Abstract
We developed a robot-assisted fracture reduction system (FRAC-Robo) to assist anatomical reduction and to maintain reduction during internal fixation while recording the procedure in a log. We conducted two experiments before using FRAC-Robo clinically. In the first experiment using the FRAC-Robo system, we measured the maximum force and torque required to pull and rotate the limbs of healthy conscious volunteers until they felt pain or abnormality. The average maximum traction force applied to the lower limb was 250.7 N, and the average maximum torque was 5.6 Nm in internal rotation and 7.6 Nm in external rotation for 30° of abduction of hip. In the second experiment, we measured the traction force and rotation torque during the reduction of proximal femoral fractures. The average traction force and rotation torque needed for reduction were 215.9 N and 3.2 Nm, respectively. On the basis of these results, we consider that FRAC-Robo can generate sufficient force and torque to reduce femoral fractures safely.
Introduction
Hip fractures are a common type of fracture in the elderly, and the number of hip fractures is increasing as the elderly population increases. Hip fractures are classified into intertrochanteric and femoral neck fractures: patients with intertrochanteric fractures tend to be older and more osteoporotic than those with femoral neck fractures.
The outcome of treatment for intertrochanteric fractures has largely been related to the quality of reduction and the devices used for fixation Citation[1]. Despite recent advances in surgical techniques and fixation devices, poor fracture reduction still affects postoperative morbidity and mortality in cases with intertrochanteric fractures. The reduction maneuver combines traction, rotation of the patient's limb, and judgment of fracture reduction using fluoroscopic guidance. This occasionally results in inappropriate reduction and fixation of the femur, leading to complications such as screw cut-out and non-union. Such complications result in costly re-operations and functional deterioration Citation[2]. To achieve accurate reduction and secure internal fixation, it is important to have a sound knowledge of the techniques and pitfalls associated with fracture reduction maneuvers.
We developed a robot-assisted fracture reduction system integrating navigation and robotics to analyze fracture reduction maneuvers by recording the movement, force, and torque required during anatomical reduction. Subsequently, an effective reduction path is programmed that employs minimum force and torque (). The robotic part of this system (FRAC-Robo) is connected to the boot that holds the ipsilateral foot. It has a motorized traction device with a six-degree-of-freedom (6-DOF) force and torque sensor (). The patient's body and the contralateral foot are placed on a standard fracture table. The system can be used in two operating modes: passive mode and active mode. Passive mode is used when moving the foot by manually guided movement during the initial set-up, with the system in a provisionally reduced position. Active mode is used to move the foot during final fine-tuning of the reduction; FRAC-Robo is moved under remote control using a navigation system that can provide a fracture reduction path based on image guidance.
The specifications of FRAC-Robo are listed in . We defined six degrees of freedom as three rotational and three translation motions; these are shown in . The direction of traction is the Y-axis, and rotation around the Y-axis is defined as the B-axis. The motor units are 1332 N in the Y-axis and 70.8 Nm in the B-axis, while the sensor units are 400 N in the Y-axis and 40 Nm in the B axis. The system has a mechanical overload absorption socket as a safety feature to avoid the application of excessive force to patients; the safety feature was initially set to intervene at 300 N in traction and 25 Nm in rotation. FRAC-Robo is equipped with an emergency stop button that is used if the operator notices an erroneous movement of the system. Force and torque measurements are displayed in real time on the screen of the remote controller. Data for the whole procedure are recorded in a log file.
Table I. FRAC-Robo specifications.
We hypothesized that FRAC-Robo could generate forces sufficient to reduce intertrochanteric fractures of the femur within safe physiologic limits. We therefore conducted the following two sets of studies in volunteers and in patients with intertrochanteric fractures of the femur to evaluate the system's safety features and to assess whether FRAC-Robo could be used clinically for treatment of hip fractures.
Material and methods
In the first experiment, we used the FRAC-Robo system to pull and rotate the limbs of healthy volunteers. We then measured the force and torque applied to the lower extremity to determine the tolerable traction force and rotation torque safety limits for the lower limbs. We measured force and torque using a 6-DOF force and torque sensor connected to the FRAC-Robo boot that holds the ipsilateral foot ().
The subjects were 32 volunteers (16 males and 16 females): average age 23.1 years (range: 19–36 years); average height 167.1 cm (range: 154–193 cm); average weight 60.7 kg (range: 41–90 kg); average thigh girth 44.3 cm (range: 37–61 cm); average body mass index (BMI) 21.6 kg/m2 (range: 16.2–29.4 kg/m2).
