Abstract
Objective: To determine whether robotic stereotactic radiotherapy of 70–75 Gy delivered in five fractions results in an improved therapeutic ratio, compared with three fractions, in the treatment of peripheral non-small-cell lung cancer (NSCLC), in which case doses of up to 85 Gy in five fractions may be feasible.
Materials and Methods: Between December 2006 and May 2010, 20 patients (9 female, 11 male, aged 65 to 88) were treated using the CyberKnife® Robotic Radiosurgery System for NSCLC with doses ranging from 67 Gy to 75 Gy based on location, histopathological type, grade of histopathological differentiation, tumor diameter/volume, and normal tissue constraints, with the doses being delivered in five fractions over 5 to 8 days. Tumor diameters ranged from 1.5 cm to 3.4 cm (median: 2.5 cm). Patients with Stage I to IV NSCLC were treated, and the results and observations were analyzed for clinical characteristics and outcomes including toxicity. All patients, except one who had refused surgery, had co-morbid conditions that precluded a lobectomy.
Results: Twenty patients were followed every three months by positron emission tomography/computed tomography (PET/CT). Mean follow-up was 23 months (range: four to 58 months). Local control was achieved in all treated tumors. Three patients expired, and three developed new regional metastases, none of which was within the planning target volume (PTV). The remainder of the patients demonstrated no evidence of recurrence or continued growth detectable by PET/CT. There was no toxicity above Grade 1.
Conclusions: It is feasible to treat peripheral NSCLC with individualized maximal tolerable doses ranging from 67 Gy to 75 Gy in five fractions chosen on the basis of location, histopathological type, grade of histopathological differentiation, tumor diameter/volume, and normal tissue constraints.
Introduction
Conventional radiotherapy for non-small-cell lung cancer (NSCLC) is considered the standard of care for patients who are not surgical candidates. However, these treatment schedules give rise to frequent local failure in the majority of cases, with reported five-year survival rates ranging from 10 to 30% Citation[1–5]. Several studies have reported a benefit to higher doses, suggesting that there is a dose-response relationship with respect to both survival and local control in these patients Citation[3], Citation[5–8]. A review of 156 medically inoperable Stage I NSCLC patients at Duke University between 1980 and 1995 demonstrated a five-year cause-specific survival of 32% with radiotherapy alone. Higher radiotherapy doses correlated significantly with achieving improved survival and local control Citation[8].
Conventional wide-field radiotherapy for NSCLC encompassed the primary tumor and regional lymphatics in the ipsilateral hilum and mediastinum. This treatment approach was based on the risk of occult nodal involvement (an incidence of up to 25%, based on surgical series), indicating better control with more extensive resections Citation[9]. However, large radiotherapy fields are poorly tolerated by patients with limited pulmonary reserve Citation[10], and more recent retrospective observations show similar survival results with fields limited to the primary tumor alone Citation[11–14].
Blomgren et al. at the Karolinska Hospital in Stockholm, Sweden, developed a stereotactic body frame and began treatment with this device in 1992. The results obtained for patients treated in the extracranial stereotactic frame for metastases in the chest and abdomen have been reported by the Swedish group. Treatment failure within the planning treatment volume (PTV) was observed in 8 of 26 patients treated with doses of up to three fractions of 20 Gy each, totaling 60 Gy Citation[15], Citation[16]. This technique, known as stereotactic body radiotherapy (SBRT), is a method of escalating the intensity of radiation to extracranial tumors. SBRT requires the ability to precisely determine the location and extent of motion of tumors in order to be able to develop complex 3D treatment plans in non-equilibrium conditions and deliver the high dose with great geometric precision, usually with 7–10 arcs or beams.
The CyberKnife® Robotic Radiosurgery System (Accuray, Incorporated, Sunnyvale, CA) is a significantly different form of SBRT, in that the linear accelerator (LINAC) is highly mobile and the system has few restrictions on range of motion and beam direction. The CyberKnife System consists of a compact 6-MV LINAC manipulated by a robot capable of moving in six degrees of freedom, two orthogonally placed X-ray units for radiographic three-dimensional target localization, and an optic-radiographic motion monitoring system for real-time kV tumor tracking, replacing the stereotactic body frame with image-guided localization and targeting. With its Synchrony® Respiratory Tracking System, it has been adapted to track tumor motion secondary to respiration by moving the LINAC in concert with the patient's breathing, and is the only commercial system with this capability. This new technology uses low-dose beams in the range of hundreds for the delivery of precise, high doses of ablative radiation to the tumor, with minimal irradiation of adjacent normal tissue and regional lymph nodes. Beams may be delivered isocentrically or non-isocentrically (). Radio-opaque fiducial markers are implanted in or around the tumor and light-emitting diodes (LEDs) are placed on the patient's chest as surrogates to model tumor motion during beam delivery.
