Abstract
Computer navigation has recently been introduced for bone tumor surgery in the orthopedic field, with the aim of achieving increased accuracy and precision in tumor resection and in custom prosthetic reconstruction. However, the technique requires bulky navigation facilities, the presence of a system operator in the operating room, and surgeons with prior experience in navigated surgery. We describe a new and simple method of using a patient-specific computer-aided design/computer-aided modeling (CAD/CAM) surgical jig to realize the preoperative planning in the surgical field. The accuracy of the proposed method was first tested in a cadaver trial. It took one minute to set the location of the jig prior to the bone resection and three minutes to perform the bone resections via the cutting slits of the jig. The dimensional difference between the achieved and planned bone resection was <1 mm on validation with the help of a junctional plate and a navigation system. The technique was then applied successfully to a patient with a low-grade osteosarcoma of the femur. An intercalated tumor resection was performed using a patient-specific surgical jig, and a custom CAD prosthesis reconstruction matched accurately to the skeletal defect. Further assessment in a larger population is necessary to determine the clinical efficacy of the technique.
Introduction
In bone sarcoma surgery, one of the major challenges for the surgeon is to resect the bone tumor with an oncologically safe margin and to reconstruct the skeletal defect to the exact anatomical dimensions necessary for good function. The achieved orientation and plane of the bony resection are particularly crucial if a custom tumor prosthesis is chosen for the bony reconstruction. Very accurate placement of bone cuts that coincide with the precise dimensions of a prosthesis custom-designed to fit the specific bone defect can ensure good alignment and superior function in the extremity.
Computer navigation has recently been introduced for bone tumor surgery in the orthopedic field, with the aim of achieving increased accuracy and precision in tumor resection and reconstruction Citation[1–6]. The technology allows multimodal image fusion for surgical planning and provides excellent intraoperative guidance to help surgeons realize the preoperative planning during the actual operation. Early results have been encouraging, and this approach may be helpful for difficult resections in regions of complex anatomy, such as those of pelvic or sacral tumors, or in joint-preserving surgery where adjacent joints can be spared after tumor resection. However, the technique requires bulky navigation facilities (an optical camera, navigation trackers and instruments, a computer with a monitor, etc.), the presence of a system operator in the operating room, and surgeons with prior experience in navigated surgery.
Surgical guides have already been used to facilitate accurate positioning of various implants. Custom-made drill guides have been commercialized for dental implant surgery Citation[7], Citation[8], while in craniomaxillofacial surgery, computer-aided planning and a computer-aided design/computer-aided modeling (CAD/CAM) surgical jig is used to guide complex mandibular osteotomies and reconstruction with a free fibular graft Citation[9], Citation[10]. Attempts to use surgical CAD/CAM guides have recently been reported in the orthopedic fields of spinal instrumented surgery Citation[11], Citation[12] and total joint arthroplasty Citation[13], Citation[14], and in difficult osteotomies in deformity correction Citation[15], Citation[16], and this approach has been shown to be a viable method of realizing computer planning.
In this paper we describe a new method of using a CAD/CAM surgical jig to reproduce a virtually planned bone resection in a desired orientation that matches a custom prosthesis precisely. The use of a surgical jig was first evaluated in a cadaveric leg, and its accuracy was validated with the help of a navigation system. A joint-preserving intercalated tumor resection and CAD prosthesis reconstruction was then performed in a patient with a low-grade osteosarcoma of the femur with the aid of a CAD/CAM surgical jig.
Materials and methods
A cadaver leg was used to study the feasibility of the concept in extremity bone tumor surgery. The fresh frozen leg was that of a middle-aged man with a normal knee joint. Axial CT images of the femur were acquired using a 16-detector scanner (LightSpeed, GE, Milwaukee, WI). Slices of 0.625 mm thickness were obtained using a soft tissue algorithm. The CT images in DICOM format were imported into a medical image processing software (Mimics® 14.1, Materialise NV, Leuven, Belgium), which ran on an ordinary desktop PC.
