Abstract
Femoral graft placement is an important factor in the success of anterior cruciate ligament (ACL) reconstruction. In addition to improving the accuracy of femoral tunnel placement, Computer Assisted Surgery (CAS) can be used to determine the anatomic location. This is achieved by using a 3D femoral template which indicates the position of the anatomical ACL center based on endoscopically measurable landmarks. This study describes the development and application of this method. The template is generated through statistical shape analysis of the ACL insertion, with respect to the anteromedial (AM) and posterolateral (PL) bundles. The ligament insertion data, together with the osteocartilage edge on the lateral notch, were mapped onto a cylinder fitted to the intercondylar notch surface (n = 33). Anatomic variation, in terms of standard variation of the positions of the ligament centers in the template, was within 2.2 mm. The resulting template was programmed in a computer-assisted navigation system for ACL replacement and its accuracy and precision were determined on 31 femora. It was found that with the navigation system the AM and PL tunnels could be positioned with an accuracy of 2.5 mm relative to the anatomic insertion centers; the precision was 2.4 mm. This system consists of a template that can easily be implemented in 3D computer navigation software. Requiring no preoperative images and planning, the system provides adequate accuracy and precision to position the entrance of the femoral tunnels for anatomical single- or double-bundle ACL reconstruction.
Introduction
Anatomical double-bundle reconstructions to restore anterior cruciate ligament (ACL) ruptures have become popular over the last few decades. The aim of the double-bundle technique is to restore the anteromedial (AM) bundle as well as the posterolateral (PL) bundle at their anatomical attachment sites, resulting in the most physiological functioning of the restored ACL. A single-bundle AM reconstruction is able to restore anterior translation, especially in the higher flexion region Citation[1–3]. The additional graft, which reconstructs the PL bundle, provides for a more physiological anterior translation in the lower flexion angles and at full extension Citation[4], Citation[5] and, perhaps more importantly, for rotational stability in that range Citation[6–8]. Therefore, a double-bundle reconstruction is theoretically better able to restore normal knee stabilization and kinematics than a traditional single-bundle reconstruction, particularly with regard to reduction of the pivot shift Citation[9–12].
However, the introduction of an extra bundle into the reconstruction technique also means the introduction of more technical difficulties. Since there are two grafts, two tunnels have to be created, and fixation of the bundles can be more complicated. These issues can lead to additional sources of failure Citation[13]. Exact graft placement is one of the most complicated steps during ACL surgery, and large numbers of misplaced grafts have been reported Citation[14–16]. These misplacements can be attributed in part to the nature of the arthroscopic procedure: The view is restricted to a local part of the ligament attachment site, and only a two-dimensional (2D) view of the three-dimensional (3D) reality can be displayed through the endoscope. Since it is difficult to judge depth from a 2D view, and because the view is so limited, it is difficult to find the correct positions for tunnel placement.
Studies have demonstrated the relationship between graft positioning errors and clinical results Citation[17], Citation[18]. Incorrectly placed grafts lead to unstable knees Citation[4], Citation[19], Citation[20], and these patients are prone to developing early osteoarthritis Citation[21]. In an anatomical reconstruction with two bundles, graft placement becomes even more relevant than in single-bundle reconstructions; grafts must be positioned accurately not only with respect to the surrounding tissues, but also relative to one another. Since the femoral positioning is most important Citation[16] and most difficult to achieve Citation[14], this study is focused on the femoral attachment sites.
Basic anatomical studies have improved the description and understanding of the position of the ACL and its two bundles in the femoral notch Citation[22–29]. Studies to develop improved methods for accurate femoral tunnel positioning and drilling have encompassed radiographic guidelines Citation[16], Citation[30], tensiometers and femoral aiming guides Citation[31], Citation[32], computer-assisted fluoroscopic navigation Citation[33–35], computer-assisted real-time navigation Citation[36–41] and robotic-assisted surgery systems Citation[42], Citation[43]. Passive computer-assisted navigation and active robotic-assisted surgery systems can contribute to minimizing variation in graft placement. The initial reports on the use of these innovative systems in ACL reconstruction have provided clear evidence that more exact bone tunnel placement relative to the preferred position can be achieved with both passive and active systems than with conventional techniques Citation[40], Citation[41], Citation[44–47].
Several tools have been developed to find the position for graft placement with the assistance of navigation using a Computer Assisted Surgery (CAS) system. These are based on two different methods: One method uses images, such as preoperative radiographs Citation[37] and CT Citation[43] or intraoperative fluoroscopic computer overlay techniques Citation[44]; the other method is image-free and uses real-time navigation, which means that structures can be digitized during surgery. With the latter method, the 3D anatomical data of the patient's knee is used to find the preferred graft position. In current systems, this method is mostly used to find positions in which notch impingement is avoided, and to locate the most isometric zone Citation[38] for isometric single-bundle ACL reconstruction. However, this is not sufficient for anatomical double-bundle reconstruction, in which the two bundles are attached at their original anatomical positions, which do not coincide with the isometric zone.