Figure 1. Dose volume comparison: seven-beam configuration versus the CyberKnife. V(CK) is the volume receiving a given percentage dose level for the CyberKnife plan and V is the volume receiving this level with either the seven-beam configuration (blue curve) or the single-arc plan (red). The isodose line range for our patients was 60 to 84%. V/V(CK) is seen to be ∼1.00 for the dose level 70–80%. The dose volume for 7B/CK includes nearly five times more adjacent tissue than CK. (From reference Citation[39]).
![Figure 1. Dose volume comparison: seven-beam configuration versus the CyberKnife. V(CK) is the volume receiving a given percentage dose level for the CyberKnife plan and V is the volume receiving this level with either the seven-beam configuration (blue curve) or the single-arc plan (red). The isodose line range for our patients was 60 to 84%. V/V(CK) is seen to be ∼1.00 for the dose level 70–80%. The dose volume for 7B/CK includes nearly five times more adjacent tissue than CK. (From reference Citation[39]).](/cms/asset/270df642-58d0-4660-be2e-69a40e21d309/icsu_a_537483_f0001_b.gif)
Here we present the results of a higher dose using CyberKnife hypofractionation delivered in five fractions, designed to test the feasibility of increasing the total dose up to 75 Gy, taking advantage of the high α/β ratio of NSCLC (which could be as high as 50 Gy), which should result in an increase in cell kill by 10%, reduce late complications by more than 10%, and thereby improve the therapeutic ratio, local control, and survival Citation[17], Citation[18].
Materials and methods
Patient selection
Patients were deemed ineligible for surgery if they lacked adequate pulmonary reserve. Eight patients were determined as Stage I, four as Stage II, three as Stage III, and five as Stage IV. Patients receiving systemic chemotherapy were included. All patients were referred for medical oncological consultation. They were all evaluated by a radiation oncologist and a thoracic surgeon, and had biopsy-proven and clinically staged NSCLC. The assignment of tumor diameter (T) was based on the planning computed tomography (CT) images, using lung windows for sizing. Patients with T1, T2 and T3 tumors (up to 4 cm maximum diameter and primary chest-wall tumors only) were included. No patients with tumors of any T-stage in the zone of the proximal bronchial tree were considered as candidates. Patients with T3 tumors based on mediastinal invasion or within 2 cm of carinal invasion were considered ineligible ().
Table I. Patient data. The column headers represent the following: Pt #: patient number;1st Rx: date of first treatment; Sex; Age; Loc: location of tumor; Dia: tumor diameter in centimeters; dose: total dose in Gy; Fx: number of fractions; V15: cc of lung irradiated with 15 Gy, total volume of both lungs minus the planning treatment volume; IDL: isodose level use to treat tumor; BED20: biological equivalent dose delivered if α/β is 20 Gy; Fiducials: number of fiducials placed in tumor; Beams: total number of beams used to deliver dose; Paths: number of paths used to deliver dose; P/M: whether primary and/or metastatic tumor treated; Cell: cell type (adeno - adenocarcinoma, NSCLC - non-small cell lung cancer, BAC - bronchial alveolar cell carcinoma, Neuro end – neuro-endocrine carcinoma, Squamous – squamous cell carcinoma); Results: results of irradiation (LC – local control, DM – distant metastasis, RR – regional recurrence, Bx – biopsy); Stage: stage of NSCLC at diagnosis and treatment. Tumor effects are the same, but late complications are much less common with 5 fractions.
