Abstract
Objective: In the practice of plastic surgery, ribs and cartilage are often harvested for use in auto-grafts. This study aimed to elucidate the effect of such harvesting on the inspiration movement of the thorax.
Materials and Methods: Sixteen three-dimensional computer simulation models were produced by reference to the CT data of 16 patients. To simulate the harvesting of ribs and costal cartilages, parts were removed from these thorax models, thereby producing models with different types of defect. By performing finite element calculation after applying contracture forces on the external intercostal muscles and diaphragm, the movement patterns exhibited by the thoraces during inspiration were analyzed. The relationships between the defect types and the movement patterns were evaluated with reference to the dynamic behavior of the sternum and the increase in thoracic volume.
Results: Although the removal of costal cartilage can cause asymmetric movement of the thorax, it did not affect the inspiration movement of the thorax. However, removal of two or more ribs not only caused asymmetric movement of the thorax, but also negatively affected that movement. Removal of two or three ribs impaired the expansion of the hemi-thorax on inspiration by 10% and 40%, respectively.
Conclusion: The present study is the first to clarify the quantitative effect of tissue harvesting from the thorax on inspiration. The findings will be useful for surgeons when planning operations requiring harvesting of tissue from the thorax.
Introduction
In the practice of plastic surgery, transplantation of auto-grafts – such tissues as auricular cartilage Citation[1–6], nasal septum cartilage Citation[7], and iliac bone Citation[8] – is often conducted. Besides these tissues, ribs and costal cartilages are also harvested and used for augmentation of the nose Citation[9] or reconstruction of the ear Citation[10–16] or skull. As materials for auto-graft transplantation, ribs and costal cartilages differ from the other materials mentioned because they are harvested from the thorax – a structure whose movement is crucial to maintain life. Usually, harvesting of ribs and costal cartilages has no effect on respiratory function, at least not to a life-threatening degree Citation[17]. However, a lack of any obvious functional loss does not necessarily mean that harvesting of these tissues has no effect on respiratory functions at all Citation[18].
The movement of the thorax can be analogized by the model shown in , in which bars are connected with springs. The bars correspond to the ribs and the springs to the intercostal muscles. Respiration occurs as a result of concerted movement of the bars and springs (, left). When parts of the bars are removed, the disconnection might hinder transmission of the dynamic effects of the springs, such that the concerted movement of the thorax as a whole may be impaired (, right). Because of this mechanism, it is possible that when some parts of the thorax are removed, the concerted movement of the ribs and intercostal muscles may be disturbed and respiratory function negatively affected.
Figure 1. Mechanism of inspiration. Left: Normal inspiration. Ribs (indicated by beams) are connected with springs. As the springs contract, the ribs exhibit concerted movement. Right: When parts of the beams are removed, the movement exhibits disharmony.
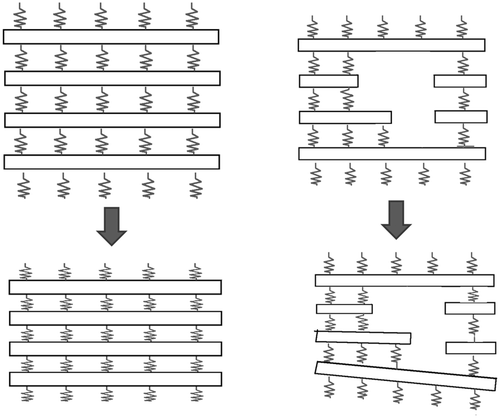
The above-stated consideration deserves particular attention when performing reconstruction in patients with poor general conditions. For instance, when plural ribs are needed for the reconstruction of skull defects caused by the removal of brain tumors, the patients are often elderly and in poor physical condition Citation[19]. To perform operations safely in these patients, surgeons must consider beforehand whether or not harvesting ribs will impair their respiratory function. For effective consideration, it is necessary to understand the effect on thoracic movement of any partial defects that may be produced, and the present study aimed to achieve such an understanding.
