Abstract
Introduction: Advances in surgical navigation have opened new possibilities for lumbosacral interbody fusion procedures. We have designed a novel navigated surgical method, Navigated Percutaneous Lumbosacral Interbody Fusion (NPLSIF), that enables lumbosacral discectomy and bone grafting to be performed percutaneously and safely.
Methods: To prove that NPLSIF is feasible from an anatomical perspective, it was simulated on 3D models of the lumbosacral spine created using CT data from 60 patients. Feasibility would be verified if both the working corridor and the S1 pedicle screw could be accommodated in the sacral ala without overlapping and without penetrating either the spinal canal or the anterior or upper sacral wall. In addition, the discectomy that could be achieved using NPLSIF was evaluated, and a surgical experiment was performed using a plastic torso model.
Results: The 3D modeling and surgical simulation were successfully completed in all cases. The feasibility of the NPLSIF approach was verified in every case, i.e., both the working corridor and the S1 pedicle screw were accommodated in the sacral ala without overlapping and without penetrating either the spinal canal or the anterior or upper sacral wall. The mean ratio of the area of discectomy that could be achieved by NPLSIF to the total area of the lumbosacral disc was 0.721 ± 0.065 (range: 0.57–0.894), 0.956 ± 0.045 (range: 0.8–1.0) and 0.945 ± 0.058 (range: 0.813–1.0) in the axial, coronal and sagittal planes, respectively. NPLSIF was also successfully executed on the plastic torso model. Preoperative planning on the navigation workstation took 5 minutes and each intraoperative CT scan took 30 seconds. Locating the entry point of the working corridor according to the preoperative plan took 3 minutes. Postoperative CT images and direct viewing of the plastic model revealed no penetration of the spinal canal or sacral wall.
Conclusion: The feasibility of Navigated Percutaneous Lumbosacral Interbody Fusion (NPLSIF) was verified from an anatomical perspective. We also demonstrated that an adequate discectomy can be achieved using the procedure. Cadaveric experiments and clinical trials are needed to further evaluate the efficacy and efficiency of NPLSIF.
Introduction
Lumbosacral interbody fusion is a common surgical procedure: As approximately half of all degenerative disc disorders occur at the L5/S1 level Citation[1], Citation[2], it accounts for a large proportion of spine surgeries. While this procedure traditionally requires an open surgical approach, advances in technique and technology have led to the development of several minimally invasive techniques, including percutaneous transforaminal lumbar interbody fusion (pTLIF) Citation[3] and axial lumbar interbody fusion (AxiaLIF) Citation[4], Citation[5]; however, both of these techniques have limitations. Percutaneous TLIF at L5/S1 is technically demanding and sometimes inapplicable, because in many patients the lumbosacral disc is wedge-shaped or the intervertebral space is too narrow, either of which can make it impossible to perform discectomy and bone grafting from a posterior approach. Although AxiaLIF is specially designed for L5/S1 interbody fusion, spine surgeons are generally not familiar with the presacral anatomy and potential complications continue to be associated with inadvertent injury to the surrounding structures.
Navigated spine surgery using infrared real-time tracking technology was developed approximately 15 years ago Citation[6], but the latest advances in surgical navigation have enabled new approaches to lumbosacral interbody fusion. To explore such new possibilities, we designed a novel surgical procedure, Navigated Percutaneous Lumbosacral Interbody Fusion (NPLSIF), and conducted a series of experiments to verify its feasibility, including a three-dimensional (3D) surgical simulation using CT data and a surgical experiment using a plastic torso model.
Materials and methods
Navigated Percutaneous Lumbosacral Interbody Fusion (NPLSIF)
NPLSIF is a percutaneous procedure for lumbosacral interbody fusion using navigation technology. It involves percutaneous discetomy, bone grafting, and pedicle screw placement. The key step in NPLSIF is the creation of a working corridor that passes through the ilium and sacral ala and proceeds into the lumbosacral disc (). Percutaneous discectomy and bone grafting are then performed through this working corridor.