Treatment dose
We individualized the treatment dose with escalating doses based on the volume, location, histology, and nuclear grade of the tumor. Patients with tumors less than 2 cm in diameter received five fractions of 15 Gy; those with tumors 2–3 cm in diameter received five fractions of 16 Gy; and patients with tumors 3–4 cm in diameter received five fractions of 17 Gy. In the case of Grade 1 tumors, the planning target volume (PTV) included the clinical target volume (CTV) and a 2-mm margin for uncertainty, and the CTV included the gross tumor volume (GTV) and a 6-mm margin to cover microscopic extension. Grade 2 tumors were treated using a PTV including the CTV and a 2-mm margin for uncertainty, and the CTV encompassed the GTV and a 5-mm margin to cover microscopic extension. For Grade 3 tumors, a 2-mm margin was added to the GTV to cover microscopic extension, together with a further 2 mm for uncertainty, to obtain the PTV. Squamous cell carcinomas were treated with margins of 4 mm and 2 mm to cover microscopic extension and uncertainty, respectively. The CyberKnife radiosurgical technique for lung tumors has been described in several previous reports from our group Citation[19–23]. The total system accuracy of the device and the respiratory motion tracking in a phantom has been reported as 1 mm in two recent articles Citation[27], Citation[28]. Normal tissue constraints comprised a mean total lung dose of less than 19 Gy, and the spinal cord dose being limited to 6 Gy per fraction and not exceeding 18 Gy in total over the five fractions. In the series, the lung V20 was kept below 19 Gy for both lungs minus the PTV, and the lung V15 ranged from 152 cc to 595 cc. The MultiPlan® (Accuray, Inc.) ray tracing algorithm was used for heterogeneity correction.
Tracking
An inverse planning algorithm was used to maximize dose conformality. Three tumors at least 1.5 cm in diameter, and whose outlines were clearly distinguishable on X-ray images, were treated fiducial-free using the Xsight® Lung Tracking System (Accuray, Inc.). Activated at different levels of the breathing cycle, the X-ray sources in the treatment room take orthogonal radiographs of the fiducial marker in the target. The detected target position is correlated with the respiratory cycle using the LED sources on the patient's chest. Eight to 10 images are taken to generate a model for calculating the dependence of breathing cycle and target position. The model is used by the robotic system to anticipate the target position and to synchronize the tumor movement in real time. During the treatment, repeat images are acquired to verify the position, and, if necessary, to update the correlation model. Thus the robotic system moves with respiration during beam application. An image may be taken before each beam or every 2–3 beams, depending on the stability of the tumor motion.
Follow-up and assessment of response
Patients were followed every three months using PET/CT imaging for the first two years, then every four to six months for the remainder of a total five-year follow-up period. Our initial assessment was based on Response Evaluation Criteria in Solid Tumors (RECIST). Any response that was readily assessed using RECIST rules as complete response (CR), partial response (PR), or stable (S) was considered as local control (LC), unless progressive disease (PD) was detected on the subsequent imaging study.
In lung tumor patients treated with high ablative doses, fibrotic or ground glass changes matching the high-dose isodose distribution were considered to represent pneumonitis or treatment effects as opposed to disease progression (), especially when PET imaging showed no corresponding hypermetabolism. However, the frequency of radiographic changes resulting from inflammation and fibrosis in the surrounding parenchyma complicated the local control evaluation. In those cases where the local control evaluation was equivocal, we compared the post-treatment images to the planning FDG-PET/CT scans, and compared the overlying treatment dose distribution. This was correlated in nearly all instances with stable or gradually improving characteristic changes in serial studies. If a progressive nodular enlargement, reappearing or enlarging opacities after shrinkage or disappearance, or increasing standardized uptake value (SUV) on FDG-PET within the lesion (PTV) raised suspicions of tumor recurrence, this prompted a pathological exploration.
Figures 2. In lung tumor patients treated by high ablative doses, fibrotic or ground glass changes matching the high-dose isodose distribution were considered to represent pneumonitis or treatment effects as opposed to disease progression. This image series shows gradually improving characteristic changes following 15 Gy × 5 fractions = 75 Gy10: (1) Planning CT scan, April 2009; (2) CyberKnife ablation at 90 days; (3) August 2009 follow-up CT scan; (4) November 2009 follow-up; and (5) February 2010 follow-up.
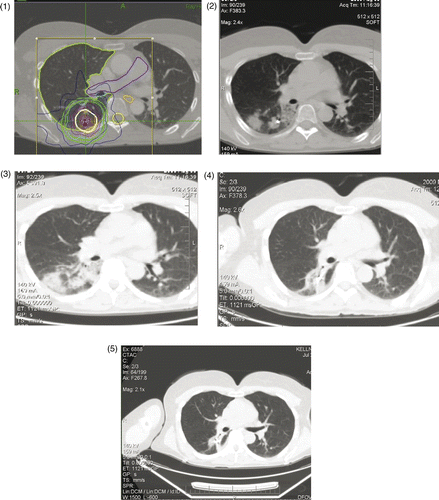
A regional recurrence is a new lesion within the same lobe, the hilar lymph nodes, or both. Regional recurrence was not considered to be due to inadequate irradiation, but rather related to occult disease.