Surgical planning
A gray value-based segmentation of the CT images excluded the soft tissues and generated a 3D reconstruction of the femoral bone for the surgical simulation. The surgeons then defined the proximal and distal planes of the bone resection (). These marked the limits of the bone segment that would be removed from the femur. Virtual resection allowed detailed examination and 3D volumetric description of the resected bone segment. This provided the electronic data for the design of the CAD/CAM surgical jig and an intercalated prosthesis to span the skeletal defect.
Figure 1. Virtual resection planning of the cadaveric femur in the CAD software. CT images in DICOM format were imported into the CAD software. Axial (B), reformatted coronal (A) and sagittal (C) images were generated. (D) The 3D femur was segmented by the thresholding process. Planes for the bone resection (proximal TP and distal TP) were defined. The planes were 1 mm in thickness, the same as that of the oscillating saw used for osteotomy in the cadaver surgery.
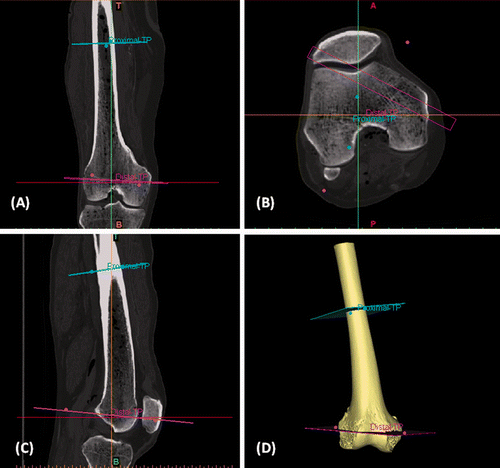
Design of a patient-specific CAD/CAM surgical jig
The femoral bone model and resection planes were exported as stereolithographic (STL) files and transferred to the rapid prototyping software (Magics RP, version 14, Materialise, Leuven, Belgium), which ran on the same computer as the Mimics software. The software allowed further editing and integration of external CAD models in STL format. A series of Boolean operations on the CAD models was conducted to combine different jig components and create the contact interface between the bone model and the jig. The design of the surgical jig () incorporated the following components:
Proximal and distal cutting blocks that could be positioned consistently on the bone surface at the planned resection sites without translation. The contour at the distal femoral condyle provided the necessary bone surface for the positioning.
Cutting slits that were based on the unique orientation of the resection planes defined during virtual simulation in the Mimics software. The slit was 1.2 mm in width so as to accommodate an oscillating saw blade with 1 mm thickness for making the osteotomy.
A built-in handle that connected the two cutting blocks and was directed anteromedially for easy surgical access.
Thermoplastic material (polyphenlysulfone) fabricated in a rapid prototyping machine (Fortus 400mc Fused Deposition Modeling (FDM) system, Stratasys Inc., Eden Prairie, MN), which has high chemical resistance and high tensile strength, and is heat stable up to 200°C and can be sterilized for use in the operating theater.
Figure 2. The 3D femur and planes of resection were exported as STL files that were then transferred to the rapid prototyping software. (A) A patient-specific CAD/CAM surgical jig was designed with cutting slits based on the exact orientation of the resection planes defined in the virtual planning. (B) The two cutting blocks were connected by a handle with an orientation that could facilitate positioning without obstructing the surgical exposure. (C) The undersurface of the surgical jig. (D) The cutting blocks had anatomical shapes that matched the bone surface at the defined resection level. This ensured easy and accurate positioning of the jig during the surgery.
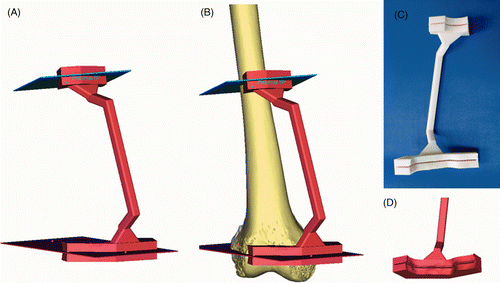
Ceramic femoral bone models for both the cadaver leg and the patient were also fabricated using a 3D printing machine (Zprinter 310, Z Corporation, Burlington, MA) for the trial positioning of the surgical jig on the bone surface. For the cadaver leg, an intercalary test prosthesis, made of the same thermoplastic material as the surgical jig, was manufactured to verify the fit with the skeletal defect following the bone resection. For the patient, a real titanium prosthesis was manufactured to reconstruct the skeletal defect following the tumor resection. To validate the accuracy of the planned bone resection using the patient-specific surgical jig, a thermoplastic CAD/CAM junctional plate, with the same cross-section as the distal femoral fragment remaining after the virtual bone resection, was designed and produced.
