Abstract
Stereotactic radiosurgery (SRS) has been proposed as an alternative treatment modality to pharmaceutical administration and deep brain stimulation (DBS) for patients suffering from movement disorders. Advanced neuroimaging is required for the identification of the functional structures and the accurate placement of the SRS lesion within the brain. Atlas-based techniques have also been used to aid delineation of the target during treatment planning. Maximum doses greater than 120 Gy have been suggested for controlling movement disorders. These high delivered doses and the irreversible character of SRS require accurate placement of the created lesions. In this article, achievements in the field of stereotactic radiosurgery, neuroimaging, and radiosurgical dose planning are reviewed, and an overview is provided of the clinical experience obtained to date in the radiosurgical treatment of movement disorders.
Introduction
The ability to precisely define the location and dimensions of a lesion is considered an absolute prerequisite for a correct neurosurgical approach to the treatment of movement disorders. Thus, following the pioneering introduction of stereotactic radiosurgery (SRS) by Leksell Citation[1], SRS was proposed as a non-invasive approach to treating functional disorders because of its ability to produce a lesion with a location and dimensions that precisely match a pre-treatment surgical plan. The observation by Guiot and Cooper Citation[2] that ablative procedures can modify and correct malfunctioning neuronal circuits involving the cortical motor areas and the basal ganglia further advanced the utility of SRS for movement disorders, with stereotactic lesions of selected anatomical targets being increasingly used in patients with Parkinson's disease (PD) and other movement disorders. With the exception of deep brain stimulation (DBS), the introduction of pharmacological treatments has shifted many treatments for patients with movement disorders away from the surgical field; however, a small number of neurosurgical centers have continued to develop experience with surgical lesions. In recent years, these centers have led a renewed interest in the use of SRS for movement disorders with the aim of reducing the invasiveness of treatment, especially for patients with advanced illness and co-morbidities that expose them to a higher operative risk. In this article we review achievements in the field of stereotactic radiosurgery, neuroimaging, and radiosurgical dose planning, and provide an overview of the clinical experience obtained to date in the radiosurgical treatment of movement disorders. Finally, an application of the described radiosurgical technique to a patient suffering from severe dystonia is presented.
Ablative procedure for treating movement disorders
For a long time after the advent of levodopa (L-DOPA), lesioning procedures were restricted to selected patients wherein functional neurosurgery interfered with pathological neuronal circuits involved in movement disorders. The principal issue arising when an ablative neurosurgical procedure is proposed for a movement disorder is, of course, whether the production of irreversible brain tissue damage could cause a deterioration in the patient's neurological performance. Cognitive deterioration following bilateral lesioning is a well-known complication of lesional procedures, but placement of the lesion within unwanted brain structures such as the internal capsule might also occur. In contrast, DBS is a type of reversible “functional” lesioning procedure, in that switching off the high-frequency stimulation will stop the inhibitory effect on the targeted neuronal population. Common targets used nowadays to treat the symptoms of movement disorders include the sensorimotor regions of the subthalamic nucleus (STN), the internal globus pallidus (GPi), and thalamic nuclei such as the nucleus ventralis intermedius (VIM). The subthalamic nucleus is a widely used target for addressing the cardinal symptoms of PD (rigidity, resting tremor, and bradyknesia), as well as a series of other disturbances (freezing, on-off phenomena, and dyskinesias) that are associated with end-stage PD and induced by chronic administration of levodopa or other dopaminergic drugs. The GPi target can be used to treat end-stage PD and dystonias, while the VIM is an excellent target for controlling intention tremor. Each of these nuclei is part of the cortico-basal ganglia-thalamocortical loop; this complex network is embedded within a densely packed network where deep gray nuclei and connecting fibers are arranged according to a strict topographic organization.
Postoperative morbidity following the placement of a brain lesion is mainly influenced by the inclusion of unwanted nuclei or fiber tracts within the lesional volume. Intraoperative neurophysiological monitoring (IOM) can guide the neurosurgeon to correctly localize the target and minimize side effects related to the stimulation or lesioning procedure. SRS offers a truly non-invasive tool for placing lesions within the brain, but is also limited by the inability to confirm the correct placement of the lesional volume using IOM. Consequently, anatomic identification of the radiosurgical target becomes of paramount importance. Recent advances in the field of neuroimaging, namely the possibility of enhancing the identification of the STN, VIM and GPi and the contrast between these nuclei and the surrounding white matter using high-power MRI, can help in this regard.
Image guided target identification
When considering SRS for treatment of a functional disorder, the imaging data on which the treatment planning will be executed must be highly accurate in terms of resolution of the deep gray nuclei, such as the STN, VIM and GPi, and their boundaries with highly eloquent adjacent fiber tracts. Centers with state-of the-art experience of functional neurosurgery, where accurate imaging is of paramount importance in the placement of quadripolar electrodes for DBS treatment of movement disorders, can easily import their functional neurosurgery imaging protocols to enhance the identification of basal ganglia targets.