Feasibility verification from an anatomical perspective
Three-dimensional surgical simulations were performed using CT data and Mimics 12.3 software (Materialise, Inc., Leuven, Belgium). Data from 60 patients (26 females, 34 males; mean age: 55.45 years; range: 17–92 years) who had undergone abdominal CT angiography were included. Most of these patients had been examined to diagnose blood vessel diseases and tumors; none of them was being treated for spinal pathology, and there was no visible pathology except for osteoporosis in their spines. All the CT data were obtained from the same hospital, and each CT data set included the entire lumbar spine, sacrum and ilia. The CT slice distance was 1 mm.
To construct the 3D models of the lumbosacral spine, the CT data were imported into the Mimics software, which was run on a desktop computer. First, S1 pedicle screw placement on the right side was simulated. We then simulated the creation of a working corridor (). Feasibility would be verified if both the working corridor and the S1 pedicle screw could be accommodated in the sacral ala without overlapping and without penetrating either the spinal canal or the anterior or upper sacral wall.
Figure 2. Simulated creation of the working corridor. The blue cylinder (10 mm in diameter) represents the working corridor and the brown cylinder (7.5 mm in diameter) represents the S1 pedicle screw. The working corridor started from the right ilium and proceeded into the center of the lumbosacral disc. The position and direction of the corridor could be adjusted to keep it within the safe zone, i.e., avoiding penetration of the spinal canal or the anterior or upper sacral wall. Feasibility would be verified if both the working corridor and the S1 pedicle screw could be accommodated in the sacral ala without overlapping and without penetrating either the spinal canal or anterior or upper sacral wall. The arrow points to the interspace between the corridor and the screw trajectory, which demonstrates that there is no overlap.
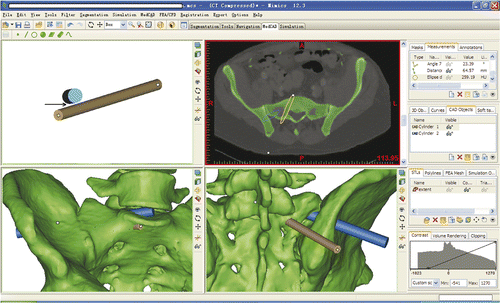
Evaluation of the discectomy
The area of the discectomy that could be achieved with NPLSIF would determine whether or not an adequate discectomy could be achieved using the procedure. A 3D cone, representing the maximum extent that our navigated curettes could reach, was imported into the Mimics software and incorporated with the working corridor, so that the maximum extent could be displayed in the axial, coronal and sagittal planes (). As a result, the area of the discectomy achievable with NPLSIF and the total area of the lumbosacral disc could be measured with ImageJ software (National Institutes of Health; http://rsbweb.nih.gov/ij/) and the ratio between them calculated. The 3D cone was defined on the basis of a series of measurements of the navigated curettes: knowing their length, diameter and angulation, the maximum theoretical extent of their reach was calculated. The results were then input into 3ds max software (Autodesk Inc., San Rafael, CA) to establish the 3D cone in order to represent this maximum extent.
Feasibility verification with a surgical experiment
To verify the feasibility of the NPLSIF procedure in reality, a surgical experiment was conducted using a plastic torso. During the experiment, the plastic torso was placed in the prone position, and a patient reference base was fixed to the right iliac crest of the torso for tracking purposes. The patient reference base was fitted with infrared reflectors and could thus be tracked by the navigation system. The torso was then scanned with an intraoperative CT scanner (O-arm, Medtronic SNT, Louisville, CO), and the CT image data were transferred within 1 minute from the scanner to the navigation system (StealthStation S7, Medtronic SNT). Consequently, the pelvis and lumbar spine of the torso could be tracked by the navigation system in real time (), along with the navigated instruments. The real-time locations of both the spine and the instruments were displayed on the screen of the navigation system so that the procedure could be performed under real-time 3D guidance.
Figure 4. The set-up of the surgical experiment showing the plastic torso model (A), the intraoperative CT scanner (B), the navigation workstation (C), and the navigated instruments (D). A patient reference was fixed to the right iliac crest of the plastic torso, which was then scanned with the intraoperative CT scanner. The CT image data were transferred to the navigation system within 1 minute. As a result, the pelvis and lumbar spine of the torso could be tracked by the navigation system in real time.