Toxicity was scored according to the National Cancer Institute Common Terminology Criteria for Adverse Events.
Results
Twenty patients were treated by escalating dosage. Three patients received 67 to 67.5 Gy in five fractions, 13 received 70 Gy in five fractions, and four patients received 75 Gy in five fractions. All patients were followed every three months by PET/CT scans, with a median follow-up of 12 months. All patients demonstrated local control of their primary tumors. Three patients expired from intercurrent disease: two had Stage IV disease, and one, who had complete resolution of the primary tumor, died as a consequence of a distant metastasis to the CNS five months post-treatment of the primary. The second patient with Stage IV disease also died of a CNS metastasis. The third death occurred as a result of congestive heart failure three months post-therapy. This patient had Stage IIB disease and maintained local control until the end. Three patients developed regional recurrences, none within the PTV. There was no toxicity greater than Grade 1. There was some chest wall discomfort in two patients, which was treated by non-steroidal therapy. Two patients, one of whom had refused surgery, had questionable responses on follow-up PET/CT scans at 3 months, and were therefore biopsied. In both cases, the fine-needle aspiration biopsies were negative for malignant cells. The remainder of the cases showed no evidence of recurrence or continued growth detectable by PET/CT scan.
Discussion
Seven years ago, we treated our first NSCLC with CyberKnife using the RTOG 0236 recommendations of 20 Gy × 3 fractions. At first we added a 3-mm expansion to the GTV for uncertainty. Later we expanded this to 5 mm to cover uncertainty and microscopic extension. In following these patients we found that, on occasion, there was recurrence within the PTV or immediately next to it. It was concluded that these recurrences were secondary to microscopic extension and/or a need for higher doses. Two years ago, we use 75 Gy in 5 fractions with an 8-mm expansion to cover microscopic extension and uncertainty. Since using a higher dose with the increased expansion we have observed no recurrence within the PTV or next to it with 100% local control. The series is small and we have a mean of 22 months follow-up. The following discussion describes how we arrived at this dosing.
Although the LCSG study is the only prospective randomized trial addressing the question of whether lobectomy is better than wedge or segmental resection, no other modern study has been able to confirm the superiority of lobectomy over wedge resection. Other studies observing Stage I NSCLC have shown no difference in survival time between patients undergoing wedge resection and those undergoing lobectomy. A meta-analysis of these studies and others has also reached the same conclusion Citation[24].
Lobectomy, the preferred procedure, results in significant loss of functional pulmonary reserve and is associated with operative morbidity and mortality. Dr. H. I. Pass (Department of Cardiothoracic Surgery, New York University) concluded that SBRT was a “good option”, and stated that “we are moving towards using SBRT in patients who otherwise have resectable disease” Citation[25].
Presently, there is a shift in the management of early NSCLC toward individualized local treatment. The question of performing lobectomy on every patient is evolving as a result of recognition of the variation among lung nodules. The question becomes whether we can perform fewer lobectomies, thereby preserving pulmonary function.
Grills et al. Citation[26], Citation[27] reported a trend toward reduced local recurrence with SBRT compared to wedge resection (4% following SBRT versus 20% with wedge resection, P = 0.07). No statistical difference was identified between outcomes with SBRT and wedge resection at 30 months, but overall survival was better with wedge resection (87% with wedge resection versus 72% with SBRT, P = 0.01). However, cause-specific survival, eliminating non-cancer deaths, was identical (94% with wedge resection versus 93% with SBRT, P = 0.53). There is no technical hindrance to obtaining adequate “margin” on all sides of the treated tumor with the photon-based CyberKnife using high target doses, and the results are comparable to lobectomy control rates. The CyberKnife technique effectively performs a “non-invasive wedge resection” via focal ablation of pulmonary tissue and tumor, preserving maximum adjacent pulmonary function.
Hayman and co-workers at the University of Michigan Citation[28] have reported on 104 patients with Stage I-III NSCLC treated by 3D-CRT with dose escalation as high as 102.9 Gy in 2-Gy fractions over 10 weeks. Of note was the fact that, despite the dose intensification, 53 patients had disease progression, with 52% failing distantly; 8% failing both distantly and within the PTV; 2% failing in a distant site, the PTV, and a nodal region outside the PTV; and 35% failing within the PTV alone. The high rate of failure within the PTV offers justification for maintaining high doses even in Stage I patients. The likely reason for the high failure rate of this study was the fact that the treatment was spread over an extended period of time, reducing the biologically equivalent dose absorbed by the tumor and giving the malignant cells a chance to repair sub-lethal damage.