The volunteer lay on the operating table and the limb was positioned according to the conventional reduction process. There are two types of intertrochanteric fracture fixation device: the intramedullary fixation type and the dynamic compression screw type. Patients are fixed in adduction using the intramedullary fixation type, whereas patients are fixed in abduction using the dynamic compression screw type. Therefore, the volunteers were positioned on the operating table with their lower limbs fixed in one of two positions: either 0° of flexion and 30° of abduction, or 0° of flexion and 0° of abduction. For both of these positions the contralateral limb was placed with the hip in 30° of abduction. Further traction was applied to the limb with an increment of 5 mm per 5 seconds using the passive mode, and the limb was rotated externally or internally with an increment of 5° per 5 seconds.
We recorded the traction force at both 0 and 30° of abduction, the rotation torque at both 0 and 30° of abduction, and the internal rotation torque using the passive mode after the limb was pulled to 30 mm using the passive mode. Active movement of the robot was stopped at the point when each volunteer felt pain or abnormality in the lower limbs.
In the second experiment, we measured the force of traction and torque of rotation during intertrochanteric fracture reduction using standard manual traction and rotation: measurements were obtained using a force sensor (Nitta Corporation, Osaka, Japan) () positioned between the foot boot and the traction device. This study was approved by the Institutional Review Board (IRB), and informed consent was obtained from each patient.
The subjects of the second study were seven females with intertrochanteric fractures; average age was 79.6 years. There were three stable and two unstable fracture types according to Evans’ classification Citation[3]. Surgery was performed under spinal anesthesia for all patients in this present study.
Each patient was positioned correctly on a standard manual traction table. One of our senior surgeons performed fracture reduction under fluoroscopic guidance following the conventional reduction process. The traction force and rotation torque were recorded digitally while the patient's limb was pulled and then rotated to a position of 30 to 45° of hip abduction. We recorded the traction force versus the traction distance and the rotation torque versus the degree of rotation.
Results
In the first experiment with the volunteers, the average maximum traction force applied to the lower limb was 268.5 N (range: 144.6–432.7 N) for 0° of abduction and 250.7 N (range: 91.7–413.6 N) for 30° of abduction (). The average maximum torque in internal rotation was 6.0 Nm (range: 0.88–14.3 Nm) for 0° of abduction and 5.6 Nm (range: 1.0–9.7 Nm) for 30° of abduction. The average maximum torque in external rotation was 8.8 Nm (range: 0.91–20.4 Nm) for 0° of abduction and 7.6 Nm (range: 1.8–16.2 Nm) for 30° of abduction. The average maximum internal rotation torque after the limb had been pulled to 30 mm was 6.9 Nm (range: 1.5–15.3 Nm) for 0° of abduction and 6.6 Nm (range: 1.6–12.5 Nm) for 30° of abduction. There was no significant difference in force and torque between limbs with 0 and 30° of abduction; however, force and torque were slightly higher for 0° of abduction than for 30° of abduction. The average maximum traction force for 0° of abduction was significantly different from that for 30° (p = 0.0041; Mann-Whitney U test); however, the other measured parameters did not show significant differences for the two measurement angles. No volunteer complained of abnormality or pain in the lower limbs following completion of the experiment.
Table II. Average maximum force and torque applied to the lower limb for the volunteers in the first experiment.
The average maximum traction distance applied to the lower limb was 94.6 mm (range: 60–140 mm) for 0° of abduction and 102.1 mm (range: 75–145 mm) for 30° of abduction. The average maximum internal rotation was 102.7° (range: 40–135°) for 0° of abduction and 101.2° (range: 45–135°) for 30° of abduction. The average maximum external rotation was 121.4° (range: 30–135°) for 0° of abduction and 125.9° (range: 75–140°) for 30° of abduction. The average maximum internal rotation after the limb had been pulled to 30 mm was 102.8° (range: 40–135°) for 0° of abduction and 98.6° (range: 35–135°) for 30° of abduction.
All values for males were significantly larger than those for females (); however, no correlation was found between maximum traction force and the volunteers’ height, weight, girth of thigh, or BMI (paired t-test); maximum traction force for 0° of hip abduction and volunteers’ weights were excluded from analysis (). We found a moderate correlation between traction force at 0 and 30° of abduction and the volunteers’ height and weight. There was no correlation between the maximum torque in rotation and height, weight, girth of thigh, or BMI.
Table III. Comparison of force and torque for female and male volunteers in the first experiment.
Table IV. Correlation between volunteers’ parameters and force and torque.