Materials and methods
Production of 3D thorax models
CT data were acquired of the chests of 16 subjects (8 males, 8 females; mean age 31.2 ± 8.4 years). None of the subjects had any deformity or disease of the chest. The data were then transformed to DICOM format. The thoracic part of the data was extracted using graphic software (Rhinoceros 4.0, Robert McNeel & Associates, Seattle, WA) (, left), and the thoraces were transformed into dynamic models Citation[20]. The transformation was performed by simulating the bones and cartilages with beams (, right) and by simulating muscles and ligaments with non-linear springs ().
Figure 2. CT data from 16 patients (left) were transformed into 3D models consisting of beams and non-linear springs (right). See also .
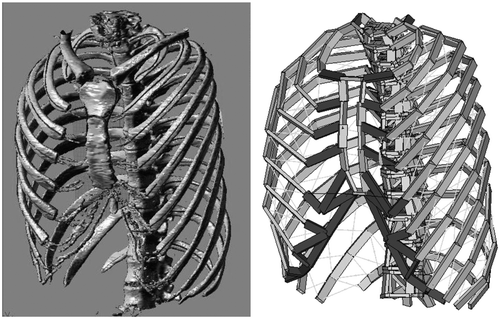
Figure 3. Inspiration muscles were modeled with non-linear springs. The red and yellow segments in the figure indicate springs simulating muscle fibers of the diaphragm and external intercostal muscles, respectively. The bones – simulated with beams – are shown thinner than in actuality to enhance the spring elements.
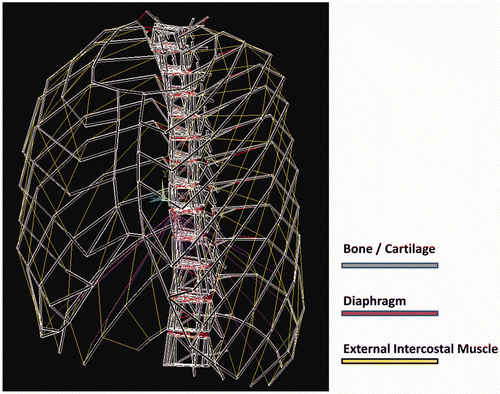
The transformation was performed using multi-purpose structural analysis software (ANSYS Workbench, ANSYS Co., Chicago, IL). In the process of element analysis, these components – beams and springs – are called elements. To reproduce interactions between elements of the original thorax appropriately, accurate material properties must be allocated to each element. These material properties were decided as follows:
Bones and cartilages
Young's moduli (E) of bone correlate with CT density (QCT), a relationship described by the equation E = −34.7 + 3230QCT Citation[21]. Based on this equation, the Young's modulus was calculated for each cartilage and bone of each thorax. shows the range and median of the Young's moduli calculated for the bone (ribs and spine) and costal cartilage. Poisson's ratio was set at 0.3 for both bone and cartilage.
Table I. Young's moduli for bone and cartilage (MPa).
Respiratory muscles
The external intercostal muscle and diaphragm were simulated with non-linear springs (Mooney-Rivilin model) and incorporated into the model (). Material properties of these springs are given as a relationship between stress and strain. This relationship – shown in – was obtained from the literature Citation[22].
Table II. Stress-strain relationships for respiratory muscles.
Ligaments
Costal-sternal ligaments, intervertebral ligaments, and costal-vertebral ligaments were simulated with non-linear springs (Mooney-Rivilin model) and incorporated into the model (). Material properties of these springs are given as a relationship between stress and strain. This stress-strain relationship – shown in – was obtained from the literature Citation[23].
Table III. Stress-strain relationships for ligaments.
Modification of 3D thorax models
The models produced through the above processes were categorized as Normal Type. By modifying each of the 16 models belonging to the Normal Type, models with various defects were produced. The modifications were performed as described below (). Since all of the following modifications were performed with each of the 16 Normal Type models, each of the following types had 16 models.
Type I-a: Thorax models with a small defect of the costal cartilage region. Produced by removing the 5th costal cartilage from the left hemi-thorax.
Type I-b: Thorax models with a large defect of the costal cartilages. Produced by removing the 5th and 6th costal cartilages and costal arch from the left hemi-thorax.
Type II-a: Thorax models with a defect of a single rib. Produced by removing the 8th rib from the left hemi-thorax.
Type II-b: Thorax models with a defect of two ribs. Produced by removing the 8th and 9th ribs from the left hemi-thorax.