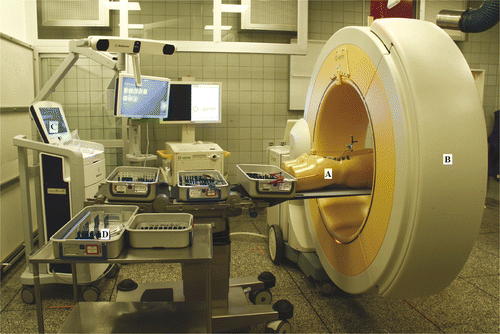
Next, a preoperative plan for the working corridor was made on the navigation workstation. The position and direction of the working corridor could be adjusted on the screen of the workstation, so that the corridor could be made within the safe zone, i.e., without penetrating either the spinal canal or the upper or anterior sacral wall.
The procedure was then undertaken using the navigated instruments in accordance with the preoperative plan. The navigated awl and taps of various sizes were used in sequence to establish the working corridor (). The discectomy and endplate preparation were performed using navigated curettes of various shapes. All the curettes were tracked in real time so that they could be kept working within the safe zone.
Figure 5. Establishment of the working corridor was performed according to the preoperative plan. The navigated awl and taps of various sizes were used in sequence. (A1) Using the navigated awl to locate the planned entry point. (A2) The green trajectory is the preoperative plan for the working corridor; the purple cylinder represents the real-time location of the navigated awl. (B1) After inserting the navigated awl into the lumbosacral disc according to the preoperative plan, the navigated tap is inserted gradually to enlarge the working corridor. (B2) While the tap is proceeding, its direction can be adjusted as necessary under the guidance of the system.
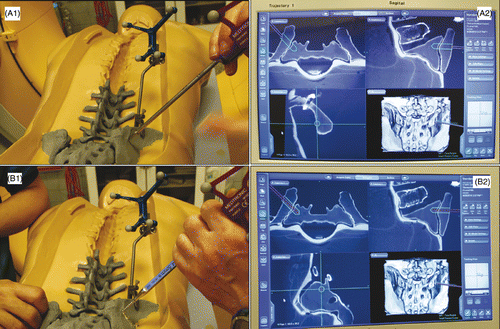
Finally, postoperative CT scanning was performed and the CT images checked slice by slice to see if there was any penetration of the spinal canal or sacral wall.
Results
Feasibility verification from an anatomical perspective
The 3D modeling and surgical simulation were successfully completed in all cases. The feasibility of the NPLSIF approach was verified in every case, i.e., both the working corridor and the S1 pedicle screw were accommodated in the sacral ala without overlapping and without penetrating either the spinal canal or the anterior or upper sacral wall. In 4 of the 60 cases, the working corridor penetrated the sacroiliac joint. The mean length of the working corridors was 58.00 ± 5.09 mm, while the mean length of the virtual S1 screws was 51.00 ± 6.55 mm (). The phenomenon of lumbosacral transitional vertebra was found in 4 of the 60 cases, but the deformity did not impede the surgical simulations. Significant osteoporosis was found in 23 cases. Osteoporosis has a negative effect on 3D image reconstruction; the lower the bone density, the more difficult it is for the software to differentiate the bone from soft tissues. However, satisfactory image quality could still be achieved by adjusting the threshold value. The time consumed by surgical simulation was 5.62 ± 1.58 min.
Table I. Parameters of screw trajectories and working corridors.
Evaluation of the discectomy
The mean ratio of the area of the discectomy that could be achieved by NPLSIF to the total area of the lumbosacral disc was 0.721 ± 0.065 (range: 0.57–0.894), 0.956 ± 0.045 (range: 0.8–1.0) and 0.945 ± 0.058 (range: 0.813–1.0) in the axial, coronal and sagittal planes, respectively ().
Figure 6. In the axial and sagittal planes that passed through the center of the lumbosacral disc, the area of the discectomy that could be achieved using NPLSIF and the total area of the disc were measured with ImageJ software and the ratio calculated. In this case, the ratio in the axial plane is 1.481/2.120 = 0.699, and the ratio in the sagittal plane is 0.354/0.371 = 0.954.