If computer navigation systems are to be used for exact anatomical double-bundle placement, the question arises as to the location where the two bundles should be attached. This information is currently lacking, with the result that navigation systems are not used to their full potential in double-bundle reconstructions. With CAS, it is common to morph the individual bone to a standardized bone that is stored within the computer. This approach could also be applied to ACL surgery, standardizing the positions of the insertion centers of the AM and PL bundles. In a previous study Citation[23], we determined the insertion centers of the anteromedial and posterolateral bundles of the ACL in cadaver knees. The purpose of the current study was to generate a standardized template, incorporated in a CAS system, by quantifying the femoral insertion centers of the AM and PL bundles in the notch. To this end, we first developed a template and determined whether the anatomical variation of the center locations in the template was within acceptable limits. We then determined the accuracy and precision of the template as incorporated in the CAS system with respect to positioning of the AM and PL tunnels.
Material and methods
Development of a femoral template
In the first part of this study, a template of the femoral notch including the mean positions of the anatomical insertion centers was developed. Thirty-three human cadaver femoral bones were used for this phase. These femora were previously dissected for an anatomical study of the insertion site of the two bundles of the ACL Citation[23]. The bones were preserved in formalin, and no exact data on the gender and age of the donors was available. However, all donors were over 60 years old, and no gross deformation of the bony structures was observed. On each femur, the outlines of the insertion sites of both the AM bundle and the PL bundle were marked with an ink line. The ACL fibers had been divided during passive knee movement: the fibers of the part that was slack in flexion, the PL bundle, were separated with a sharp scalpel from the fibers that were tight with the knee in that position, i.e., the AM bundle.
To enable definition of the insertion sites in the local coordinate system of the femur, the following points () were digitized using an electromagnetic 3Space Fastrak system (Polhemus Navigation Sciences, Colchester, VT):
I. Ligament outline points: the attachment sites of the ACL and its two separate bundles (AM and PL), by means of points distributed over the outlines of the three insertion areas; II. Notch surface points (roof points): a mapping of the uncovered part of the surface of the lateral and medial wall and the roof of the intercondylar notch, by means of distributed multiple points; and III. Cartilage line points: the posterior joint cartilage edge at the medial wall in the lateral notch, by means of points distributed on the total osteocartilage transition line.
Figure 1. Dorsal view of the femoral intercondylar notch with the areas three-dimensionally digitized by Fastrak: (I) the outlines of the AM (white stars) and PL (black stars) ligament insertion sites; (II) the surface of the lateral notch (including the insertion areas); and (III) the cartilage line of the posterior joint cartilage edge at the medial wall of the lateral condyle (black dots).
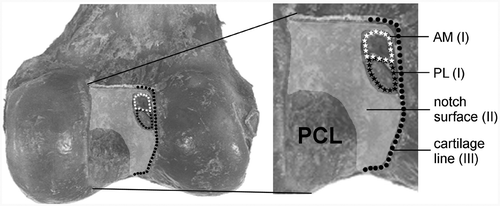
The accuracy of the x, y and z coordinates of each point, as digitized with the stylus, was 0.35 mm Citation[48]. Hence, 33 files were acquired, each containing the femoral ACL insertion sites relative to the anatomical distal geometry of a femur. In the next step, the points were used to define a template including the center positions of the ACL, AM bundle and PL bundle. These centers were determined as the geometric mean of all collected points on the outlines (I).
First, the roof points (II) were used to define a 3D model in the notch, fitting a cylinder shape through these points using a least-squares fit. The roof points determined the radius, orientation and position of the cylinder (). Then, the points of the cartilage line (III) were projected perpendicularly on the surface of the cylinder to define them in the cylindrical coordinate system, using a coordinate in the longitudinal direction (Z in mm) and an angle in the transverse plane (φ in rad) (). Subsequently, the most dorsal point, the apex of the curved posterior joint cartilage edge, was found by fitting a second-order polynomial through its cylinder coordinates by a least-squares fit. The top of this parabola was the most dorsal point and was defined as the origin (0, 0) of the cylindrical coordinate system, determining the cylinder position in the proximal-distal direction and the rotation along the longitudinal axis (). To quantify the validity of the chosen curve-fitting method, the mean explained variances (R2) of all fits were calculated. Finally, the points of the ACL, AM and PL centers were projected perpendicularly on the surface of the cylinder. The positions were determined by the Z coordinate and angle φ in the cylindrical coordinate system. The angle φ determined the X coordinate and the value of the 2D vector D, the distance between the origin and the three centers of the ACL, AM bundle and PL bundle ().
Figure 2. The notch surface in the dorsal view (A), lateral view (B) and notch view (C), which can be approximated by a cylindrical shape. The radius, orientation and position of the cylinder are determined by the roof points (A). The cylinder position in the proximal-distal direction (B) and the rotation along the longitudinal axis (C) were determined by the origin (0, 0) of the cylindrical coordinate systems (also see ).