Type II-c: Thorax models with a defect of three ribs. Produced by removing the 8th, 9th and 10th ribs from the left hemi-thorax.
Simulation of inspiration
After fixing the 1st and 12th vertebrae, loads were applied on each thorax model by assuming 15% contracture of the non-linear springs simulating the external intercostal models and diaphragm. The thorax transforms in response to this loading (). By performing dynamic calculation with software for multi-purpose finite element analysis (ANSYS, ANSYS Co., Chicago, IL), transformation patterns exhibited by the models on inspiration were obtained.
Figure 5. An example of thoracic transformation presented by a Type I-b model. The left and right figures indicate thorax shapes before (left) and after (right) inspiration. The dotted lines in the figures at right indicate the thorax shape before inspiration. The contours indicate the degree of deviation in millimeters.
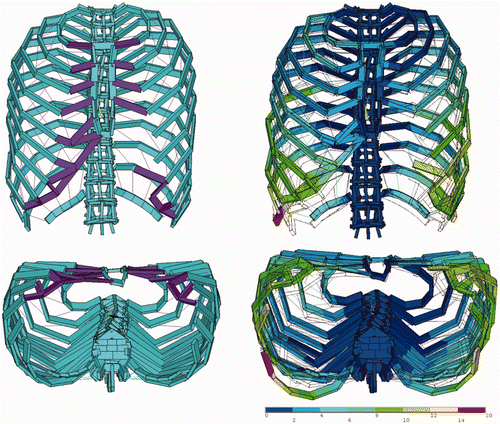
Quantitative evaluation of thorax transformation patterns
The transformation patterns presented by the thoraces on inspiration were evaluated with reference to the following parameters:
Increase in hemi-thorax volume on inspiration. The plane surrounded by the first ribs was defined as the upper boundary of the thorax; the planes connecting the bilateral 12th ribs and costal arches were defined as the lower boundary of the thorax (). The 3D region surrounded by the upper and lower lids and the thoracic wall was divided into right and left hemi-thoraces at the midline. The volumes of the left and right hemi-thoraces were defined as LTV (Left Thoracic Volume) and RTV (Right Thoracic Volume), respectively. The increases in LTV and RTV on inspiration were defined as ΔLTV and ΔRTV, respectively.
Evaluation of asymmetric movement. The angle of rotation presented by the sternum on inspiration was defined as ASR (Angle of Sternal Rotation). ASR was measured at the level of the 4th costal-sternal joint, and was assigned positive values when the sternum rotated in the clockwise direction as viewed from the bottom ().
Statistical evaluation of results
ΔLTV, ΔRTV and ASR were compared between the models. Since these values presented skewed distributions, a non-parametric test (the Mann-Whitney test) was used for the comparison. For the statistical calculation, SPSS Version 17.0 (SPSS Inc., Chicago, IL) was used; p-values less than 0.05 were considered statistically significant.
Verification of methodology
The following experiments were performed to verify the validity of the present study's simulation system.
Evaluation of thoracic movement in live persons
Transformation patterns of the thorax on inspiration were examined with three volunteers. First, CT data of the thorax were collected at the resting stage of respiration. Five numbered marker points were set on the thorax: Points 1, 2 and 3 were marked at the most lateral points of the 3rd, 6th and 10th ribs, respectively; Points 4 and 5 were set at the sternal notch and xiphoid process, respectively. Three-dimensional coordinates of these points were measured using graphic software (Real Intage, K.G.T. Co., Tokyo, Japan). Thereafter, CT data were again collected at the maximum inspiration stage and the 3D coordinates of the marker points were measured in the same way. By comparing the 3D coordinates of the marker points before and after inspiration, the deviations exhibited by the marker points on inspiration were measured.
Simulation of inspiration in models
For each of the three volunteers, a 3D model was produced. Inspiration was then simulated by applying a contracture ratio on inspiration. The contracture ratio – set at 15%, as described in the subsection headed Simulation of inspiration – was adjusted in this verification experiment for each sample so that the displacement of Point 3 in the actual measurement matched its counterpart in the simulation. The displacements of the marker points in response to the thus-defined load were calculated.