Higher dose and fractionation
Higher dose has been an ongoing focus of national cooperative clinical trials and institutional studies in an effort to improve on locoregional control rates and ultimately on survival outcomes, while at the same time maintaining acceptable levels of morbidity.
Chi et al. have analyzed the patterns of failure of SBRT in the treatment of early NSCLC. Local control ranged from 80% to 100% in most studies with adequate biological effective dose (BED) Citation[29]. Recurrences were associated with increasing tumor size. Studies by Onimaru et al. Citation[30] and Koto et al. Citation[31] have suggested significantly increased local control and overall survival rates, in other words, a steep dose-response gradient, in larger tumors. These results demonstrate treatment regimens in patients with T1 tumors, while failing to provide adequate control of T2 tumors in the lower-dose arm. Timmerman et al. Citation[32] and McGarry et al. Citation[33] at Indiana University performed a phase I dose-escalation toxicity study in 47 patients with medically inoperable lung cancer. The starting dose was 24 Gy in 3 fractions, and a total of seven subsequent dose levels were tested. The maximum tolerated dose was never reached for T1 tumors and T2 tumors of less than 5 cm, despite reaching 60 to 66 Gy in 3 fractions. For the largest tumors (5–7 cm), dose was escalated to 72 Gy in 3 fractions, which proved to be too toxic. Dose-limiting toxicity in that subset included bronchial injury, pneumonia, and pericardial effusion. On completion of the phase I study to find a clearly potent dose for SBRT, the group embarked on a 70-patient phase II study in the same population. The phase II study used a total dose of 60 Gy in 3 fractions for the small tumors and 66 Gy in 3 fractions for the large tumors (35 patients for each group). The actuarial 2-year local control for this potent dose regimen is 95%, and isolated hilar or mediastinal nodal relapse is extremely rare despite clinical staging. The overall 2-year survival for this frail population is poor at 56%, with most of the deaths related to co-morbid illness rather than disease progression or toxicity. The protocol placed no time limits on scoring treatment-related toxicity. Although fewer than 20% of patients have experienced high-grade toxicity, interim analysis showed that severe toxicity (grades 3 to 5) was significantly more likely in patients treated for tumors in the regions around the proximal bronchial tree or central chest region.
Dose escalation studies Citation[12–14], Citation[34] have revealed a clear dose-response curve for 30-month survival. It appears the efficacy of radiosurgery in killing tumor cells is proportional not only to the total dose delivered in the course of the treatment, but also to the size of the radiosurgical fraction delivered daily, the tumor volume, and the location of the tumor. Clinical studies indicate that local control of primary lung tumors treated by radiotherapy is proportional to the total Biologically Effective Dose delivered, allowing for the number and size of fractions Citation[17]. Tumor cells may be able to repair a proportion of radiation injury after each fraction, depending on the slope of the survival curve, and this effect may be less marked when higher doses and fewer consecutive fractions are used. Such high doses may be needed to achieve local tumor control based on the hypothesis that, if radio-resistant hypoxic cells are present, or some cells are in a resistant phase of the cell cycle, then doses two-and-a-half to three times as great as those required to kill well-oxygenated cells are required to kill this cell population. A total dose of 75 Gy to 85 Gy would then offer a chance of reducing the tumor burden for a 1- to 10 -g tumor to one viable cell on average Citation[17], Citation[18], Citation[33]. If reoxygenation is incomplete, and only 1% of tumor cells remain hypoxic, then doses many orders of magnitude higher would be required to kill these cells. Furthermore, Fowler argues that tumor cell kill varies between 16 and 27 logs to base 10, but reduces to 8–13 logs when an α/β of 20 Gy, instead of 10 Gy, is assumed Citation[35] () for dosing schedules ranging from four fractions of 12 Gy to three fractions of 23 Gy. Although high cell killing rates were predicted for three fractions of 15 to 16 Gy within less than two weeks, local recurrences have been reported.
Table II. Comparison of 5-fraction and 3-fraction SBRT for lung tumors α/β 20 Gy.
The explanation for the higher end of this scale is the possible presence of hypoxic or otherwise extraordinarily resistant cells. Possible tolerability of such large doses by “parallel type organs” has been shown to be theoretically possible, provided that suitably small volumes are irradiated, with a rapid fall-off of dose outside the PTV, and a mean dose to both lungs, excluding the PTV, of less than 19 Gy normalized total dose (NTD). Thus, smaller tumors of stage IA are more likely to be controlled by doses of three fractions of 18.5 Gy and three fractions of 20 Gy (34, 35, 38).