Whether “direct” or “indirect” targeting should be considered optimal remains controversial and, as addressed in a review by Rezai et al. Citation[3], there is no clear evidence in favor of one approach or the other. Nevertheless, direct identification of the target using a 1.5-T MRI (or 3-T, if available) is preferred by most practitioners. Indirect targeting based on ventriculography was developed when no direct visualization of the target nuclei could be performed, and it is based on the more or less reliable spatial relationships between basal ganglia targets and critical intraventricular landmarks such as the anterior and posterior commissure. Because of its invasiveness, ventriculography has been to a large extent replaced by CT and MRI, the latter allowing for a much-improved resolution of the brain tissue in which the STN and GPi can be easily identified on T2 FSE imaging Citation[4]; however, it is a matter of debate whether the MRI-visible areas of those structures are merely the iron-rich parts, and thus not representative of the true extent of the nucleus Citation[5]. The introduction of 3-T and volumetric MR imaging offers enhanced visualization of basal ganglia and thalamic targets, and also allows identification of targets such as the VIM that are not otherwise visible using most 1.5-T MRI scanners Citation[5–7]. Further improvement should be expected with advances in MRI techniques and scanners, as pointed out in a recent article by Cho et al. Citation[8], who analyzed the possible impact on direct visualization of targets in patients with movement disorders using imaging generated by a 7-T MRI scanner.
While more accurate image-based identification of the target to be treated by DBS can be useful, it is not indispensable to the success of the procedure due to the possibility of correcting the final position of the electrode by taking into account information from the microelectrode recording. In contrast, direct visualization of the target plays a key role in SRS ablation, which is an irreversible procedure based on a non-invasive approach that does not offer the chance to confirm the target location electrophysiologically. We have developed and validated an accurate semi-automatic method for three-dimensional (3D) mapping of the nuclei relevant to functional neurosurgery and radiosurgery, such as the STN, the GPi and globus pallidus pars externa (GPe), the centromedian nucleus (CMN), and the red nucleus Citation[9]. Atlas-based volumetric reconstruction provides further proof that the target is within the planned lesional volume and can confirm the target location provided through direct visualization. Since it was proven safe to perform MRI scans of patients with implanted DBS electrodes, several studies Citation[10–13] have investigated the relationship between the preoperative location of the target and postoperative electrode placement with respect to the clinical outcome. However, no experience is available regarding the implementation of a localizing tool based on a highly refined atlas overlaid through multiple fusion procedures on the intended target.
We performed MRI studies on 10 patients with implanted electrodes in the STN and GPi to generate a method to automatically localize those nuclei Citation[9]. The precision of this technique was tested using different parameters such as the distance from commonly used anatomical landmarks (the AC-PC line), the distance between the centers of mass of the nuclei and the implanted DBS electrode, and the volume of the nuclei. These parameters were then compared with those from the Montreal Neurological Institute Atlas and anatomical data available in the literature. Lastly, this was correlated with the clinical outcomes in patients. Distances, considering a maximum localization error of 2 mm corresponding to the radius of stimulation of the electrodes, were similar to those presented in the literature and showed high agreement with clinical outcomes expressed by clinical evaluation scales. Similar validation was achieved in a second study Citation[14] in which the same “atlas-to-patient” technique was applied to the localization of the red nucleus, a structure located medially, posteriorly and inferiorly to the STN, that is clearly visible on MRI. The major differences in the measurements obtained for both the STN and red nucleus were in the inferior-superior direction. Improved localization can be achieved by using high-field MRI, as demonstrated in a recent article Citation[15].
Radiosurgical technique
The aim of those authors who have reported their experience with SRS for movement disorders was to achieve a therapeutic effect through the induction of a selective radio-necrotic lesion limited to a specific population of neurons volumetrically contained within the selected target. While the vast majority of functional radiosurgeries have been performed using the frame-based Gamma Knife system (Elekta AB, Stockholm, Sweden), the use of the CyberKnife frameless image guided robotic radiosurgery system (Accuray, Inc., Sunnyvale, CA) has also been suggested Citation[16].
High prescribed doses (greater than 120 Gy) are required to achieve the desired radiobiological effect, but the exact mechanism by which radiation produces a clinical effect in functional radiosurgery is still controversial. Kondziolka et al. Citation[17] recently hypothesized that irradiation spreading beyond the 50% isodose line (which is supposed to lose its necrotic effect on neural tissue) in patients undergoing thalamotomy for tremor might still affect surrounding kinesthetic cells without destroying them. On the other hand, Ohye et al. Citation[18] demonstrated in a very elegant way that a clinical effect requires neuronal necrosis and that the necrotic margins are very sharp, conforming to the 50% isodose line. Using depth microrecording in two patients who underwent SRS thalamotomy with an unsatisfactory result, they showed a lack of neuronal activity and the presence of the rhythmic discharge of tremor cells within and immediately outside the target, respectively. This observation is of increased importance because it shows the high spatial selectivity of radiosurgery. On the basis of this experience, the author modified his treatment planning for successive treatments, expanding the target coverage with the aim of completely covering the region of tremor cells.