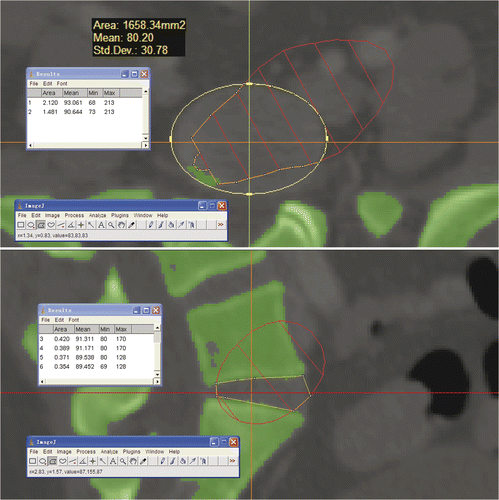
Feasibility verification with a surgical experiment
The NPLSIF procedure was successfully executed on the plastic torso model. On the navigation workstation, the preoperative plan took 5 min and each intraoperative CT scan took 30 seconds. Locating the entry point of the working corridor according to the preoperative plan took 3 min. Postoperative CT images and direct viewing of the plastic model showed no penetration of the spinal canal or sacral wall.
Discussion
Lumbosacral interbody fusion and current minimally invasive techniques
Lumbosacral interbody fusion is widely performed by spine surgeons all over the world. Currently, the minimally invasive techniques for lumbosacral interbody fusion include percutaneous transforaminal lumbar interbody fusion (pTLIF) and axial lumbar interbody fusion (AxiaLIF). Neither of these techniques is, however, without its drawbacks.
Percutaneous TLIF at L5/S1 is technically demanding: a total facetectomy on one side is required and the working corridor has to pass through the space between the dural sac and exiting nerve root, while care must be taken to protect the exiting and traversing nerve root during discetomy and bone grafting. Most importantly, in many patients either the lumbosacral disc is wedge-shaped or the intervertebral space is very narrow, both of which can preclude performance of a pTLIF. The CT images in our current study showed an extremely narrow intervertebral space in 6 of the 60 patients, so for these 6 patients pTLIF was not an option.
AxiaLIF is specially designed for lumbosacral interbody fusion Citation[4], Citation[5]. Following a minimally invasive incision, access to the lumbosacral disc is obtained through the presacral space. Although the presacral anatomy is familiar to general and colo-rectal surgeons, spine surgeons are generally not as comfortable with this approach. As with any surgical approach, it is critical that the operating surgeon has a thorough understanding of the presacral anatomy in order to reduce the risk of injury to the surrounding structures, and complications continue to be associated with such injury.
Advances in surgical navigation and new possibilities
Computer assisted spinal surgery was first developed approximately 15 years ago using infrared real-time tracking technology Citation[6]. With the recent development of intraoperative CT scanners, several problematic issues associated with surgical navigation, such as time-consuming registration processes Citation[7] and poor image quality Citation[8], have been satisfactorily resolved. These issues formerly undermined the practical value of navigation surgery. When connected to the intraoperative CT scanner, the navigation system can provide highly accurate 3D surgical guidance for implant placement. This guidance function can also be used to accurately establish a corridor in bone, which may open new possibilities for lumbosacral interbody fusion. If a corridor can be accurately established in the ilium and sacral ala, it is possible to perform discectomy and bone grafting through it. In addition, use of navigation eliminates the need for exposure, enabling the procedure to be performed percutaneously. Based on these hypotheses, we designed a new procedure: Navigated Percutaneous Lumbosacral Interbody Fusion (NPLSIF).
Verifying the feasibility of NPLSIF entailed proving that it is feasible from an anatomical perspective. The NPLSIF procedure was therefore simulated on 3D spine models of 60 patients. The results showed that in each case both the working corridor and the S1 pedicle screw were accommodated in the sacral ala without overlapping and without penetrating either the spinal canal or the anterior or upper sacral wall. In other words, the feasibility of NPLSIF from an anatomical perspective was verified in every case.