A typical pattern of the traction force and torque in rotation is displayed in c. Traction force increased proportionally with traction length, whereas torque initially increased slowly as internal rotation was initiated in increments of 5°, after which torque increased sharply. When the limb was rotated internally after being pulled to 30 mm, internal rotation torque increased slowly before increasing sharply.
Figure 6. Traction force and rotation torque for a simulated reduction using volunteers: (a) comparison of traction force versus traction length for 0 and 30° of abduction; (b) comparison of rotation torque versus degree of rotation for 0 and 30° of abduction; and (c) comparison of internal rotation torque versus degree of rotation for the cases of rotation only and for both traction and rotation.
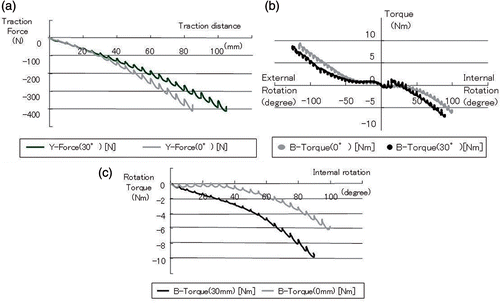
The results for all patients in the second experiment are listed in and . The average maximum traction force applied to the lower limb was 215.9 N (range: 146.3–294.9 N), and the average maximum torque was 3.2 Nm (range: 1.6–4.4 Nm) in internal rotation. The average force required to be applied to the lower limb to maintain reduction was 163.8 N (range: 106.7–274.0 N), and the average torque required to maintain reduction was 2.4 Nm (range: 1.2–4.8 Nm) in internal rotation. In cases with stable fractures, the average force required to maintain reduction was 136.7 N, and the average torque required to maintain reduction was 3.8 Nm in internal rotation. In cases with unstable fractures, the average force required to maintain traction was 229.2 N, and the average torque required to maintain traction was 1.5 Nm in internal rotation.
Table V. Force and torque measurements for reduction and for maintaining reduction for all patients in the second experiment.
Table VI. Average maximum traction force, force to maintain traction, and internal rotation torque for the patients in the second experiment.
shows a typical pattern of traction force and torque in rotation during reduction for a 70-year-old female with a stable intertrochanteric fracture. The traction force increased sharply as the limb was pulled, before reaching a plateau (a), whereas the torque increased slightly during rotation of the limb but returned to the previous level while the position was maintained (b). Traction force and torque values for limb rotation in the remaining patients were similar to those in .
Discussion
There has been recent progress in the field of navigation systems for computer-assisted fracture reduction, such as those used for guidewire insertion Citation[4], screw positioning, and CT-based navigation, e.g., in the reduction of pelvic ring fractures Citation[5]. However, we found only one report in the literature that describes robotic surgery for femoral shaft fractures Citation[6]. This previously developed robot was equipped with a two-fingered gripper that could grip the AO fixator clamp. The femoral shaft fracture in vitro was stabilized with a standard AO fixator, and the reduction maneuver was performed using a two-fingered gripper. In contrast, our robot has a motorized traction device connected to the boot that holds the ipsilateral foot, and a 6-DOF force and torque sensor. The reduction maneuver was performed to apply traction and rotate the limb as in manual reduction.
The robotic part of this system (FRAC-Robo) has a motorized traction device, but it measures the force, torque, translation length, and degree of rotation during reduction. FRAC-Robo also enables analysis of manual reduction.
Before commencing clinical use of the FRAC-Robo system, it is necessary to estimate whether the FRAC-Robo motor and sensor unit settings are able to generate sufficient force and torque for fracture reduction.
In the second experiment, the average maximum traction force for intertrochanteric fracture was 215.9 N and the average maximum internal rotation torque was 3.2 Nm. The average maximum traction force required to maintain reduction of the fracture was 163.8 N with a maximum internal rotation torque of 2.4 Nm. The traction force required to maintain reduction was less than that required to achieve reduction, and the rotation torque. The rotation torque required to maintain reduction was smaller than the torque required for reduction. Interestingly, we found no significant differences between the traction forces for the stable and unstable fracture types; however, we observed a tendency to use larger traction forces to maintain reduction in unstable fractures as compared to stable fractures. Because the motor unit can generate up to 1332 N of traction force and 70.8 Nm of rotational torque, and the sensor unit can bear up to 400 N in the Y-axis and 40 Nm in the B-axis, the average maximum traction force and rotation torque for reduction were far less than the maximum range of the sensor unit. Although the patient study population was small and all patients were female, the motor and sensor units of the FRAC-Robo system appear to have a greater range of force and torque than that necessary for the reduction of intertrochanteric fractures. According to Gosling et al., the maximum resulting force during reduction of the femoral shaft fracture is 411 N, and the maximum resulting torque is 74 Nm Citation[7]. FRAC-Robo can be used for the reduction of femoral shaft fractures as well as intertrochanteric fractures; however, we need to consider a higher sensor unit setting when reducing femoral shaft fractures.