Ohye and colleagues have also published an interesting analysis of the outcome of functional radiosurgery for movement disorders, assessing the extent and location of the lesion on MRI, as well as its clinical effect Citation[19]. In essence, two different patterns of radio-induced brain tissue change were identified: a well-circumscribed lesion with peripheral contrast enhancement surrounding a low-signal center, and an irregularly shaped area of high signal extending out from the target. The latter type has been correlated with a higher risk of extensive vasogenic edema, which accounts for most of the post-therapeutic side effects of SRS. An influence of the dose rate delivered to the target could be hypothesized on the basis of the observation that better outcomes were observed in patients treated after reloading the cobalt source. However, a genetic hypersensitivity to radiation could also explain more extensive radio-induced MR changes and consequent side effects.
It may take up to one year to achieve a clinical effect with functional radiosurgery; early results can be seen one month after treatment, but the full therapeutic effect is usually delayed until 6 months or longer post-irradiation. Radio-induced edema does not appear until at least 3 months after the procedure, and can last up to 1 year, requiring substantial amounts of steroids for clinical management. Permanent neurological complications have also been described following functional radiosurgery Citation[20], Citation[21]. The delay before clinical efficacy and the complications may be seen as substantial drawbacks of this treatment modality.
In general, the risk of complications in radiosurgery applications correlates with the volume of normal tissue within, or immediately adjacent to, the prescribed isodose surface Citation[22]. To estimate this volume, several indices have been proposed, such as the conformity (CI) and new conformity (nCI) indices Citation[23], Citation[24]. Nakamura et al. Citation[25] evaluated the CI and nCI values of the planned dose distributions delivered to an increased number of targets and tried to associate them with the risk of complications. They found that serious complications (ranging from mild side effects to those requiring corticosteroids or surgical resection for radionecrosis to mortality) were associated with higher CIs and nCIs; however, no correlation was observed when the treated lesions were smaller than 1 ml, as with the targets used in functional radiosurgery. Besides the small volume of the irradiated targets, which are surrounded by healthy tissue, functional radiosurgery is also characterized by large prescribed doses. Therefore, treatment plan distributions with very high spatial dose gradients should be used to minimize the dose to the surrounding healthy neural tissue and thus avoid side effects and complications. Since the spatial dose gradient depends on the collimator size, the smallest available collimator (i.e., the 4-mm collimator for the Gamma Knife and the 5-mm collimator for the CyberKnife) should be used for treatment planning. Furthermore, the adjacent sensitive structures should also be identified and spared during treatment planning calculations Citation[26]. To this end, non-isocentric beams may have an advantage over the classically isocentric beam configuration used during treatment planning.
Clinical experience in radiosurgery for movement disorders
The first reported experiences with the use of SRS for the treatment of tremor were published in the early 1990s by Lindquist et al. Citation[27] in Europe, Rand et al. Citation[28] in the US, and Ohye et al. Citation[29] in Japan. Since these initial reports, there have been several other retrospective series on the placement of stereotactic lesions in the VIM, GPi and STN. To date, however, there have been no randomized trials or comparisons with more conventional approaches such as radiofrequency (RF) ablation. provides a summary of publications reporting SRS for movement disorders and the following sub-sections summarize those reports with respect to each chosen target.
Table I. Series of SRS for movement disorders.
Thalamotomy
Radiosurgical ablation of the VIM nucleus for the treatment of tremor in patients with PD, essential tremor (ET) or multiple sclerosis is, to date, the most widely reported procedure in functional radiosurgery. Differences in target location, patient selection, pre- and post-radiosurgery evaluation (including quantification of disability using specific testing), modalities of follow-up, inaccuracies in reporting scores, and/or selection bias may all affect the reports from different groups. In all thalamotomy series reported so far, the target was the VIM contralateral to the dominant side or contralateral to the most affected side, with the series by Ohye and colleagues also partially covering the nucleus ventralis oralis (VO) in PD patients also affected by rigidity Citation[18], Citation[19], Citation[28], Citation[29].