Comparison of measured and calculated data
The measured and calculated data were compared for the five marker points, and a ratio determined in the form of the measured data expressed as a percentage of the calculated data. By this means, the compatibility of the data was evaluated.
Results
The values of ΔLTV, ΔRTV and ASR are shown in , and , respectively. For ASR, Types I-b, II-b, and II-c presented statistically significant differences compared with the Normal Type. ΔRTV presented no statistically significant difference between the Normal Type and any other type. ΔLTV presented a statistically significant decrease for Types II-b and II-c compared to the Normal Type.
Table IV. Angle of sternal rotation (ASR).
Table V. Increase in right thoracic volume (ΔRTV).
Table VI. Increase in left thoracic volume (ΔLTV).
Effect of costal cartilage harvesting
When costal cartilages are harvested from a wide region including the costal arch, the inspiration movement of the thorax presents asymmetric patterns.
Regardless of the size of the costal cartilages harvested, harvesting them from a hemi-thorax does not affect its inspiration movement.
Effect of rib harvesting
When two or more ribs are harvested from the thorax, asymmetric movement results upon inspiration.
When two or more ribs are harvested, the inspiration movement of the thorax is negatively affected. The expansion function of the hemi-thorax decreases by 10% and 40% for the harvesting of two and three ribs, respectively.
Verification of modeling system
For all marker points on all samples, the discrepancy between the calculated data and measured data was less than 10% (), indicating the validity of the simulation system.
Table VII. Measured values as a percentage of calculated values for three volunteer subjects. Numbers in parentheses indicate the measured value (numerator) and calculated value (denominator).
Discussion
Methodology
The present study sought to elucidate the influence of tissue harvesting from the thorax on its movement during inspiration. A 3D simulation system using finite element analysis was the principle method employed. Regarding this methodological choice, some might question the use of simulation instead of evaluation of actual patients, because the study design might be more straightforward if actual patients were used. However, such a study is difficult to conduct with real patients because ribs and costal cartilages are harvested in a wide range of patterns, and obtaining patient data for any single pattern in numbers sufficient for statistical analysis is problematic. Furthermore, to evaluate the movement patterns of the thorax accurately, it is necessary to visualize the positional change of each thoracic component, and this is also difficult to achieve through studies on actual patients.
These problems can be solved by employing a simulation system. In contrast to actual patients, our simulation system enables us to produce any type of defect at will. Hence, we can easily collect sufficient numbers of samples for statistical analysis. Furthermore, employment of finite element calculations enables measurement of the deviation of each part of the thorax with an accuracy of 0.1 mm, which is advantageous for quantitative evaluation of asymmetric movement of thoraces. For these reasons, we employed simulation with finite element models in the present study. Finite element analysis is an established study method in the field of biomechanics, and is used for dynamic analyses of such tissues as skin Citation[24–26], facial bones Citation[27–29] and thoraces Citation[30–32].
On the other hand, since finite element analysis is a theoretical methodology based on mathematical calculation, the results obtained do not necessarily reproduce reality. Hence, the present study proved the appropriateness of the simulation system by performing a verification experiment. The results of this verification experiment demonstrate optimal compatibility of the calculated and measured data, proving the validity of the methods used.
Review of the results
Herein, the present study's findings are reviewed with reference to the dynamic principles of inspiration. A normal thorax can be modeled with plural half-rings connected with springs: The half-rings and springs correspond to ribs/costal cartilages and external intercostal muscles, respectively. When the springs are loose, the half-rings droop (, top left). Under this condition – corresponding to the resting stage of respiration – the region surrounded by the half-rings presents a relatively small volume. However, the half-rings are pulled upward when the springs contract. Accordingly, the volume of the region surrounded by the half-rings increases (, bottom left). Inspiration movement is caused by this dynamic mechanism.
Figure 8. Left: Inspiration pattern of normal thorax. With the elevation of the ribs, the volume of the thorax increases. Center: Elevation of the thorax can occur even after the removal of costal cartilages, although some ribs (indicated with arrows) exhibit deviation during inspiration. Right: Elevation of the thorax is impaired when ribs are removed from the lateral parts.