It is also necessary to evaluate the safety feature of FRAC-Robo, which involves a mechanical overload absorption socket at 300 N in traction and 25 Nm in rotation. Fracture reduction requires force and torque to be applied to soft and hard tissues; for example, bone, muscle, tendon, joint capsule and ligaments, skin and fat. To determine the threshold values of force and torque at which the mechanical overload absorption socket intervenes as a safety feature, we measured the force and torque applied to the lower limb during simulated fracture reduction maneuvers in healthy volunteers. The average forces at which the volunteers felt pain or abnormality in the lower limbs were 268.5 N and 250.7 N at 0 and 30° of abduction, respectively. The average torques at which the volunteers felt pain or abnormality in the lower limbs were 6.0 Nm and 5.6 Nm at 0 and 30° of abduction, respectively. For safety, we regarded excessive force to be 300 N and excessive torque to be 25 Nm. We consider that our excessive traction-force setting was appropriate; however, the excessive rotation torque value needs to be re-evaluated, as the maximum value was set too high.
In the current study, the examination was performed using conscious rather than anesthetized volunteers. Compared to the force and torque required for reduction under anesthesia, it might be necessary to apply greater force and torque to the lower limb to pull or rotate the limb in conscious subjects due to the higher tonus of muscles; therefore, we consider that FRAC-Robo can be used safely for fracture reduction without harm to the lower limbs, even when approaching the limits of maximum traction force and maximum rotation torque.
The average traction force and rotation torque were larger for healthy males than for healthy females. Traction force tended to show correlations with both height and weight, but neither rotation torque nor traction force showed a correlation with structural parameters. Differences in soft-tissue elasticity may be a key factor in this regard. In the experiment using volunteers, the traction force was proportional to the distance that the leg was pulled, whereas the rotation torque increased sharply during the reduction process. In longitudinal traction, the structure of the lower limbs may be free from excess play. The greater the longitudinal traction length, the greater the proportional increase in traction force. During rotation, the hip, knee, and ankle joints are initially rotated. As there may be excess play in the joints, torque does not increase at first. After the maximum ranges of hip, knee, and ankle motion are reached, excess play is no longer present and rotation torque increases sharply past the maximum range of joint motion. When the limb is rotated internally after having being pulled in longitudinal traction, the torque increases incrementally because the joint is free from excess play.
Conclusion
FRAC-Robo can generate sufficient force and torque to reduce intertrochanteric femoral fractures. The fail-safe system consists of an emergency stop button and a mechanical overload absorption socket that intervenes at 300 N in traction and 25 Nm in rotation, and seems to be appropriate for clinical use. In a future study, we intend to analyze fracture reduction maneuvers using FRAC-Robo by recording the required movement, force, and torque during anatomical reduction.
References
- Miller CW. Survival and ambulation following hip fracture. J Bone Joint Surg Am 1978; 60: 930–934
- Hesse B, Gachter A. Complications following the treatment of trochanteric fractures with the gamma nail. Arch Orthop Trauma Surg 2004; 124: 692–698
- Evans E. The treatment of trochanteric fractures of the femur. J Bone Joint Surg Br 1949; 31B: 190–203
- Mayman D, Vasarhelyi EM, Long W, Ellis RE, Rudan J. Pichora DR. Computer-assisted guidewire insertion for hip fracture fixation. J Orthop Trauma 2005; 19: 610–615
- Hufner T, Pohlemann T, Tarte S, Gansslen A, Geerling J, Bazak N, Citak M, Nolte LP, Krettek C. Computer-assisted fracture reduction of pelvic ring fracture: An in vitro study: Clin Orthop Relat Res 2002; 339: 231–239
- Füchtmeier B, Egersdoerfer S, Mai R, Hente R, Dragoi D, Monkman G. Nerlich M. Reduction of femoral shaft fractures in vitro by a new developed reduction robot system “RepoRobo”. Injury, Int J Care Injured 2004; 35: S-A113–S-A119
- Gösling T, Westphal R, Faülstich J, Sommer K, Wahl F, Krettek C. Hufner T. Forces and torques during fracture reduction: Intraoperative measurements in the femur. J Orthop Res 2006; 24(3)333–338