Escalation of the dose from three fractions of 20 Gy to higher doses can be done more efficiently in five fractions than in three. This is because damage to normal tissue at risk rises more rapidly than damage to tumor cells for larger doses per fraction, so the five smaller fractions produce the same effect on tumors with less damage to normal tissue. For example, consider an equal tumor dose of 109 Gy NTD given with three fractions of 20 Gy or five fractions of 14 Gy. The three fractions deliver to late-damaging normal tissue the much higher NTD of 276 Gy, compared to only 238 Gy in the case of five fractions (a 15% saving). All NTDs stated above correspond to the effect that would be caused when delivered in 2-Gy fractions. At an escalated dose level, an equivalent tumor dose of 143 Gy would be caused by three fractions of 23 Gy or five fractions of 17 Gy, but to normal tissues (α/β = 3 Gy) the three fractions would deliver 390 Gy NTD while the five-fraction regimen would give only 304 Gy NTD (a saving of 12% in NTD). That is why we prefer to use five-fraction schedules of 15 Gy, 16 Gy, or 17 Gy rather than three fractions of 20, 22, or 23 Gy for gradual dose escalation. Stepping up with five fractions, from 15 Gy to 16 Gy, gives only an apparently modest 10% increase in dose to normal tissues, but a substantial 20% increase in tumor NTD ().
The therapeutic ratio of tumor NTD/normal tissue NTD is almost exactly reversed for three fractions versus five fractions. Three fractions of 23 Gy would result in a therapeutic ratio of 20/30 dose units, which is undesirably less than unity. Five fractions of 16 Gy would produce a healthy 20/10 therapeutic ratio, and five fractions of 17 Gy would produce a 31/23 therapeutic ratio, both being greater than unity. Although survival is influenced by local control with all cell types of NSCLC, and a progressive increase in survival can be demonstrated with increasing biological doses, survival is not so direct a measure of efficacy of irradiation as tumor control: as seen in this series, we obtained 100% local control with 70–75 Gy/5F in tumors from 1.5 to 3.5 cm in diameter with no significant toxicity.
Conclusions
CyberKnife focal irradiation allows higher target doses while limiting irradiation to adjacent normal tissues as an outpatient noninvasive therapy. Resultant benefits include rapid recovery, minimal discomfort, and cost-effectiveness with extremely high rates of local control. CyberKnife is a non-surgical alternative that is superior to conventionally fractionated radiotherapy, and slandered SBRT (7–12 beams) with results that may be approaching those obtained with lobectomy. The main pattern of failure is distant metastasis. Individualized maximal tolerable dose irradiation based on the location, volume, histology, and grade of tumor and normal tissue constraints is feasible. Additional trials are needed to better evaluate the efficacy of this form of therapy.
Declaration of interest: James G. Schwade is owner and Chief Executive Officer of Miami CyberKnife Center. There are no other potential conflicts of interest known to the authors.
References
- Armstrong JG, Minsky BD. Radiation therapy for medically inoperable stage I and II non-small cell lung cancer. Cancer Treat Rev 1989; 16: 247–255
- Coy P, Kennelly GM. The role of curative radiotherapy in the treatment of lung cancer. Cancer 1980; 45: 698–702
- Dosoretz DE, Katin MJ, Blitzer PH, Rubenstein JH, Salenius S, Rashid M, Dosani RA, Mestas G, Siegel AD, Chadha TT, et al. Radiation therapy in the management of medically inoperable carcinoma of the lung: Results and implications for future treatment strategies. Int J Radiat Oncol Biol Phys 1992; 24: 3–9
- Haffty BG, Goldberg NB, Gerstley J, Fischer DB, Peschel RE. Results of radical radiation therapy in clinical stage I, technically operable non-small cell lung cancer. Int J Radiat Oncol Biol Phys 1988; 15: 69–73
- Kaskowitz L, Graham MV, Emami B, Halverson KJ, Rush C. Radiation therapy alone for stage I non-small cell lung cancer. Int J Radiat Oncol Biol Phys 1993; 27: 517–523