The first series outlining the feasibility of SRS in thalamotomy for tremor was published by Duma and coworkers Citation[31]. This paper reported on the radiosurgical treatment of 34 patients with PD-related tremor. Follow-up extended to 5 years, and clinical assessments based on the Unified Parkinson's Disease Rating Scale (UPDRS) were provided to quantify the post-treatment improvement in tremor score. Overall, the authors reported clinical efficacy of the treatment in 89% of the patients. A complete disappearance of tremor or excellent relief occurred in 24% and 26% of the patients, respectively; and a good effect (considered to be an improvement of between 33% and 66% in UPDRS scores) was observed in 29%. A mild improvement was found in 10.5% of the patients, while no improvement was seen in 10.5% of the patients. The authors also performed an interesting comparison between high and low delivered doses (average maximum dose of 160 Gy and 120 Gy, respectively), and found a statistical association between good outcomes (such as good, excellent, and complete relief of tremor) and higher doses.
The largest series of SRS thalamotomies to date is that reported by Young and colleagues Citation[32]. The authors analyzed and described in detail the results with 158 patients treated by Gamma Knife radiosurgery for tremor due to PD, ET or another pathological entity. The assessment at follow-up was executed by an independent group of movement disorder specialists, using conventional scale systems including the UPDRS and the Fahn-Tolosa-Martin Clinical Rating Scale for Tremor. The profile between efficacy and side effect was clearly favorable: 76.5% and 11.8% of the treated patients achieved a free or nearly free tremor condition, respectively, while 11.8% experienced poor or no improvement in tremor reduction. A modest rate of permanent neurological complications was reported (1.3%). Similar results were presented in an earlier publication by the same author Citation[33], with significant clinical improvements achieved in 88.9% of the patients treated (24 of 27).
Another series of SRS thalamotomies for tremor from Ohye et al. in 2005 Citation[30] re-evaluated the group's experience since 1993 as partially published in previous papers Citation[19], Citation[20], Citation[29]. The principal aim was to assess the effects of Gamma Knife thalamotomy on tremor before and after reloading of the radioactive cobalt source. From their entire experience with 94 radiosurgically treated patients with movement disorders, they analyzed a subgroup of 51 patients, including 35 with PD and 11 with ET, the rest being affected by various neurological diseases including secondary tremor. An extensive analysis of the neurological outcomes was not performed, but the authors state that the treatment was satisfactory in around 80% of the cases treated. The analysis focused on the time relationship between signal changes on the patients’ follow-up MR images, the size of the lesions, and the clinical response. These results were consistent with those presented earlier Citation[19], Citation[29], where major clinical improvements (clinical reduction of the tremor to less than 25% of the preoperative level) were found in 80% of the cases treated.
The most recent series comes from the Pittsburgh group Citation[17], who analyzed 26 patients with ET alone, excluding those already published in 2000 Citation[34] with MS-related tremor. The results obtained with this cohort showed an improvement in action tremor and writing score on the Fahn-Tolosa-Marin tremor score scale in 69% of patients. Fifty percent of the patients experienced complete or almost complete disappearance of tremor, while an improvement was found in 90% of cases. Comparable data were reported in the earlier paper from the same authors Citation[34] and by Young et al. Citation[33].
Pallidotomy
The internal globus pallidus (GPi) is a commonly used target for treating dystonias that was also popular for the treatment of PD prior to the introduction of subthalamic surgery. Experience with pallidotomy based on RF ablation has been extensively reported in the literature. This procedure was later replaced by GPi DBS. Radiosurgical GPi ablation has also been reported, but on a much smaller scale. Rand et al. Citation[28] and Friedman et al. Citation[35] were the first groups to present a series of patients (a total of 8 and 4 patients, respectively) treated with Gamma Knife pallidotomy. In both cases, the treated patients experienced an improvement in their dyskinesia scores. A high incidence of side effects was also reported. According to Friedman et al., improved results have been achieved in the most recent patients Citation[36].
Subsequently, Young et al. Citation[33] presented a detailed analysis of patients treated with radiosurgical pallidotomy together with some thalamotomy, followed by an analysis comparing their series of RF GPi lesions Citation[37]. In the later report, 29 patients with PD-related bradykinesia, rigidity, and L-DOPA-induced dyskinesias underwent SRS pallidotomy. More than the 80% of the patients achieved a significant improvement in dyskinesia, and two-thirds had improvements in bradykinesia or rigidity.
Subthalamotomy
The subthalamic nucleus is the region most commonly used today in functional neurosurgery. Bilateral DBS implantation is considered a state-of-the-art treatment for end-stage PD patients developing levodopa-related complications (freezing, on-off phenomena, and dyskinesias) and for those affected by the cardinal symptoms of PD (tremor, rigidity, and akinesia). While there is extensive experimental evidence that lesioning of the STN induces significant symptomatic relief, so far only one case report has been published involving the radiosurgical ablation of this target. This report illustrates the case of an elderly patient with severe PD-related motor symptoms (motor fluctuations, dyskinesia and bradykinesia) who had been previously treated with RF ablation of the contralateral STN. The subsequent radiosurgical procedure was successful, resulting in the disappearance of bradykinesia and rigidity, with persistence of minimal focal (oral) dyskinesias Citation[38].