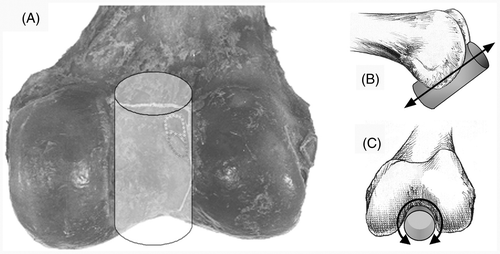
Figure 3. (A) Ventrolateral view of the fitted cylinder with the projected data of the cartilage border and the AM and PL insertion centers. A parabolic curve was fitted through the points of the cartilage border. The top of the parabola was defined as the origin (0, 0) at the cylindrical surface. (B) From the mean center points, the distance in the axial direction (Z) in mm and the angle φ were defined. The direct distance (2D vector D) between the centers and the origin was calculated with Pythagoras’ theorem, with XD being the result of sin 0.5φ*R*2.
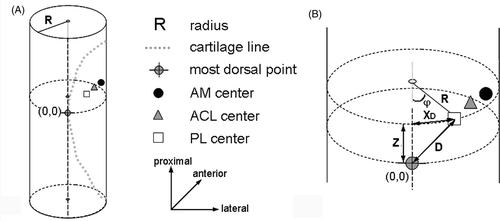
To develop an average cylinder-based insertion definition, the 33 individual cylinders were scaled. The mean radius (R) of all cylinders was calculated, and the ratio of the individual radius to the mean radius resulted in the individual scaling factor. With this factor, each cylinder with the projected points on its surface was scaled. In the last stage of the development, the mean positions of the 33 ACL, AM and PL insertion centers relative to the cylindrical origin were calculated, thereby quantifying the distance Z (in mm), the angle φ (in rad) and the 2D vector D.
To address the first research question, the anatomical variation in the positions of the centers in the developed template was quantified by calculating the SD of the vectors (D) between the centers and the apex of the cartilage border. The 95% CI (as 2 × 1.96 SD) was displayed in both directions in a diagram to visualize the size of the area in which 95% of the anatomical centers were positioned.
Femoral template incorporation
To enable the femoral template to be used for anatomical positioning of the tunnels in the individual patient during ACL reconstruction, it was incorporated in the ACL Replacement Module of the SurgiGATE navigation system (Medivision, Oberdorf, Switzerland). This system and the associated software were developed at the M.E. Müller Institute for Biomechanics at the University of Bern for performing intraoperative planning for ACL replacement Citation[37], Citation[38]. The system consists of an opto-electric camera (Optotrak 3020; Northern Digital, Inc., Waterloo, Ontario, Canada), a computer and computer-tracked devices. The Optotrak system has three cameras that track the 3D positions of light-emitting diodes (LEDs). The LEDs are located on the dynamic reference bases (DRBs), which are rigidly fixated to the femur and tibia of the patient. LEDs are also mounted on specially designed surgical instruments to enable navigated surgery. This system allows for real-time tracking of the bones and interactive digitization and labeling of anatomical structures identified under direct visual or endoscopic control. The interface of the SurgiGATE system guides the surgeon through the steps of acquiring the required landmarks for the template alignment. First, the DRB is fixed to the femur so that it can be used to digitize points on the femur. The Optotrak system tracks the LEDs on the DRB and the ACL module displays them on the monitor. Subsequently, the computer-tracked palpation hook (Stille hook) and a computer-tracked pedicle awl are calibrated. The axes of the femur (the long axis between the trochanter major femoralis and the mid condyles, and the medial-lateral axis between the femoral epicondyles) are digitized with the Stille hook to provide the system with information about the orientation of the femur. During the following steps the surgeon digitizes femoral points, after which the template is aligned in the femoral notch by the program:
The notch surface is digitized as a cloud of points by the surgeon () and visualized by the program with a 3D surface fitted through the points with a Levenberg-Marquardt algorithm (). The cylinder algorithm starts to fit the cylinder template onto the notch surface points, and the size, position and orientation are determined. Since surgical orientation of the reference base on the femur is arbitrary, the system can give several different starting conditions for both algorithms; it chooses the solution having the smallest residual. Refining the notch surface with additional points can also improve cylinder positioning.
The cartilage line is digitized through multiple points on the posterior joint cartilage edge (). After the input of the last point, the system places the template in the notch, positioning the origin of the cylindrical coordinate system at the apex of the curve of the cartilage line (). Rotation about the cylindrical axis and positioning in the proximodistal direction are determined. The template is visualized as a 3D cylinder to verify proper algorithm convergence. The AM, PL and ACL center points are also projected.
The drill-hole location(s) in the notch can be marked with a physical indent of the computer-tracked awl (). The anatomic center, target point for the mark, is visualized on the CAS system monitor, as is the awl ().