- Dosoretz DE, Galmarini D, Rubenstein JH, Katin MJ, Blitzer PH, Salenius SA, Dosani RA, Rashid M, Mestas G, Hannan SE, et al. Local control in medically inoperable lung cancer: An analysis of its importance in outcome and factors determining the probability of tumor eradication. Int J Radiat Oncol Biol Phys 1993; 27: 507–516
- Dosoretz DE, Katin MJ, Blitzer PH, Rubenstein JH, Galmarini DH, Garton GR, Salenius SA. Medically inoperable lung carcinoma: The role of radiation therapy. Semin Radiat Oncol 1996; 6: 98–104
- Sibley GS, Jamieson TA, Marks LB, Anscher MS, Prosnitz LR. Radiotherapy alone for medically inoperable stage I non-small-cell lung cancer: The Duke experience. Int J Radiat Oncol Biol Phys 1998; 40: 149–154
- Ginsberg RJ, Rubinstein LV. Randomized trial of lobectomy versus limited resection for T1 N0 non-small cell lung cancer. Lung Cancer Study Group. Ann Thorac Surg 1995; 60: 615–622, discussion 622–613
- Curran WJ, Jr, Moldofsky PJ, Solin LJ. Analysis of the influence of elective nodal irradiation on postirradiation pulmonary function. Cancer 1990; 65: 2488–2493
- Krol AD, Aussems P, Noordijk EM, Hermans J, Leer JW. Local irradiation alone for peripheral stage I lung cancer: Could we omit the elective regional nodal irradiation?. Int J Radiat Oncol Biol Phys 1996; 34: 297–302
- Robertson JM, Ten Haken RK, Hazuka MB, Turrisi AT, Martel MK, Pu AT, Littles JF, Martinez FJ, Francis IR, Quint LE, et al. Dose escalation for non-small cell lung cancer using conformal radiation therapy. Int J Radiat Oncol Biol Phys 1997; 37: 1079–1085
- Slotman BJ, Antonisse IE, Njo KH. Limited field irradiation in early stage (T1-2N0) non-small cell lung cancer. Radiother Oncol 1996; 41: 41–44
- Williams TE, Thomas CR, Jr, Turrisi AT, 3rd. Counterpoint: Better radiation treatment of non-small cell lung cancer using new techniques without elective nodal irradiation. Semin Radiat Oncol 2000; 10: 315–323
- Blomgren H, Lax I, Näslund I, Svanström R. Stereotactic high dose fraction radiation therapy of extracranial tumors using an accelerator. Clinical experience of the first thirty-one patients. Acta Oncol 1995; 34: 861–870
- Lax I, Blomgren H, Näslund I, Svanström R. Stereotactic radiotherapy of malignancies in the abdomen. Methodological aspects. Acta Oncol 1994; 33: 677–683
- Fowler JF. Comment on “Magical protons?” editorial by Dr. Michael Goitein (Int J Radiat Oncol Biol Phys 2008;70:654–656). Int J Radiat Oncol Biol Phys 2008;72:1270–1271.
- Fowler JF. Sensitivity analysis of parameters in linear-quadratic radiobiologic modeling. Int J Radiat Oncol Biol Phys 2009; 73: 1532–1537
- Brown WT, Wu X, Amendola B, Perman M, Han H, Fayad F, García S, Lewin A, Abitbol A, de la Zerda A, et al. Treatment of early non-small cell lung cancer, stage IA, by image-guided robotic stereotactic radioablation – CyberKnife. Cancer J 2007; 13: 87–94
- Brown WT, Wu X, Fayad F, Fowler JF, Amendola BE, García S, Han H, de la Zerda A, Bossart E, Huang Z, et al. CyberKnife radiosurgery for stage I lung cancer: Results at 36 months. Clin Lung Cancer 2007; 8: 488–492
- Brown WT, Wu X, Fayad F, Fowler JF, García S, Monterroso MI, de la Zerda A, Schwade JG. Application of robotic stereotactic radiotherapy to peripheral stage I non-small cell lung cancer with curative intent. Clin Oncol (R Coll Radiol) 2009; 21: 623–631
- Brown WT, Wu X, Fowler JF, García S, Fayad F, Amendola BE, de la Zerda A, Schwade JG. Lung metastases treated by CyberKnife image-guided robotic stereotactic radiosurgery at 41 months. South Med J 2008; 101: 376–382
- Brown WT, Wu X, Wen BC, Fowler JF, Fayad F, Amendola BE, García S, de la Zerda A, Huang Z, Schwade JG. Early results of CyberKnife image-guided robotic stereotactic radiosurgery for treatment of lung tumors. Comput Aided Surg 2007; 12: 253–261
- Nakamura H, Kawasaki N, Taguchi M, Kabasawa K. Survival following lobectomy vs limited resection for stage I lung cancer: A meta-analysis. Br J Cancer 2005; 92: 1033–1037
- Love N. Conversations with oncology investigators: Bridging the gap between research and patient care. Lung Cancer Update; 2009.