Representative case
Our group has recently treated a patient suffering from a painful hemi-dystonia induced by brain ischemia related to a cardiac surgery procedure. The dystonia devoloped a few months after recovery from the acute event. The patient, who was on anticoagulants and presented with many other severe co-morbidities, was evaluated preoperatively using the Burke-Fahn-Marsden Dystonia Rating Scale (BFMRS) for motor function and the visual analogic scale (VAS) for pain. A frameless radiosurgical pallidotomy was performed using the CyberKnife system Citation[39]. Axial CT images (0.53 mm in-plane and 1-mm slice thickness) were acquired and used for treatment planning and target tracking during treatment delivery. High-resolution T1- and T2-weighted MRI images (1 mm isotropical voxel) were acquired using a 1.5-T MAGNETOM Avanto scanner (Siemens AG, Erlangen, Germany) and used for delineation of the target and critical structures.
The GPi was selected as the target and was spatially defined on the patient's MRI by an expert functional neurosurgeon, aided by atlas-based computerized identification Citation[9]. Treatment planning was performed using the MultiPlan® v.2.0.5 treatment planning system (TPS). The critical structure of the optic tract was delineated and a dose limit of 15 Gy was assigned during treatment planning optimization Citation[16]. Additional artificial critical structures were delineated to increase the spatial dose gradient of the created dose distribution. Two treatment plans were generated and compared; one using the 5-mm collimator (defined at the nominal source-to-axis distance [SAD] of 800 mm, which is typically used for intracranial applications) combined with the head path, and one using the 7.5-mm collimator combined with the trigeminal path of the system (the SAD for this path is 650 mm and the field size is therefore equal to 6.1 mm) Citation[39]. The second treatment plan with the slightly larger field size, which consisted of 164 non-isocentric beams (), was selected in an effort to minimize treatment time (102 minutes). The spatial dose gradient for the selected treatment plan was up to 32 Gy per mm, 24 Gy per mm and 15 Gy per mm in the left-right, superior-inferior and anterior-posterior directions, respectively (see also ). A dose of 120 Gy was prescribed to the 65% isodose line, resulting in a total of 35,423 Monitor Units (MUs). General anesthesia was used because the patient was in such severe pain that they could not remain still during the treatment. The treatment began, as usual, without anesthesia, but from the outset the patient, who was placed in a fixed position on the treatment table, developed severe spasm and pain. The head movements caused by the patient's discomfort prevented accurate targeting and we were forced to resort to general anesthesia. In essence, general anesthesia was used in this case as a last resort and only after failure of conservative measure (such as extensive padding, administration of spasmolytic and analgesic drugs, mild i.v. sedation with remyfentanil) with the aim of preserving the accuracy of beam delivery. Only by using this approach could inaccurate delivery caused by head movements and prolonged treatment time due to frequent checks of the head position (which would have further increased inaccuracy) be avoided in this case. presents the translations and rotations of the skull of the patient during treatment delivery; translations of up to 3 mm and rotations up to 1° can be observed. It should be noted that these values were fed into the robotic manipulator to realign the corresponding therapeutic beams and thereby correct for any target movements during treatment delivery.
Figure 1. Direct identification of subthalamic nucleus (STN) on T2 TSE coronal 3-T MR images (A and B) and of the nucleus ventralis intermedius (VIM) on TSE dual-echo axial DP 3-T MR images (C and D).
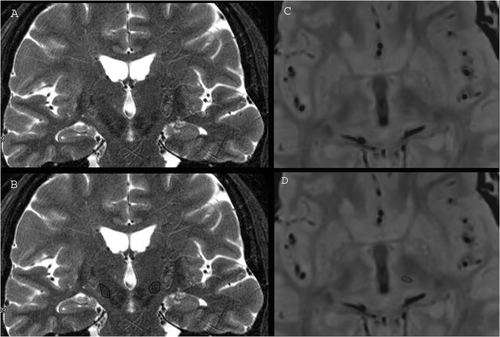
Figure 2. The 3D beam distribution (upper left) and the axial (upper right), sagittal (lower left) and coronal (lower right) views of the pallidotomy case treatment plan visualized on the patient T2-weighted MRI volume.