Figure 4. (A) Digitizing the notch surface points with the computer-tracked Stille hook. The femur is tracked through the dynamic reference base (DRB). (B) The digitized notch surface is visualized in three dimensions with a Levenberg-Marquardt algorithm for better orientation. The CAS system is calculating the cylinder-algorithm to fit the notch surface. Left: notch view; right: lateral-medial view.
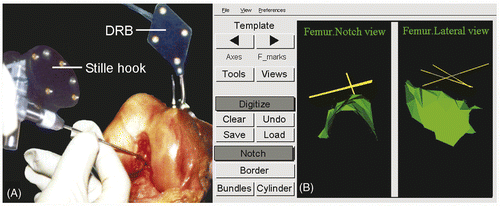
Accuracy and precision of the femoral template
To address the second research question concerning the accuracy and precision of AM and PL tunnel positioning by the template incorporated in the SurgiGATE CAS system, the procedure was tested by the first author (J.L.) on 31 of the 33 specimens from which it was developed. Two specimens of the original group could not be measured as these bones had been used for other experiments in our laboratory. The accuracy and precision were determined by calculating the differences between the positions of the template centers and those of the real anatomic centers in the 3D coordinate system of the CAS system. The mean difference expressed the accuracy of the method, representing how accurately the surgeon could locate the insertion centers using the method. The standard deviation (SD) is a measure of the precision of the method for this group of specimens.
First, the 3D coordinate system of the CAS system was defined () and the templates with the AM and PL centers were positioned in the individual knees. This was done according to the steps specified by the SurgiGATE system. As a result, the template AM (AMt) and PL (PLt) centers in the individual knees were expressed as positions in the defined CAS system 3D coordinate system. In the second step, the system was used as a 3D digitizer to define the 3D position of the anatomic AM (AMa) and PL (PLa) centers in the same CAS 3D coordinate system, similar to the method employed during the development phase. Finally, the differences between anatomic and template centers were defined by calculating the mean and SD of the distances between both centers in the 31 specimens in all three planes of the 3D CAS coordinate system: deep-shallow along the roofline (Z-axis); high-low from the roof (X-axis); and perpendicular to the surface (Y-axis). However, since the tunnel is placed at the bony surface, the distance perpendicular to the surface (Y) is not important and was not evaluated further. The 95% CI was also calculated. To determine the accuracy and precision in a single value, the total distance in the 2D plane of the medial wall of the lateral condyle (deep-shallow Z against high-low X) was calculated as a vector by means of Pythagoras’ theorem.
Figure 7. Three-dimensional coordinate system as defined in the SurgiGATE CAS system for the accuracy and precision measurements of the femoral template. The axes of the femur were defined by digitizing points with the Stille hook. The long Z-axis (white) between the distal point in the middle of the condyles and the middle of the shaft 10 cm proximal above the knee, where the femur was cut, is comparable to the shallow-deep direction in the notch. (This is in contrast with the regular situation, where the trochantor major femoralis is digitized.) The medial-lateral Y-axis (gray line) between the two femoral epicondyles, perpendicular to the notch wall, will not be taken into account, since the tunnel is placed at the bony surface. The X-axis (black line) follows from the other two axes and runs from ventral to dorsal, comparable to the high-low direction in the notch.
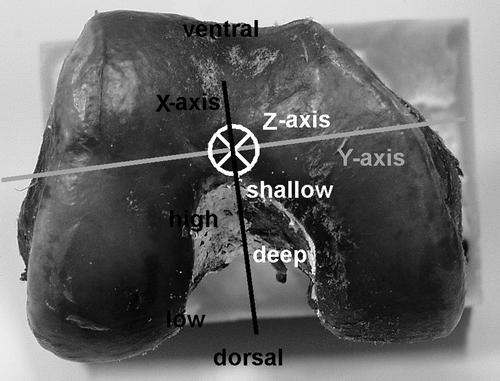
Results
Femoral template
In all 33 femora, a cylinder could be fitted to the notch surface. The mean radius of all cylinders was 10.5 mm (±1.0 mm). The second-degree polynominal curves, which were fitted to the cartilage edge data to create the cylindrical origin, showed a mean explained variance (R2) of 92% (±7%).
The mean distance in the longitudinal direction on the cylinder surface from the most dorsal point of the posterior joint cartilage edge to the center of the total ACL insertion (Z) was 5.9 mm (SD = 1.7). The mean φ-angle in the transverse plane was 0.9 rad (SD = 0.1). The center of the AM was further from the top at 6.9 mm (SD = 1.9) and 1.1 rad (SD = 0.2), while the PL center was closer to the top at 4.5 mm (SD = 2.1) and 0.6 rad (SD = 0.2) ( and ). The area in which 95% of the centers were positioned ranged in the deep-shallow direction from 6.6 mm (ACL) to 7.6 mm (AM) and 8.4 mm (PL). In the high-low direction, 95% of the centers were positioned within 6.0 mm (ACL), 7.0 mm (PL) and 8.0 mm (AM). The variation in the positions of the anatomical centers, as defined by the SD of the direct distance D between the centers and the most dorsal point of the cartilage border (the 2D vector), was 1.7 mm for the ACL, 2.0 mm for the PL, and 2.2 mm for the AM ( and ).