- Grills IS, Fitch DL, Goldstein NS, Yan D, Chmielewski GW, Welsh RJ, Kestin LL. Clinicopathologic analysis of microscopic extension in lung adenocarcinoma: Defining clinical target volume for radiotherapy. Int J Radiat Oncol Biol Phys 2007; 69: 334–341
- Grills IS, Mangona VS, Welsh R, Chmielewski G, McInerney E, Martin S, Wloch J, Ye H, Kestin LL. Outcomes after stereotactic lung radiotherapy or wedge resection for stage I non-small-cell lung cancer. J Clin Oncol 2009; 28: 928–935
- Hayman JA, Martel MK, Ten Haken RK, Normolle DP, Todd RF, III, Littles JF, Sullivan MA, Possert PW, Turrisi AT, Lichter AS. Dose escalation in non-small-cell lung cancer using three-dimensional conformal radiation therapy: Update of a phase I trial. J Clin Oncol 2001; 19: 127–136
- Chi A, Liao Z, Nguyen NP, Xu J, Stea B, Komaki R. Systemic review of the patterns of failure following stereotactic body radiation therapy in early-stage non-small-cell lung cancer: Clinical implications. Radiother Oncol 2010; 94: 1–11
- Onimaru R, Fujino M, Yamazaki K, Onodera Y, Taguchi H, Katoh N, Hommura F, Oizumi S, Nishimura M, Shirato H. Steep dose-response relationship for stage I non-small-cell lung cancer using hypofractionated high-dose irradiation by real-time tumor-tracking radiotherapy. Int J Radiat Oncol Biol Phys 2008; 70: 374–381
- Koto M, Takai Y, Ogawa Y, Matsushita H, Takeda K, Takahashi C, Britton KR, Jingu K, Takai K, Mitsuva M, et al. A phase II study on stereotactic body radiotherapy for stage I non-small cell lung cancer. Radiother Oncol 2007; 85: 429–434
- Timmerman R, Papiez L, McGarry R, Likes L, DesRosiers C, Frost S, Williams M. Extracranial stereotactic radioablation: Results of a phase I study in medically inoperable stage I non-small cell lung cancer. Chest 2003; 124: 1946–1955
- McGarry RC, Papiez L, Williams M, Whitford T, Timmerman RD. Stereotactic body radiation therapy of early-stage non-small-cell lung carcinoma: Phase I study. Int J Radiat Oncol Biol Phys 2005; 63: 1010–1015
- Mehta M, Scrimger R, Mackie R, Paliwal B, Chappell R, Fowler J. A new approach to dose escalation in non-small-cell lung cancer. Int J Radiat Oncol Biol Phys 2001; 49: 23–33
- Fowler JF. Linear quadratics is alive and well: In regard to Park et al. (Int J Radiat Oncol Biol Phys 2008;70:847–852). Int J Radiat Oncol Biol Phys 2008;72:957; author reply 958.
- Reed CE, Harpole DH, Posther KE, Woolson SL, Downey RJ, Meyers BF, Heelan RT, MacApinlac HA, Jung SH, Silvestri GA, et al. Results of the American College of Surgeons Oncology Group Z0050 trial: The utility of positron emission tomography in staging potentially operable non-small cell lung cancer. J Thorac Cardiovasc Surg 2003; 126: 1943–1951
- Errett LE, Wilson J, Chiu RC, Munro DD. Wedge resection as an alternative procedure for peripheral bronchogenic carcinoma in poor-risk patients. J Thorac Cardiovasc Surg 1985; 90: 656–661
- Timmerman R, McGarry R, Yiannoutsos C, Papiez L, Tudor K, DeLuca J, Ewing M, Abdulrahman R, DesRosiers C, Williams M, et al. Excessive toxicity when treating central tumors in a phase II study of stereotactic body radiation therapy for medically inoperable early-stage lung cancer. J Clin Oncol 2006; 24: 4833–4839
- Brown WT, Perman M, Wu X, Yang J, Schwade JG. Image-guided robotic stereotactic radiosurgery for treatment of lung tumors. Robotic Radiosurgery, RF Mould, RA Schulz, RD Bucholz, GJ Gagnon, PC Gerszten, JJ Kresl, PC Levendag. CyberKnife Society Press, Sunnyvale, CA 2005; 1: 255–268