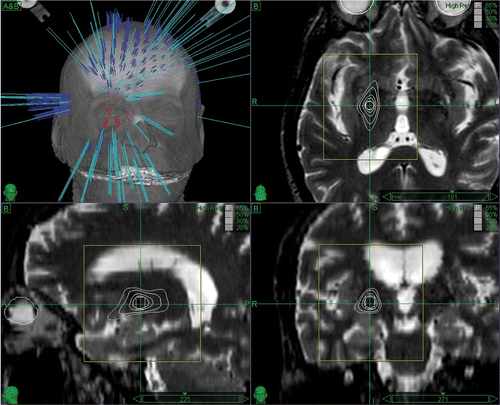
Figure 3. Translations and rotations of the skull of the pallidotomy patient during treatment delivery. These values were fed into the system to realign the therapeutic beam and aim at the target during treatment delivery.
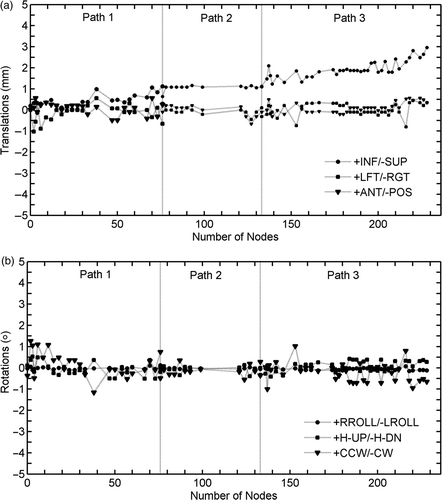
presents the T2-weighted MRI images of the patient acquired 6 months post-treatment. An excellent placement of the radio-induced lesion can be observed, albeit surrounded by vasogenic edema causing contralateral motor weakness that required prolonged steroid coverage Citation[16]. The planned dose distribution and the dose gradients of the created treatment plan are also overlaid, showing a fine spatial coincidence with the radio-induced lesion, and serving as a qualitative measure of the clinical accuracy of the treatment. The dystonia BFM motor subscore improved by an average of 86% and the BFM disability subscore by 80% at 6 months, while related pain moved from a mean VAS value of 8 to 0. Medical therapy to manage the dystonic spasms and pain was completely suspended 12 months after treatment.
Figure 4. T2-weighted MRI axial (upper left), sagittal (upper right) and coronal (bottom) slices of the pallidotomy patient acquired 6 months post-treatment. The planned dose distribution (solid black lines; starting from the inside, these are the 120 Gy, 80 Gy and 40 Gy isodose lines) and the dose gradients (arrows) are also superimposed, allowing for a qualitative estimation of the clinical accuracy and related dose gradients of the treatment.
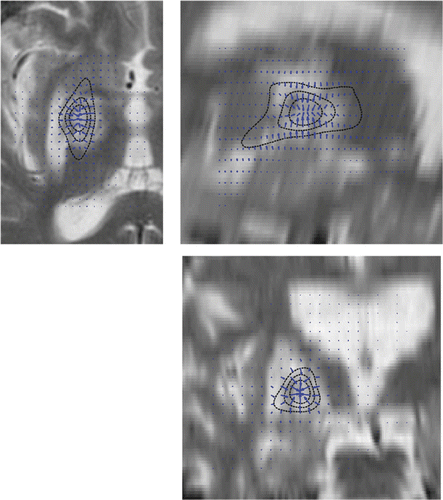
Conclusions
Stereotactic radiosurgery is an image guided non-invasive technique that can be used to create focal brain lesions. The lack of neurophysiological guidance and the irreversibility of the generated lesion are strong arguments against the use of radiosurgery to treat movement disorders. On the other hand, state-of–the-art neuroimaging and implementation of target localization using newly developed techniques of image analysis that support refined atlas-based target localization can substantially increase the safety of these procedures, providing a new option for patients who are unfit for open surgery.