Navigation planning to assess the accuracy of the CAD/CAM surgical jig
The virtual CAD model of the test prosthesis was imported as STL files back into the Mimics software. The virtual CAD prosthesis was overlaid on the original CT images of the cadaveric femur, which were then exported in DICOM format. The modified DICOM files contained the information of the newly designed CAD prosthesis in addition to that of the original femur Citation[17]. The original CT images of the cadaveric femur and the virtually planned, modified DICOM datasets were transferred into a CT-based navigation system (Stryker Navigation System – OrthoMap 3D module, version 2.0, Stryker, Mahwah, NJ) for resection planning (). The navigation system provides automatic image fusion of the original and modified DICOM data sets, a segmentation tool for extracting a 3D CAD object, and also tools to define a planned bone resection.
Figure 3. Preoperative screen images of surgical navigation planning on the navigation monitor for the cadaver study. The original CT image datasets (CT01) were fused with a modified CT dataset (CT02) containing the virtual CAD model of the test prosthesis, and with another modified CT dataset (CT03) containing the proximal and distal resection planes. The virtual CAD prosthesis and resection planes could be extracted with the aid of the segmentation tools in the navigation software. Proximal and distal resection planes were then marked as planned in the CAD software. Axial (A), sagittal (B) and coronal (C) images and the reconstructed 3D planning bone model (D) illustrate the transfer of the CAD virtual planning to the navigation system by the method of CAD to DICOM conversion and image fusion.
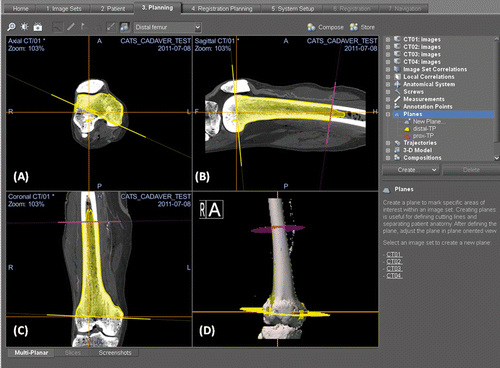
Surgery of a cadaver leg
A medial subvastus approach was adopted. The distal two-thirds of the femur was exposed, and a universal tracker was attached to the shaft of the femur at a location that would not hinder the subsequent positioning of the surgical jig. Image-to-bone registration was performed using a paired-point and surface-matching algorithm. The registration error was 0.53. We could rely on the accuracy of the navigation system when a real-time match between the virtual CT images and the cadaveric femur was obtained. The surgical jig was then set on the surface at the planned bone resection site (). The surgeon held the jig against the bone through manual pressure. Stable positioning of the jig was ensured when the femur was osteotomized via the cutting slits of the jig (). As the universal tracker was still attached to the resected bone and maintained its registration, the accuracy of the achieved bone resection was then assessed under computer navigation. Also, a junctional plate that matched to the cross-section of the resected bone ends in the distal femoral osteotomy during virtual simulation was used to measure the difference between the planned and achieved bone resection.
Figure 4. (A) After surgical exposure of the distal femur via a medial subvastus approach, the patient-specific CAD/CAM surgical jig was positioned at the planned resection sites. (B) The bone resection was performed with an oscillating saw through the cutting slits of the surgical template. (C) A junctional plate that matched the dimensions of the resected end during virtual simulation of the distal femoral osteotomy was compared with the distal femoral resection actually achieved. (D) The dimensional difference between the planned resection (represented by the junctional plate) and the achieved resection was <1 mm, and therefore validated the feasibility and accuracy of the technique.
