors were found when analyzing all the cases.
Plastic vertebrae have no condensed areas, so we had to use tantalum markers to designate landmarks. In our experience, these were no easier to match than bony structures in the human vertebra. The Mann-Whitney U-test did not indicate any significant difference between the plastic or human volumes. The number of landmarks chosen on the vertebrae was fixed at nine. With nine landmarks, it was possible to obtain a reasonable spread in three dimensions. Use of more landmarks would probably improve the accuracy, but would also be more time-consuming, and greater accuracy is not clinically necessary. To gain acceptance for this method among clinicians, resolution has to be lowered. We have already performed several cases for future studies with low-resolution CT scans on humans, and there is no problem detecting condensed areas for landmarking.
The possibility of visually evaluating 3D segmental movement is an option that we find contributes to the understanding of segmental translation. To our knowledge, there are no other methods that can present such visualization of segmental movement in three dimensions and superimposed on slices. We believe this may be clinically useful for evaluation of different devices used in spine surgery.
Our method can be used on any CT volumes, i.e., data can be acquired wherever there is a CT scanner. At present, the position of the patient will influence the direction of the measured movement. In previous studies on hip implants, we addressed this issue by first re-orienting the data volume so that the CT volume coordinate system became parallel to the pelvic coordinate system. This meant that the patient could be placed in the CT scanner in any position without influencing the results. There is a possibility of defining the same standard orientation for the spine so that the cardinal axes are aligned before analyzing data. As previously mentioned, this was not done in the current study, which meant that movements that were not in the direction of the cardinal axes would induce movement in other directions. Having a defined standard orientation would improve accuracy and repeatability, and hence it will be included in future studies so that translations and rotations can be presented in the three cardinal axes independent of patient positioning during scanning.
In summary, we have reported results from a model study of a new non-invasive CT-based method for measuring lumbar spine movements. Specific advantages of this method are that movement is analyzed both visually and numerically in three dimensions. We believe our method can be useful both in clinical practice and in research applications.
References
- Axelsson P, Karlsson BS. Standardized provocation of lumbar spine mobility: Three methods compared by radiostereometric analysis. Spine 2005; 7: 792–797
- Boocock MG, Jackson JA, Burton AK, Tillotson KM. Continuous measurement of lumbar posture using flexible electrogoniometers. Ergonomics 1994; 37: 175–185
- Dvorak J, Panjabi MM, Chang DG, Theiler R, Grob D. Functional radiographic diagnosis of the lumbar spine. Flexion-extension and lateral bending. Spine 1991; 16: 562–571
- Paquet N, Malouin F, Richards CL, Dionne JP, Comeau F. Validity and reliability of a new electrogoniometer for the measurement of sagittal dorsolumbar movements. Spine 1991; 16: 516–519
- Steffen T, Rubin RK, Baramki HG, Antoniou J, Marchesi D, Aebi M. New technique for measuring lumbar segmental motion in vivo: method, accuracy, and preliminary results. Spine 1997; 22(2)156–166
- Leivseth G, Brinckmann P, Frobin W, Johnsson R, Strömkvist B. Assessment of sagittal plane segmental motion in the lumbar spine. A comparison between distortion-compensated and stereophotogrammetric roentgen analysis. Spine 1998; 23: 2648–2655
- Saraste H, Brostrom LA, Aparisi T, Axdorph G. Radiographic measurement of the lumbar spine. A clinical and experimental study in man. Spine 1985; 10: 236–241
- Wall JL, Oppenheim WL. Measurement error of spondylolisthesis as a function of radiographic beam angle. J Pediatr Orthop 1995; 15: 193–198
- Pearcy M, Portek I, Shepherd J. Three-dimensional x-ray analysis of normal movement in the lumbar spine. Spine 1984; 9: 294–297
- Johnsson R, Selvik G, Strömkvist B, Sunden G. Mobility of the lower lumbar fusion evaluated by roentgen stereophotogrammetric analysis. Spine 1990; 15: 347–350
- Noz ME, Maguire GQ Jr, Zeleznik MP, Kramer EL, Mahmoud F, Crafoord JA. Versatile functional-anatomic image fusion method for volume data sets. J Med Syst 2001; 25: 297
- Olivecrona H, Weidenhielm L, Olivecrona L, Noz ME, Maguire GQ Jr, Zeleznik MP, Svensson L, Jonson T. Spatial component position in Total Hip Arthroplasty. Accuracy and repeatability with a new CT method. Acta Radiol 2003; 44: 84–91
- Ranstam J, Ryd L, Önsten I. Accurate accuracy assessment. Acta Orthop Scand 2000; 71(1)106–108
- Frobin W, Brinkmann P, Leviseth G, Biggemann M, Reikerås O. Precision measurement of segmental motion from flexio-extension radiograph of lumbar spine. Clin Biomech 1996; 8: 457–465
- Noz ME, Maguire GQ Jr. A minimal but highly portable image display and handling toolkit. Computer Meth Programs Biomed 1988; 27: 229
- Olivecrona L, Olivecrona H, Weidenhielm L, Noz ME, Maguire GQ Jr, Zeleznik MP. Model studies on acetabular component migration in total hip arthroplasty using CT and a semi-automated program for volume merging. Acta Radiol 2003; 44: 419–429
- Olivecrona H, Olivecrona L, Weidenhielm L, Noz ME, Maguire GQ Jr, Zeleznik MP, Svensson L, Jonson T. Stability of acetabular axis after total hip arthroplasty; repeatability using CT and a semiautomated program for volume fusion. Acta Radiol 2003; 44: 653–661