References
- Leksell L. The stereotaxic method and radiosurgery of the brain. Acta Chir Scand 1951; 102: 316–319
- Cooper IS. Ligation of the anterior choroidal artery for involuntary movements of parkinsonism. Psychiat Quart 1953; 27: 317–319
- Rezai AR, Kopell BH, Gross RE, Vitek JL, Sharan AD, Limousin P, Benabid AL. Deep brain stimulation for Parkinson's disease: Surgical issues. Mov Disord 2006; 21(Suppl 14)S197–S218
- Zhu XL, Hamel W, Schrader B, Weinert D, Hedderich J, Herzog J, Volkmann J, Deuschl G, Müller D, Mehdorn HM. Magnetic resonance imaging-based morphometry and landmark correlation of basal ganglia nuclei. Acta Neurochir 2002; 144(10)959–969
- Spiegelmann R, Nissim O, Daniels D, Ocherashvilli A, Mardor Y. Stereotactic targeting of the ventrointermediate nucleus of the thalamus by direct visualization with high-field MRI. Stereotact Funct Neurosurg 2006; 84(1)19–23
- Slavin KV, Thulborn KR, Wess C, Nersesyan H. Direct visualization of the human subthalamic nucleus with 3T MR imaging. Am J Neuroradiol 2006; 27: 80–84
- Rampini PM, Locatelli M, Alimehmeti R, Tamma F, Caputo E, Priori A, Pesenti A, Rohr M, Egidi M. Multiple sequential image fusion and direct MRI localisation of the subthalamic nucleus for deep brain stimulation. J Neurosurg Sci 2003; 47: 33–39
- Cho ZH, Min HK, Oh SH, Han JY, Park CW, Chi JG, Kim YB, Paek SH, Lozano AM, Lee KH. Direct visualization of deep brain stimulation targets in Parkinson disease with the use of 7-tesla magnetic resonance imaging. J Neurosurg 2010; 113: 639–647
- Stancanello J, Romanelli P, Modugno N, Cerveri P, Ferrigno G, Uggeri F, Cantore G. Atlas-based identification of targets for functional radiosurgery. Med Phys 2006; 33(6)1603–1611
- Yelnik J, Damier P, Demeret S, Gervais D, Bardinet E, Bejjani BP, Francois C, Houeto JL, Arnule I, Dormont D, et al. Localization of stimulating electrodes in patients with Parkinson disease by using a three-dimensional atlas magnetic resonance imaging coregistration method. J Neurosurg 2003; 99: 89–99
- Atkinson JD, Collins DL, Bertrand G, Peters TM, Pike GB, Sadikot AF. Optimal location of thalamotomy lesions for tremor associated with Parkinson disease: A probabilistic analysis based on postoperative magnetic resonance imaging and an integrated digital atlas. J Neurosurg 2002; 96: 854–866
- Starr PA, Vitek JL, DeLong M, Bakay RA. Magnetic resonance imaging-based stereotactic localization of the globus pallidus and subthalamic nucleus. Neurosurgery 1999; 44: 303–314
- St-Jean P, Sadikot AF, Collins L, Clonda D, Kasrai R, Evans AC, Peters TM. Automated atlas integration and interactive three-dimensional visualization tools for planning and guidance in functional neurosurgery. IEEE Trans Med Imaging 1998; 17: 672–680
- Stancanello J, Romanelli P, Sebastiano F, Modugno N, Muacevic A, Cerveri P, Esposito V, Ferrigno G, Uggeri F, Cantore G. Direct validation of atlas-based red nucleus identification for functional radiosurgery. Med Phys 2007; 34(8)3143–3148
- Stancanello J, Muacevic A, Sebastiano F, Modugno N, Cerveri P, Ferrigno G, Uggeri F, Romanelli P. 3T MRI evaluation of the accuracy of atlas-based subthalamic nucleus identification. Med Phys 2008; 35(7)3069–3077
- Stancanello J, Romanelli P, Pantelis E, Sebastiano F, Modugno N. Atlas-based functional radiosurgery: Early results. Med Phys 2009; 36(2)457–463
- Kondziolka D, Ong JG, Lee JY, Moore RY, Flickinger JC, Lunsford LD. Gamma Knife thalamotomy for essential tremor. J Neurosurg 2008; 108(1)111–117
- Ohye C, Shibazaki T, Zhang J, Andou Y. Thalamic lesion produced by gamma thalamotomy for movement disorders. J Neurosurg 2002; 97(5 Suppl)600–606
- Ohye C, Shibazaki T, Hirato H, Inoue H, Andou Y. Gamma thalamotomy for Parkinsonian and other kinds of tremor. Stereotact Funct Neurosurg 1996; 66: 333–342
- Siderowf A, Gollump SM, Stern MB, Baltuch GH, Riina HA. Emergence of complex, involuntary movements after gamma knife radiosurgery for essential tremor. Mov Disord 2001; 16: 965–967
- Bonnen JG, Iacono RP, Lulu B, Mohamed AS, Gonzalez A, Schoonenberg T. Gamma knife palidotomy: Case report. Acta Neurochir (Wien) 1997; 139(5)442–445
- Smith V, Verhey L, Serago CF. Comparison of radiosurgery treatment modalities based on complication and control probabilities. Int J Radiat Oncol Biol Phys 1998; 40(2)507–513