Next, the extent of the discectomy that could be achieved using NPLSIF was evaluated. In each case, the area of disctectomy that could be achieved and the total area of the lumbosacral disc were measured and the ratio calculated. The results showed that, in theory, the navigated curettes could reach approximately 70% of the disc in the axial plane, and approximately 90% of the disc in either the coronal or sagittal plane. In other words, adequate discetomy can be achieved by the NPLSIF procedure. However, the efficacy of the NPLSIF discectomy requires further evaluation in cadaveric and clinical studies.
Finally, to further validate the NPLSIF concept, a surgical experiment with a plastic torso model was conducted. On the navigation workstation, the preoperative planning took 5 min and each intraoperative CT scan took 30 seconds. Postoperative CT images and direct viewing of the plastic model showed no penetration of the spinal canal or sacral wall. One of the limitations of our current study is that the area of discectomy in this experiment could not be measured because the lumbosacral disc of the plastic model was X-ray transparent. Further cadaveric experiments should be performed to evaluate the efficacy and efficiency of the discectomy and endplate preparation when using NPLSIF.
Advantages of the new method
NPLSIF could be more advantageous than other minimally invasive techniques, as its application does not depend on the shape of the lumbosacral disc. In addition, because the working corridor is within the ilium and sacral ala, there is no contact with nerves or vessels, so NPLSIF may prove safer than other techniques. Because the working corridor connects the autobone graft in the intervertebral space with the bone marrow of the sacrum, fusion rates might increase, but this possibility remains to be confirmed by further studies. A further potential application of NPLSIF is to strengthen the construct of a long posterior spinal fusion that is extended down to the sacrum, because the weak point of such a construct is usually located at the S1 level. Lastly, because the navigation is based on infrared tracking technology, exposure of the operating room personnel to radiation can be completely avoided.
Conclusion
This study verified the feasibility of Navigated Percutaneous Lumbosacral Interbody Fusion (NPLSIF) from an anatomical perspective. We also demonstrated that an adequate discectomy can be achieved using NPLSIF. Cadaveric experiments and clinical trials are necessary to further evaluate the efficacy and efficiency of the NPLSIF procedure.
Acknowledgments
The authors wish to thank Michel Bach Hellfritzsch for his assistance in collecting CT data, and Linda Marie Nygaard for her revisions of the manuscript.
References
- Herkowitz HN, Abraham DJ, Albert TJ. Management of degenerative disc disease above an L5-S1 segment requiring arthrodesis. Spine 1999; 24(12)1268–1270
- Freeman BJ, Davenport J. Total disc replacement in the lumbar spine: A systematic review of the literature. Eur Spine J 2006; 15(Suppl 3)S439–S447
- Humphreys SC, Hodges SD, Patwardhan AG, Eck JC, Murphy RB, Covington LA. Comparison of posterior and transforaminal approaches to lumbar interbody fusion. Spine 2001; 26: 567–571
- Cragg A, Carl A, Casteneda F, Dickman C, Guterman L, Oliveira C. New percutaneous access method for minimally invasive anterior lumbosacral surgery. J Spinal Disord Tech 2004; 17: 21–28
- Marotta N, Cosar M, Pimenta L, Khoo LT. A novel minimally invasive presacral approach and instrumentation technique for anterior L5-S1 intervertebral discectomy and fusion: Technical description and case presentations. Neurosurg Focus 2006; 20: E9
- Nolte LP, Zamorano L, Visarius H, Berlemann U, Langlotz F, Arm E, Schwarzenbach O. Clinical evaluation of a system for precision enhancement in spine surgery. Clin Biomech 1995; 10: 293–303
- Wang HC, Yang YL, Lin WC, Chen WF, Yang TM, Lin YJ, Rau CS, Lee TC. Computer-assisted pedicle screw placement for thoracolumbar spine fracture with separate spinal reference clamp placement and registration. Surgical Neurol 2008; 69: 597–601
- Hott JS, Deshmukh VR, Klopfenstein JD, Sonntag VK, Dickman CA, Spetzler RF, Papadopoulos SM. Intraoperative Iso-C C-arm navigation in craniospinal surgery: The first 60 cases. Neurosurgery 2004; 54: 1131–1136