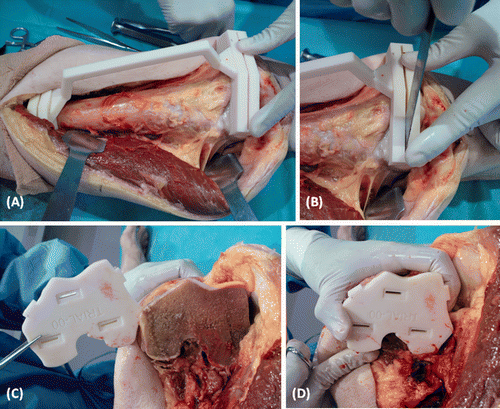
Results
The CAD/CAM surgical jig could be fitted snugly onto the bone surface at the planned resection sites. It took one minute to set the location of the jig prior to the bone resection and three minutes to make the bone resections via the cutting slits of the jig. The direction of the oscillating saw was well guided by the cutting slits and the guided bone resection could easily be performed without extra adjustment. The junctional plate also matched well to the distal bone end, with <1 mm difference in dimensions (). On assessing the accuracy of the achieved bone resection with the help of the navigation system, the difference between the planned and achieved bone resection was found to be <1 mm for the four quadrants of the resected bone ends (). The concept and the workflow were thus validated, and the technique was applied to a patient with primary bone sarcoma.
Figure 5. The accuracy of the technique was also validated with the help of a CT-based navigation system. (A) After bone resection with the aid of the surgical jig, the resected bone segment with the attached reference tracker still retained the image-to-bone registration and was used for the validation. When the navigation pointer was in contact with the resected distal bone end, the virtual tip of the pointer (the orange cross) was compared with the planned resection plane (shown in yellow) on the navigation display. The coronal (B) and sagittal (C) images and the 3D bone model (D) showed the achieved resection to differ by <1 mm from the planned resection. This suggested that the technique could help execute the preoperative planning accurately in the surgical field.
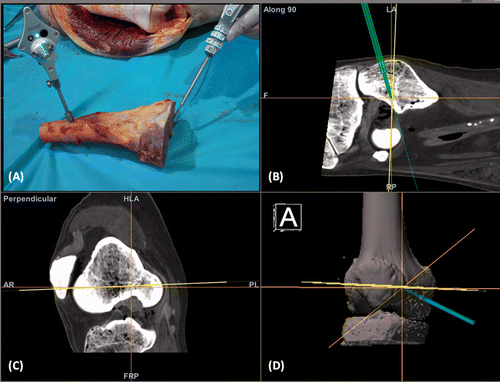
Case report
A 46-year-old man was diagnosed with a low-grade osteosarcoma of the left distal femoral metaphysis (). CT and MR scans of the left femur were performed. The bone resection levels were determined on the MR images that better showed the actual extent of the tumor. CT images in DICOM format were imported into the Mimics software for surgical simulation and definition of the planned resection planes (). The working files of the surgical simulation were forwarded to an implant manufacturing company (Stanmore Implants, Elstree, UK) to allow production of a custom CAD intercalary tumor prosthesis to reconstruct the skeletal defect.
Figure 6. (A) A coronal T1-weighted MR image of a patient with a low-grade osteosarcoma of the left femur (red arrows). (B) CT images of the femur were imported into the CAD software. The surgeon defined the proximal and distal bone resections that determined the design of a CAD/CAM surgical jig. (C) The matching contour surface at the distal resection site (the green region represents the actual bone contact area). (D) A custom CAD intercalary prosthesis was designed to match exactly the bone defect after resection. Extracortical plates were added to improve the initial stability of fixation for the reconstruction.
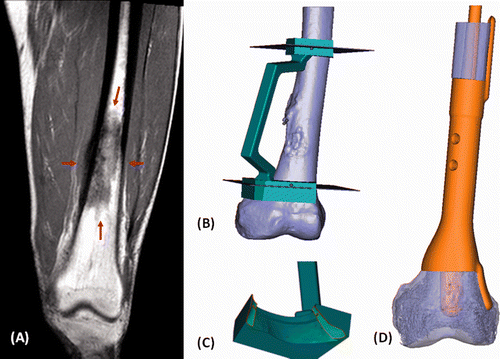
The prosthetic design comprised two assembled components with bone-implant junction dimensions that matched exactly the skeletal defect during virtual bone tumor resection (). Extracortical plates and screws were included for additional stability of the reconstruction. Hydroxyapatite (HA) coating was applied to the bone-implant junction to facilitate osseous integration with the host bone.