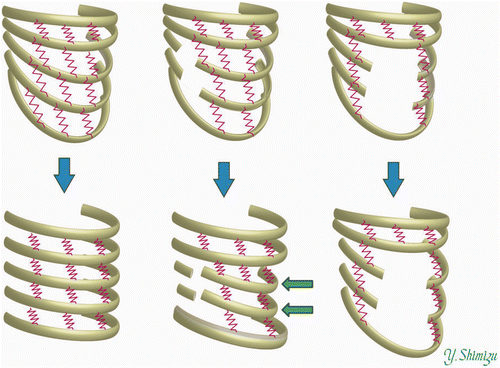
Let us consider how this analogy describes the removal of the ribs and costal cartilages. On inspiration, each half-ring presents a lifting rotation movement around the axis connecting the two ends of the half-ring. One end of the half-ring corresponds to the costal-sternal joint; the other end corresponds to the costal-vertebral joint. The costal cartilages exist close to the costal-sternal junction. Hence, the thorax from which the costal cartilages are harvested can be simulated with a model that has defects near the ends of the half-rings (, top center). With these models, dynamic connection of the half-rings is preserved, with the movements of the upper half-rings being transmitted to the lower rings, although some half-rings present slight deviation and move toward the axes. Hence, the volume increase of the region surrounded by the half-rings on inspiration does not present lower values than those for normal thoraces.
On the other hand, ribs are usually harvested from the side of the thorax. Hence, thoraces from which the ribs are harvested can be simulated with a model that has defects in the region away from the axes (, right). The sites of the half-rings on which the springs work are lacking in these models. Accordingly, movements of the upper half-rings are not effectively transmitted to the lower half-rings, and the expansion of the region surrounded by the half-rings does not occur. Hence, the increase in the thoracic volume is impaired in thoraces from which the ribs are harvested.
Clinical significance of the findings
The results of the present study are itemized and summarized as general rules in the Results section. Although these rules are derived from experiments on finite element models produced on the basis of CT data from young and middle-aged adults (with an average age of 31.2 years), they are also applicable to adolescent and elderly patients, because the general rules were obtained through inter-group comparison between a normal thorax group and each defect type. Even if age-related differences exist in thoracic volumes, the differences are offset during the process of inter-group comparison.
The general rules are helpful in deciding how ribs or costal cartilages are to be harvested by referring to specific conditions of the patients. Let us first consider costal cartilage harvesting. Costal cartilages are often harvested and used for ear reconstruction in cases of microtia, for mid-face reconstruction in Binder syndrome, or for mandible reconstruction in patients with micrognathia. Most of these operations are performed on children. Hence, if harvesting of the costal cartilages impairs growth of the respiratory function of a patient, this must be explained prior to the operation as a potential postoperative complication. If the patient does not accept this potential complication, costal cartilages are rendered unavailable, and alternative materials must be sought. However, the present study demonstrates that the inspiration function of the thorax is not impaired by the harvesting of costal cartilages - even when they are harvested from relatively wide regions. This is encouraging information for plastic surgeons treating the previously mentioned deformities, since it emancipates surgeons from having to present the patient with the likelihood of the abovementioned complication.
On the other hand, we have demonstrated that thoraces with wide-range defects of the costal cartilages (Type I-b) exhibit a greater degree of sternum rotation than normal thoraces. This finding indicates that harvesting of costal cartilages can induce asymmetric movement of the thorax. It is speculated that continuous asymmetric movement over a long period of time might cause morphological asymmetry of the thorax, which must be taken into consideration when determining the region from which costal cartilage is to be harvested.
We now turn to a consideration of rib harvesting. The volume increase of the hemi-thorax did not represent a statistically significant difference between normal hemi-thoraces and hemi-thoraces with a single rib defect. Hence, it can be concluded that harvesting of just one rib will have minimal effect on respiratory function. However, statistically significant differences in terms of the volume increase of the hemi-thorax were observed between normal thoraces and those with two or more ribs harvested. The volume increase of the hemi-thorax is reduced by 10% on average with the harvesting of two ribs. In practice, a 10% decrease would have little impact for most patients. Therefore, surgeons need not be greatly concerned about functional loss during inspiration when harvesting two ribs from the thorax. However, the function of thoracic expansion plummets, representing a 40% reduction on average, when three ribs are harvested. These findings provide a general rule that harvesting of more than three ribs substantially reduces respiratory function. Reference to such general rules would help surgeons when planning operations. For instance, when operating on elderly patients or those with poor pulmonary function, harvesting of three or more ribs should be avoided to prevent postoperative respiratory complications. Reconstruction of bone defects in the skull or facial bones by rib grafting is often performed on patients being treated for trauma or malignant tumors, and the general rules given in the present study would provide useful information on such occasions.