Figure 8. Diagram of the points on the cylinder surface (left) and a lateral view of a femur (with the lateral condyle dissected) (right) showing the alignment of the 3D cylinder template including the projections on the surface of the origin of the coordinate system (the apex of the cartilage border) and the mean position of the centers of the ACL, AM and PL. Z is the distance in the shallow-deep direction; X is the distance in the low-high direction. The error bars represent 1.96*SD, containing 95% of the individual centers (95% CI).
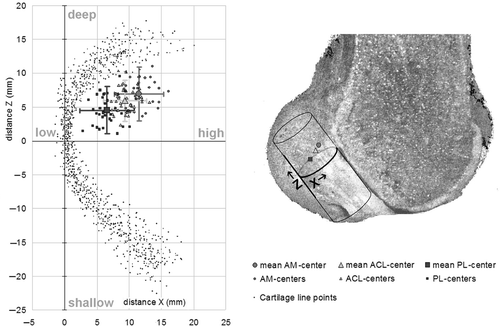
Table I. Mean anatomical positions and variations (SD) of the insertion centers in the template, relative to the apex of the cartilage border.
Accuracy and precision of the femoral template
The accuracy of the system, i.e., the mean distance between the AM and PL centers suggested by the template and the real anatomic centers, was close to 0 mm in all directions ( and ). The area in which 95% of the template centers were positioned was within 7.2 mm in the deep-shallow direction and 7.9 mm in the high-low direction for the AM, and within 7.2 mm and 6.0 mm in those respective directions for the PL, resulting in a precision (SD) in positioning of the AMt and PLt centers compared to the AMa and PLa centers in the deep-shallow direction (Z-axis) of 1.8 mm for both centers. In the high-low direction (X-axis) the precision of the PL was somewhat better than the AM (1.5 mm versus 2.0 mm).
Figure 9. The positions of the template centers relative to the anatomic centers (bull's eye) for AM (A) and PL (B). The circles indicate the distance in mm. The total error is composed of the accuracy, represented by the distances in the high-low and shallow-deep directions between the bull's eye and the mean template centers, and the precision, represented by 1.96*SD (black lines).
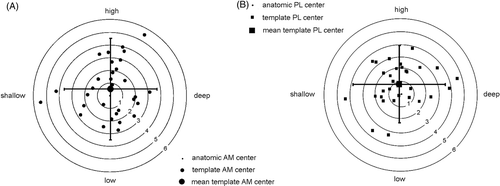
The accuracy in the 2D plane of the condyle wall, representing the absolute deviation of the template centers from the anatomic centers, was 2.5 mm for the AM and 2.2 mm for the PL, with the precision being 1.2 mm for both centers ().
Table II. Accuracy and precision of the tunnels placed by the template using the CAS system, relative to the anatomical centers.
Discussion
Accurate tunnel placement is one of the main difficulties in ACL reconstruction. Various methods, from conventional guiding instruments to computer navigation and robotic systems, have been developed to help the surgeon position tunnel entrances at the desired location, mostly based on isometry or prevention of notch impingement Citation[38]. The use of computer-assisted systems for femoral tunnel positioning during ACL reconstructions has been shown to improve accuracy Citation[44–47].
In anatomical double-bundle ACL reconstruction, the two grafts are supposed to be attached at the original anatomic sites. To the authors’ knowledge, no navigation system exists for determining the femoral tunnel at the anatomical positions for AM and PL grafts based on in situ digitization of landmarks. This study showed the possibility of developing a computer-assisted interactive technique, using a computer model which positions the marks for the femoral tunnel(s) in the individual knee at the anatomical centers. This computer model is an average cylindrical template, developed by statistical shape analysis of 33 femoral notches containing the positions of the centers of the ACL, AM and PL. The anatomical variation of the insertion centers in the cylinder, with SDs ranging between 1.5 and 2.1 mm, was consistent with 95% of the centers lying within 4.3 mm of the mean position (1.96*SD 2D vector, ).
The accuracy of the developed method (2.2 and 2.5 mm for the AM and PL tunnels, respectively) was very similar to the anatomical variation of the real AM and PL centers defined in the template, i.e., 2.2 and 2.0 mm, respectively. The precision in this set of specimens was 1.2 mm for both tunnels. These values are smaller than the usual bone tunnel diameters, of approximately 8–10 mm. These results were comparable with those from several other studies which have quantified the accuracy or precision of ACL navigation systems Citation[40], Citation[43–45]. However, the measurements in three of those studies only compared the position of the preoperatively planned tunnels with the postoperative results, not with the actual position of the anatomical insertion centers; two of the studies Citation[43], Citation[44] used sawbones, and one Citation[45] used cadaveric knees. Musahl et al. Citation[42] defined the accuracy and precision of preoperative tunnel planning with an active robotic system in relation to a tunnel placed in the actual femoral ACL insertion, the values being 1.3 mm (mean) and 1.0 (SD), respectively. The reported precision is similar to our own result, while the accuracy seems superior. However, the system also showed a deviation of 1.3 mm between preoperatively planned tunnels and postoperative results Citation[45].