- Shaw E, Scott C, Souhami L, Dinapoli R, Kline R, Loeffler J, Farnan N. Single dose radiosurgical treatment of recurrent previously irradiated primary brain tumors and brain metastases: Final report of RTOG protocol 90-05. Int J Radiat Oncol Biol Phys 2000; 47: 291–298
- Paddick I. A simple scoring ratio to index the conformity of radiosurgical treatment plans. J Neurosurg 2003; 93(3)219–222
- Nakamura JL, Verhey LJ, Smith V, Petti PL, Lamborn KR, Larson DA, Wara WM, McDermott MW, Sneed PK. Dose conformity of gamma knife radiosurgery and risk factors for complications. Int J Radiat Oncol Biol Phys 2001; 51: 1313–1319
- Pantelis E, Papadakis N, Verigos K, Stathochristopoulou I, Antypas C, Lekas L, Tzouras A, Georgiou A, Salvaras N. Integration of functional MRI and white matter tractography in stereotactic radiosurgery clinical practice. Int J Radiat Oncol Biol Phys 2010; 78(1)257–267
- Lindquist C, Kihlström L, Hellstrand E. Functional neurosurgery — a future for the gamma knife?. Stereotact Funct Neurosurg 1991; 57: 72–81
- Rand RW, Jacques DB, Melbye RW, Copcutt BG, Fisher MR, Levenick MN. Gamma Knife thalamotomy and pallidotomy in patients with movement disorders: Preliminary results. Stereotact Funct Neurosurg 1993; 61(Suppl 1)65–92
- Ohye C, Shibazaki T, Ishihara J, Zhang J. Evaluation of gamma thalamotomy for parkinsonian and other tremors: Survival of neurons adjacent to the thalamic lesion after gamma thalamotomy. J Neurosurg 2000; 93(Suppl 3)120–127
- Ohye C, Shibazaki T, Sato S. Gamma knife thalamotomy for movement disorders: Evaluation of the thalamic lesion and clinical results. J Neurosurg 2005; 102(Suppl)234–240
- Duma CM, Jacques DB, Kopyov OV, Mark RJ, Copcutt B, Farokhi HK. Gamma knife radiosurgery for thalamotomy in Parkinsonian tremor: 5-year experience. J Neurosurg 1998; 88: 1044–1049
- Young RF, Jacques S, Mark R, Kopyov O, Copcutt B, Posewitz A, Li F. Gamma knife thalamotomy for treatment of tremor: Long term results. J Neurosurg 2000; 93(Suppl 3)128–135
- Young RF, Shumway-Cook A, Vermeulen SS, Grimm P, Blasko J, Posewitz A, Burkhart WA, Goiney RC. Gamma knife radiosurgery as a lesioning technique in movement disorder surgery. J Neurosurg 1998; 89(2)183–193
- Niranjan A, Kondziolka D, Baser S, Heyman R, Lunsford LD. Functional outcomes after gamma knife thalamotomy for essential tremor and MS-related tremor. Neurology 2000; 55: 443–446
- Friedman JH, Epstein M, Sanes JN, Lieberman P, Cullen K, Lindquist C, Daamen M. Gamma knife pallidotomy in advanced Parkinson's disease. Ann Neurol 1996; 39: 535–538
- Friedman DP, Goldman HW, Flanders AE, Gollomp SM, Curran WJ, Jr. Stereotactic radiosurgical pallidotomy and thalamotomy with the gamma knife: MR imaging findings with clinical correlation - preliminary experience. Radiology 1999; 212: 143–150
- Young RF, Vermeulen S, Posewitz A, Shumway-Cook A. Pallidotomy with the gamma knife: A positive experience. Stereotact Funct Neurosurg 1998; 70(Suppl 1)218–228
- Keep MF, Mastrofrancesco L, Erdman D, Murphy B, Ashby LS. Gamma knife subthalamotomy for Parkinson disease: The subthalamic nucleus as a new radiosurgical target. Case report. J Neurosurg 2002; 97(5 Suppl)592–599
- Antypas C, Pantelis E. Performance evaluation of a CyberKnife G4 image-guided robotic stereotactic radiosurgery system. Phys Med Biol 2008; 53: 4697–4718
- Otsuki T, Jokura H, Takahashi K, Ishikawa S, Yoshimoto T, Kimura M, Yoshida R, Miyazawa T. Stereotactic gamma-thalamotomy with a computerized brain atlas: Technical case report. Neurosurg 1994; 35(4)764–767
- Friehs GM, Ojakangas CL, Pachatz P, Schröttner O, Ott E, Pendl G. Thalamotomy and caudatotomy with the Gamma Knife as a treatment for parkinsonism with a comment on lesion sizes. Stereotact Funct Neurosurg 1995; 64(Suppl 1)209–221
- Pan L, Dai JZ, Wang BJ, Xu WM, Zhou LF, Chen XR. Stereotactic Gamma thalamotomy for the treatment of parkinsonism. Stereotact Funct Neurosurg 1996; 66(Suppl 1)329–32
- Okun MS, Stover NP, Subramanian T, Gearing M, Wainer BH, Holder CA, Watts RL, Juncos JL, Freeman A, Evatt ML, et al. Complications of gamma knife surgery for Parkinson disease. Arch Neurol 2001; 58(12)1995–2002
- Mathieu D, Kondziolka D, Niranjan A, Flickinger J, Lunsford LD. Gamma knife thalamotomy for multiple sclerosis tremor. Surg Neurol 2007; 68(4)394–399