For many surgeons, it might be intuitively evident that harvesting of three or more ribs impairs respiratory movement of the thorax. However, the negligible effect of harvesting one or two ribs may be somewhat surprising. The present study is the first to demonstrate the degree of functional loss in a quantitative fashion. The authors believe that the findings will be helpful for plastic surgeons when considering surgical plans for operations requiring auto-grafting of ribs or cartilages.
Conclusion
The present study has evaluated how tissue harvesting from the thorax affects its movement and function during inspiration. Frequently performed tissue-harvesting patterns were categorized into five types, and 16 3D computer models were produced for each type. Inspiration was simulated with these models by applying contracture forces on inspiration muscles. Thereafter, inspiration movement of the thorax was evaluated in terms of function and asymmetry. Inspiration function was evaluated with reference to the increase in the hemi-thoracic volume on inspiration; asymmetry was evaluated with reference to the rotation angle of the sternum on inspiration.
Regardless of the area of the region of harvesting, the harvesting of costal cartilages does not affect the functional aspect of inspiration. However, when costal cartilages are harvested from a wide region including the costal arch, the thorax exhibits asymmetric movement. Harvesting of two or more ribs not only induces asymmetric movement of the thorax, but also negatively affects its inspiration function. Volume increase of the hemi-thorax on inspiration is reduced by 10% for two-rib harvesting, and by 40% for three-rib harvesting. These findings will be helpful for plastic surgeons when considering harvesting of costal cartilages or ribs.
Declaration of interest: The authors report no declarations of interest.
References
- Boccieri A. Subtotal reconstruction of the nasal septum using a conchal reshaped graft. Ann Plast Surg 2004; 53(5–6)118–125
- Constantian MB. Functional effects of alar cartilage malposition. Ann Plast Surg 1993; 30: 487–499
- Koch CA, Friedman O. Modified back-to-back autogenous conchal cartilage graft for caudal septal reconstruction. Arch Facial Plast Surg 2011; 13(1)20–25
- Chua DYK, Tan HKK. Repair of nasal septal perforations using auricular conchal cartilage graft in children: Report on three cases and literature review. Int J Pediatr Otorhinolaryngol 2006; 70(7)1219–1224
- Pirsig W, Kern EB, Verse T. Reconstruction of anterior nasal septum: Back-to-back autogenous ear cartilage graft. Laryngoscope 2004; 114(4)627–638
- Muenker R. The bilateral conchal cartilage graft: A new technique in augmentation rhinoplasty. Aesth Plast Surg 1984; 8(1)37–42
- Bayat M, Momen-Heravi F, Khalilzadeh O, Mirhosseni Z, Sadeghi-Tari A. Comparison of conchal cartilage graft with nasal septal cartilage graft for reconstruction of orbital floor blowout fractures. Br J Oral Maxillofac Surg 2010; 48(8)617–620
- Sakakibara S, Hashikawa K, Terashi H, Tahara S. Reconstruction of the orbital floor with sheets of autogenous iliac cancellous bone. J Oral Maxillofac Surg 2009; 67(5)957–961
- Sherris DA, Kern EB. The versatile autogenous rib graft in septorhinoplasty. Am J Rhinol 1998; 12(3)221–227
- Zim SA. Microtia reconstruction: An update. Curr Opin Otolaryngol Head Neck Surg 2003; 11(4)275–281
- Sabbagh W. Early experience in microtia reconstruction: The first 100 cases. J Plast Reconstr Aesthet Surg 2011; 64(4)452–458
- Matsumoto K. A new method of reconstruction in microtia, with emphasis on conchal creation. Ann Plast Surg 1980; 5(1)51–57