On the basis of the preoperative surgical simulation in the Mimics software, a CAD/CAM surgical jig to realize the planned bone resections was designed and manufactured using a rapid prototyping technology (). A junctional plate was also designed to assess the accuracy of the achieved distal resection during the surgery and help guide the rotational alignment of the distal component ().
Figure 7. (A) A junctional plate was designed to validate the accuracy of the planned resection. The central slot of the plate guided the medullary position of the distal prosthetic stem. The planned location of the extracortical plate could also be marked with the junctional plate and thus helped to position the prosthesis in the correct rotational alignment. (B) The junctional plate matched well with the achieved bone resection (black arrow). (C) The distal prosthetic component fitted perfectly to the bone resection (black arrow). A cable wire was applied medially to further enhance the initial stability of the reconstruction. Cancellous bone graft was added at the bone-prosthesis junction. At two months after surgery, a plain radiograph (D) showed satisfactory alignment of the prosthesis and the patient could achieve full knee flexion (E).
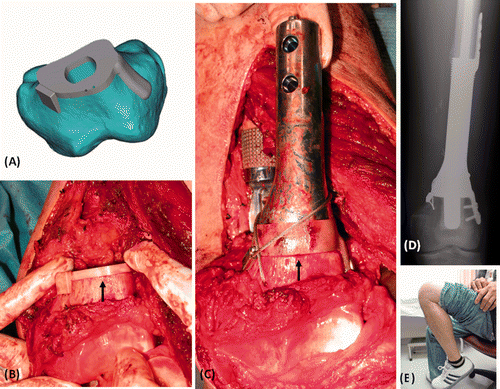
A medial subvastus approach to the femur was used. Femoral vessels were identified and protected, and the tumor was dissected from the surrounding soft tissue. The surgical jig showed excellent passive fit on the femoral bone surface contours and no adjustment was necessary. It took one minute to set the location of the jig and two minutes to make the two bone resections via the cutting slits of the jig. The junctional plate matched to the bone end of the distal resection with <1 mm difference in dimensions, thus validating the accuracy of the achieved bone resection (). The prosthesis was then inserted with cemented stems and titanium screws in the extracortical plates on the distal remaining femur (). Bone graft was added to the HA collar to enhance bone formation at the junction. A postoperative plain radiograph showed satisfactory prosthesis alignment (). No postoperative wound infection was noted, and the patient could achieve full knee flexion at two months after surgery ().
Discussion
CT and MR images are necessary for preoperative planning of complex musculoskeletal bone tumor resection and reconstruction. Tumor surgeons must mentally integrate the information in 2D format into a 3D model for surgical planning. The resection is technically demanding as it is necessary to ensure that the resection margin is oncologically acceptable, and that the orientation of the resection plane precisely matches the custom prosthesis.
Accurate tumor resection and placement of implants as planned are of critical importance to a good outcome in bone tumor surgery. However, such accurate execution of surgical planning may not be always possible even in experienced hands. Cartiaux et al. Citation[18] reported on the surgical accuracy of an experienced surgeon in performing a pelvic tumor resection with a planned 1 cm surgical margin. The surgeon could achieve a 1 cm surgical margin (±5 mm) with a probability of only 52%.
In this study, we used a patient-specific CAD/CAM surgical jig to realize the preoperative planning in bone tumor surgery. We developed and tested the accuracy of the proposed workflow in a cadaver trial, and then applied the new technique to perform an intercalated tumor resection and a custom CAD prosthesis reconstruction in a patient with a low-grade osteosarcoma of the femur.