Disadvantages of the system, as presented in this study, relative to the traditional arthroscopic single-bundle reconstruction technique are, obviously, the costs of the navigation system, the slightly longer operative time (on the order of 10 minutes), and the use of the DRB, which causes an extra wound and subsequent discomfort for the patient. A limitation of this software version was the necessity for visual inspection of the cylinder fit, since algorithm convergence did not always yield the correct solution on the first trial. However, template positioning was possible for each knee in the study, the notch surface being refined with additional points. This limitation could be solved by an algorithm that allows for continuous monitoring of the sufficiency of the input data. Furthermore, the method cannot be used in ACL-deficient knees with notch deformation due to chronic instability.
In addition to the 3D approach, the benefits of the presented system are the use of the CAS system in defining the location of the femoral drill hole, based on the average knee anatomy. Even with several starting conditions, the total calculation time of the optimization algorithm for the cylinder was approximately 10–15 seconds on a standard personal computer. In contrast to other CAS systems, which use radiographic overlays Citation[34], computer graphic overlays added to the fluoroscopic view Citation[44] or CT scans Citation[45], Citation[46], the method proposed in this study requires no extra images and no fluoroscopy. It is a simple CAS arthroscopic method, which enables the surgeon to place the graft at the anatomic AM, PL or ACL center. The SurgiGATE CAS system can also help in using an outside-in drill technique, avoiding notch impingement through virtual planning of the ligaments, and predicting the graft elongation pattern as a function of the knee flexion angle. Pitfalls in tunnel drilling such as dorsal notch outbreak of the drill hole and cartilage damage may be prevented using this method.
Conclusions
The method developed in this study combines anatomic placement with the accuracy of a CAS system. Intraoperative digitization of intra-articular landmarks, which are visible and easily found during endoscopic navigation without the use of other imaging techniques, makes the system easy to use and independent of the preoperative planning methods. With a precision of 2.4 mm, the system has a small variability in anatomical tunnel placement relative to a 10-mm bone tunnel diameter. Further studies are required to validate the system on other specimens and to determine the degree of precision required in anatomic ACL double-bundle reconstruction to affect clinical performance.
Acknowledgments
The authors would like to thank Marwan Sati, Ivan Bourquin, Willem van de Wijdeven, René van der Venne and Huub Peeters for their contributions to this work.
Declaration of interest: This study was funded by grant 99-W14 from the AO Research Fund, Association for Osteosynthesis Foundation, Switzerland.
References
- Mae T, Shino K, Matsumoto N, Hamada M, Yoneda M, Nakata K. Anatomical two-bundle versus Rosenberg's isometric bi-socket ACL reconstruction: A biomechanical comparison in laxity match pretension. Knee Surg Sports Traumatol Arthrosc 2007; 15(4)328–334
- Woo SL, Kanamori A, Zeminski J, Yagi M, Papageorgiou C, Fu FH. The effectiveness of reconstruction of the anterior cruciate ligament with hamstrings and patellar tendon. A cadaveric study comparing anterior tibial and rotational loads. J Bone Joint Surg Am 2002; 84(6)907–914
- Yagi M, Wong EK, Kanamori A, Debski RE, Fu FH, Woo SL. Biomechanical analysis of an anatomic anterior cruciate ligament reconstruction. Am J Sports Med 2002; 30(5)660–666
- Musahl V, Plakseychuk A, VanScyoc A, Sasaki T, Debski RE, McMahon PJ, Fu FH. Varying femoral tunnels between the anatomical footprint and isometric positions: Effect on kinematics of the anterior cruciate ligament-reconstructed knee. Am J Sports Med 2005; 33(5)712–718
- Yamamoto Y, Hsu WH, Woo SL, Van Scyoc AH, Takakura Y, Debski RE. Knee stability and graft function after anterior cruciate ligament reconstruction: A comparison of a lateral and an anatomical femoral tunnel placement. Am J Sports Med 2004; 32(8)1825–1832