- Nagata S. A new method of total reconstruction of the auricle for microtia. Plast Reconstr Surg 1993; 92(2)187–201
- Nagata S. Modification of the stages in total reconstruction of the auricle: Part I. Grafting the three-dimensional costal cartilage framework for lobule-type microtia. Plast Reconstr Surg 1994; 93(2)221–230; discussion 267–268
- Nagata S. Modification of the stages in total reconstruction of the auricle: Part II. Grafting the three-dimensional costal cartilage framework for concha-type microtia. Plast Reconstr Surg 1994; 93(2)231–242; discussion 267–268
- Brent B. Technical advances in ear reconstruction with autogenous rib cartilage grafts: Personal experience with 1200 cases. Plast Reconstr Surg 1999; 104(2)319–334;, discussion 335–338
- Fonkalsrud EW. Open repair of pectus excavatum with minimal cartilage resection. Ann Surg 2004; 240(2)231–235
- Ohara K, Nakamura K, Ohta E. Chest wall deformities and thoracic scoliosis after costal cartilage graft harvesting. Plast Reconstr Surg 1997; 99(4)1030–1036
- Stueber K, Salcman M, Spence RJ. The combined use of the latissimus dorsi musculocutaneous free flap and split-rib grafts for cranial vault reconstruction. Ann Plast Surg 1985; 15: 155–160
- Yang TY. Basic principle of finite element analysis. In: Handbook of Finite Element Method for Structural Analysis. Gihodo Co., Tokyo 1996; 11–66
- Kopperdahl DL, Pearlman JL, Keaveny TM. Biomechanical consequences of an isolated overload on the human vertebral body. J Orthop Res 2000; 18(5)685–690
- Yamada H, Evans FG, editors. Strength of Biological Materials. Philadelphia, PA: The Williams & Wilkins Company; 1970.
- Abe H, Hayashi K, Sato M, editors. Data Book on Mechanical Properties of Living Cells, Tissues, and Organs. Berlin: Springer-Verlag; 1996.
- Akaishi S, Akimoto M, Ogawa R, Hyakusoku H. The relationship between keloid growth pattern and stretching tension: Visual analysis using the finite element method. Ann Plast Surg 2008; 60(4)445–451
- Miyamoto J, Nagasao T, Miyamoto S. Biomechanical analysis of surgical correction of syndactyly. Plast Reconstr Surg 2010; 125(3)963–968
- Miyamoto J, Nagasao T, Miyamoto S, Nakajima T. Biomechanical analysis of stresses occurring in vertical and transverse scars on the lower leg. Plast Reconstr Surg 2009; 124(6)1974–1979
- Nagasao T, Miyamoto J, Jiang H, Kaneko T, Tamaki T. Biomechanical analysis of the effect of intracranial pressure on the orbital distances in trigonocephaly. Cleft Palate Craniofac J 2011; 48(2)190–196
- Nagasao T, Miyamoto J, Jiang H, Tamaki T, Kaneko T. Interaction of hydraulic and buckling mechanisms in blowout fractures. Ann Plast Surg 2010; 64(4)471–476
- Nagasao T, Tamada I, Miyamoto J, Ogata H, Kaneko T, Nagasao M, Nakajima T. Effectiveness of additional transmalar Kirschner wire fixation for a zygoma fracture. Plast Reconstr Surg 2007; 119(3)1010–1019
- Nagasao T, Noguchi M, Miyamoto J, Jiang H, Ding W, Shimizu Y, Kishi K. Dynamic effects of the Nuss procedure on the spine in asymmetric pectus excavatum. J Thorac Cardiovasc Surg 2010; 140(6)1294–1299.e1
- Nagasao T, Miyamoto J, Ichihara K, Jiang H, Jin H, Tamaki T. Age-related change of postoperative pain location after Nuss procedure for pectus excavatum. Eur J Cardiothorac Surg 2010; 38(2)203–208
- Nagasao T, Miyamoto J, Tamaki T, Ichihara K, Jiang H, Taguchi H, Yozu R, Nakajima T. Stress distribution on the thorax after the Nuss procedure for pectus excavatum results in different patterns between adult and child patients. J Thorac Cardiovasc Surg 2007; 134(6)1502–1507