In both the cadaver trial and the clinical case, CAD/CAM software enabled us to define precisely and perform virtual bone resection, and also generated the patient-specific surgical jig that could restore directly the preoperative surgical planning in the operating room. The difference of <1 mm in dimensions between the planned and achieved bone resection suggested that the workflow was practical. The achieved accuracy was comparable to that obtained when operating under computer navigation Citation[3], Citation[5]. However, the time required to perform the precise bone resection was only a few minutes – much shorter than the mean time of 24–28 minutes reported for navigation-guided approaches Citation[5], Citation[6]. Therefore, intraoperative use of the surgical jig generated from the virtual surgical planning might facilitate and expedite bone resection along a desired orientation, resulting in a precise CAD prosthesis reconstruction performed in less time.
In contrast to the bone tumor surgery performed under computer navigation, the operating time was greatly reduced as there was no need to set up the navigation system, perform image-to-patient registration, or mark the resection level under navigation guidance. The bone resection level was determined once the surgical jig was firmly fitted onto the bone surface at the planned resection sites. The surgical jig had the corresponding anatomical shape of the bone surface that enabled easy physical registration of the surgical planning to the patient's anatomy. The patient-specific CAD/CAM surgical jig may thus be regarded as a communication tool to transfer the virtual planning to the surgical field. Also, the cutting slits of the jig helped confine the oscillating saw to the planned plane of resection. This increases safety as the surgeon can concentrate his attention on the operative field rather than looking at the virtual images on the navigation display while performing the osteotomy. summarizes the differences between the navigation-guided and surgical jig-guided approaches in bone tumor surgery.
Table I. Comparison between navigation-guided and surgical jig-guided approaches for bone tumor surgery.
In bone sarcoma surgery, the CAD/CAM jig-guided approach is expected to have planning errors similar to those in the navigation-guided approach Citation[6], because both approaches have adopted the same workflow in the preoperative planning phase. For dental implant placement, one area of concern during the surgery is the ability to position the surgical jig in the jaws exactly as planned and also to reproduce the same position each time the jig is used Citation[19], Citation[20]. We did not encounter a similar problem, and the surgical jig could easily be fitted onto the femoral bone surface. The positioning of the jig had already been tried and tested on the physical bone model that was manufactured from the patient's CT data prior to the actual surgery. This easy fitting between the jig and bone surface may be attributed to the irregular curvatures of the distal femur. Further studies are needed to investigate how much bone contact surface is sufficient for consistent positioning of the jig, and whether the jig can also be applied to complex regions such as the pelvis or sacrum in bone tumor surgery.
In conclusion, the technique presented in this paper may provide a simple, all-in-one solution for bone tumor resection and custom prosthesis reconstruction. Virtual surgical simulation performed in CAD software can be transferred to the surgical field with the aid of a patient-specific CAD/CAM surgical jig. Surgical jig-guided bone resection may help reduce the operating time and attain the level of accuracy and precision that are crucial to success in bone sarcoma surgery. Further studies with a larger sample size are required to confirm the clinical efficacy of this new technique which has great potential.
Acknowledgments
We thank Mr Sudha Shunmugam (biomedical engineer), the design team, and Dr Paul Unwin (Stanmore Implants, Elstree, UK) for the design and manufacture of the custom CAD prosthesis. We acknowledge the great assistance of Mr Lawrence Yu and Mr Rock Hu (Bio-Medical Engineering, Materialise China) in using the Mimics software. We also thank Mr. Watson Chan (Navigation product manager, Stryker China Limited) for operating the navigation system in the cadaver study.
Declaration of interest: The authors report no declarations of interest. The Stryker, Materialise and Stanmore Implants companies did not fund or sponsor this research in any way.