- Radford WJ, Amis AA. Biomechanics of a double prosthetic ligament in the anterior cruciate deficient knee. J Bone Joint Surg Br 1990; 72(6)1038–1043
- Yagi M, Wong EK, Kanamori A, Debski RE, Fu FH, Woo SL. Biomechanical analysis of an anatomic anterior cruciate ligament reconstruction. Am J Sports Med 2002; 30(5)660–666
- Loh JC, Fukuda Y, Tsuda E, Steadman RJ, Fu FH, Woo SL. Knee stability and graft function following anterior cruciate ligament reconstruction: Comparison between 11 o’clock and 10 o’clock femoral tunnel placement. Arthroscopy 2003; 19(3)297–304
- Ishibashi Y, Tsuda E, Tazawa K, Sato H, Toh S. Intraoperative evaluation of the anatomical double-bundle anterior cruciate ligament reconstruction with the OrthoPilot navigation system. Orthopedics 2005; 28(10 Suppl)s1277–s1282
- Yasuda K, Kondo E, Ichiyama H, Tanabe Y, Tohyama H. Clinical evaluation of anatomic double-bundle anterior cruciate ligament reconstruction procedure using hamstring tendon grafts: Comparisons among 3 different procedures. Arthroscopy 2006; 22(3)240–251
- Järvelä T. Double-bundle versus single-bundle anterior cruciate ligament reconstruction: A prospective, randomize clinical study. Knee Surg Sports Traumatol Arthrosc 2007; 15(5)500–507
- Yagi M, Kuroda R, Nagamune K, Yoshiya S, Kurosaka M. Double-bundle ACL reconstruction can improve rotational stability. Clin Orthop Relat Res 2007; 454: 100–107
- Harner CD, Poehling GG. Double bundle or double trouble. Arthroscopy 2004; 20(10)1013–1014
- Kohn D, Busche T, Carls J. Drill hole position in endoscopic anterior cruciate ligament reconstruction. Results of an advanced arthroscopy course. Knee Surg Sports Traumatol Arthrosc 1998; 6(Suppl 1)S13–S15
- Topliss C, Webb J. An audit of tunnel position in anterior cruciate ligament reconstruction. Knee 2001; 8(1)59–63
- Behrend H, Stutz G, Kessler MA, Rukavina A, Giesinger K, Kuster MS. Tunnel placement in anterior cruciate ligament (ACL) reconstruction: Quality control in a teaching hospital. Knee Surg Sports Traumatol Arthrosc 2006; 14(11)1159–1165
- Sommer C, Friederich NF, Müller W. Improperly placed anterior cruciate ligament grafts: Correlation between radiological parameters and clinical results. Knee Surg Sports Traumatol Arthrosc 2000; 8(4)207–213
- Moisala AS, Järvelä T, Harilainen A, Sandelin J, Kannus P, Järvinen M. The effect of graft placement on the clinical outcome of the anterior cruciate ligament reconstruction: A prospective study. Knee Surg Sports Traumatol Arthrosc 2007; 15(7)879–887
- Markolf KL, Hame S, Hunter DM, Oakes DA, Zoric B, Gause P, Finerman GA. Effects of femoral tunnel placement on knee laxity and forces in an anterior cruciate ligament graft. J Orthop Res 2002; 20(5)1016–1024
- Zavras TD, Race A, Amis AA. The effect of femoral attachment location on anterior cruciate ligament reconstruction: Graft tension patterns and restoration of normal anterior-posterior laxity patterns. Knee Surg Sports Traumatol Arthrosc 2005; 13(2)92–100
- Jonsson H, Kärrholm J, Elmqvist LG. Laxity after cruciate ligament injury in 94 knees. The KT-1000 arthrometer versus roentgen stereophotogrammetry. Acta Orthop Scand 1993; 64(5)567–570
- Takeuchi R, Saito T, Mituhashi S, Suzuki E, Yamada I, Koshino T. Double-bundle anatomic anterior cruciate ligament reconstruction using bone-hamstring-bone composite graft. Arthroscopy 2002; 18(5)550–555
- Luites JW, Wymenga AB, Blankevoort L, Kooloos JG. Description of the attachment geometry of the anteromedial and posterolateral bundles of the ACL from arthroscopic perspective for anatomical tunnel placement. Knee Surg Sports Traumatol Arthrosc 2007; 15(12)1422–1431
- Colombet P, Robinson J, Christel P, Franceschi JP, Djian P, Bellier G, Sbihi A. Morphology of anterior cruciate ligament attachments for anatomic reconstruction: A cadaveric dissection and radiographic study. Arthroscopy 2006; 22(9)984–992
- Mochizuki T, Muneta T, Nagase T, Shirasawa S, Akita KI, Sekiya I. Cadaveric knee observation study for describing anatomic femoral tunnel placement for two-bundle anterior cruciate ligament reconstruction. Arthroscopy 2006; 22(4)356–361
- Takahashi M, Doi M, Abe M, Suzuki D, Nagano A. Anatomical study of the femoral and tibial insertions of the anteromedial and posterolateral bundles of human anterior cruciate ligament. Am J Sports Med 2006; 34(5)787–792