Reference
- Cho HS, Oh JH, Han I, Kim HS. Joint-preserving limb salvage surgery under navigation guidance. J Surg Oncol 2009; 100(3)227–232
- Reijnders K, Coppes MH, van Hulzen AL, Gravendeel JP, van Ginkel RJ, Hoekstra HJ. Image guided surgery: New technology for surgery of soft tissue and bone sarcomas. Eur J Surg Oncol 2007; 33(3)390–398
- So TY, Lam YL, Mak KL. Computer-assisted navigation in bone tumor surgery: Seamless workflow model and evolution of technique. Clin Orthop Relat Res 2010; 468(11)2985–2991
- Wong KC, Kumta SM, Chiu KH, Cheung KW, Leung KS, Unwin P, Wong MC. Computer assisted pelvic tumor resection and reconstruction with a custom-made prosthesis using an innovative adaptation and its validation. Comput-Aided Surg 2007; 12(4)225–232
- Wong KC, Kumta SM, Chiu KH, Antonio GE, Unwin P, Leung KS. Precision tumour resection and reconstruction using image-guided computer navigation. J Bone Joint Surg Br 2007; 89(7)943–947
- Wong KC, Kumta SM, Antonio GE, Tse LF. Image fusion for computer-assisted bone tumor surgery. Clin Orthop Relat Res 2008; 466(10)2533–2541
- Ersoy AE, Turkyilmaz I, Ozan O, McGlumphy EA. Reliability of implant placement with stereolithographic surgical guides generated from computed tomography: Clinical data from 94 implants. J Periodontol 2008; 79(8)1339–1345
- Vercruyssen M, Jacobs R, Van Assche N, van Steenberghe D. The use of CT scan based planning for oral rehabilitation by means of implants and its transfer to the surgical field: A critical review on accuracy. J Oral Rehabil 2008; 35(6)454–474
- Roser SM, Ramachandra S, Blair H, Grist W, Carlson GW, Christensen AM, Weimer KA, Steed MB. The accuracy of virtual surgical planning in free fibula mandibular reconstruction: Comparison of planned and final results. J Oral Maxillofac Surg 2010; 68(11)2824–2832
- Abou-ElFetouh A, Barakat A, Abdel-Ghany K. Computer-guided rapid-prototyped templates for segmental mandibular osteotomies: A preliminary report. Int J Med Robot 2011; 7(2)187–192
- Mac-Thiong JM, Labelle H, Rooze M, Feipel V, Aubin CE. Evaluation of a transpedicular drill guide for pedicle screw placement in the thoracic spine. Eur Spine J 2003; 12: 542–547
- Lu S, Xu YQ, Lu WW, Ni GX, Li YB, Shi JH, Li DP, Chen GP, Chen YB, Zhang YZ. A novel patient-specific navigational template for cervical pedicle screw placement. Spine (Phila Pa 1976) 2009; 34(26)E959–E966
- Hafez MA, Chelule KL, Seedhom BB, Sherman KP. Computer assisted total knee arthroplasty using patient-specific templating. Clin Orthop Relat Res 2006; 444: 184–192
- Kunz M, Rudan JF, Xenoyannis GL, Ellis RE. Computer-assisted hip resurfacing using individualized drill templates. Arthroplasty 2010; 25(4)600–606
- Murase T, Oka K, Moritomo H, Goto A, Yoshikawa H, Sugamoto K. Three-dimensional corrective osteotomy of malunited fractures of the upper extremity with use of a computer simulation system. J Bone Joint Surg Am 2008; 90(11)2375–2389
- Miyake J, Murase T, Moritomo H, Sugamoto K, Yoshikawa H. Distal radius osteotomy with volar locking plates based on computer simulation. Clin Orthop Relat Res 2011; 469(6)1766–1773
- Wong KC, Kumta SM, Leung KS, Ng KW, Ng EW, Lee KS. Integration of CAD/CAM planning into computer assisted orthopaedic surgery. Comput Aided Surg 2010; 15(4–6)65–74
- Cartiaux O, Docquier PL, Paul L, Francq BG, Cornu OH, Delloye C, Raucent B, Dehez B, Banse X. Surgical inaccuracy of tumor resection and reconstruction within the pelvis: An experimental study. Acta Orthop 2008; 79(5)695–702
- Pettersson A, Kero T, Gillot L, Cannas B, Fäldt J, Söderberg R, Näsström K. Accuracy of CAD/CAM-guided surgical template implant surgery on human cadavers: Part I. J Prosthet Dent 2010; 103(6)334–342
- Kero T, Pettersson A, Fäldt J, Andersson M, Gillot L, Cannas B, Näsström K, Söderberg R. Virtual variation simulation of CAD/CAM template-guided surgeries performed on human cadavers: Part II. J Prosthet Dent 2010; 104(1)48–55