- Edwards A, Bull AM, Amis AA. The attachments of the anteromedial and posterolateral fibre bundles of the anterior cruciate ligament. Part 2: Femoral attachment. Knee Surg Sports Traumatol Arthrosc 2007; 16(1)29–36
- Ferretti M, Ekdahl M, Shen W, Fu FH. Osseous landmarks of the femoral attachment of the anterior cruciate ligament: An anatomic study. Arthroscopy 2007; 23(11)1218–1225
- Iriuchishima T, Ingham SJ, Tajima G, Horaguchi T, Saito A, Tokuhashi Y, van Houten AH, Aerts MM, Fu FH. Evaluation of the tunnel placement in the anatomical double-bundle ACL reconstruction: A cadaver study. Knee Surg Sports Traumatol Arthrosc 2010; 18(9)1226–1231
- Bernard M, Hertel P, Hornung H, Cierpinski T. Femoral insertion of the ACL. Radiographic quadrant method. Am J Knee Surg 1997; 10(1)14–21
- Milankov M, Miljkovic N. A new positioning device for precise femoral insertion of the anterior cruciate ligament autograft. Knee Surg Sports Traumatol Arthrosc 2000; 8(3)149–153
- Hantes ME, Dailiana Z, Zachos VC, Varitimidis SE. Anterior cruciate ligament reconstruction using the Bio-TransFix femoral fixation device and anteromedial portal technique. Knee Surg Sports Traumatol Arthrosc 2006; 14(5)497–501
- Hiraoka H, Kuribayashi S, Fukuda A, Fukui N, Nakamura K. Endoscopic anterior cruciate ligament reconstruction using a computer-assisted fluoroscopic navigation system. J Orthop Sci 2006; 11(2)159–166
- Klos TV, Banks SA, Habets RJ, Cook FF. Sagittal plane imaging parameters for computer-assisted fluoroscopic anterior cruciate ligament reconstruction. Comput Aided Surg 2000; 5(1)28–34
- Pace A, Fergusson C. Preoperative computerized radiograph measurement of Blumensaat's line as an aid to correct placement of the femoral tunnel in ACL reconstruction. J Knee Surg 2006; 19(3)176–180
- Dessenne V, Lavallée S, Julliard R, Orti R, Martelli S, Cinquin P. Computer-assisted knee anterior cruciate ligament reconstruction: First clinical tests. J Image Guid Surg 1995; 1(1)59–64
- Sati M, Stäubli H, Bourquin Y, Kunz M, Käsermann S, Nolte LP. Clinical integration of computer-assisted technology for arthroscopic anterior cruciate ligament reconstruction. Operat Tech Orthoped 2000; 10(1)40–49
- Sati M, Stäubli H, Bourquin Y, Kunz M, Nolte LP. Real-time computerized in situ guidance system for ACL graft placement. Comput Aided Surg 2002; 7(1)25–40
- Müller-Alsbach UW, Staubli AE. Computer aided ACL reconstruction. Injury 2004; 35(Suppl 1)S-7
- Plaweski S, Cazal J, Rosell P, Merloz P. Anterior cruciate ligament reconstruction using navigation: A comparative study on 60 patients. Am J Sports Med 2006; 34(4)542–552
- Ishibashi Y, Tsuda E, Fukuda A, Tsukada H, Toh S. Future of double-bundle anterior cruciate ligament (ACL) reconstruction: incorporation of ACL anatomic data into the navigation system. Orthopedics 2006; 29(10 Suppl)S108–S112
- Musahl V, Burkart A, Debski RE, van Scyoc A, Fu FH, Woo SL. Anterior cruciate ligament tunnel placement: Comparison of insertion site anatomy with the guidelines of a computer-assisted surgical system. Arthroscopy 2003; 19(2)154–160
- Petermann J, Kober R, Heinze R, Frölich JJ, Heeckt P, Gotzen L. Computer-assisted planning and robot-assisted surgery in anterior cruciate ligament reconstruction. Operat Tech Orthoped 2000; 10(1)50–55
- Klos TV, Habets RJ, Banks AZ, Banks SA, Devilee RJ, Cook FF. Computer assistance in arthroscopic anterior cruciate ligament reconstruction. Clin Orthop Relat Res 1998, 354: 65–69
- Picard F, DiGioia AM, Moody J, Martinek V, Fu FH, Rytel M, Nikou C, LaBarca RS, Jaramaz B. Accuracy in tunnel placement for ACL reconstruction. Comparison of traditional arthroscopic and computer-assisted navigation techniques. Comput Aided Surg 2001; 6(5)279–289
- Burkart A, Debski RE, McMahon PJ, Rudy T, Fu FH, Musahl V, van Scyoc A, Woo SLY. Precision of ACL tunnel placement using traditional and robotic techniques. Comput Aided Surg 2001; 6(5)270–278
- Musahl V, Burkart A, Debski RE, van Scyoc A, Fu FH, Woo SL. Accuracy of anterior cruciate ligament tunnel placement with an active robotic system: A cadaveric study. Arthroscopy 2002; 18(9)968–973
- Sidles JA, Larson RV, Garbini JL, Downey DJ, Matsen FA, III. Ligament length relationships in the moving knee. J Orthop Res 1988; 6(4)593–610