Abstract
Purpose: Anatomic double-bundle ACL reconstruction presents a unique technical challenge for surgeons, requiring precise placement of multiple tunnels in a relatively small area. As the necessity of anatomic reconstruction has been stressed throughout the literature, developing a method to consistently improve the accuracy and precision of tunnel placement is essential. We aimed to investigate whether computer-assisted navigation allows novice surgeons to place double-bundle ACL tunnels with a similar degree of accuracy to experienced surgeons operating without computer assistance.
Methods: A novice surgeon group comprising three medical students performed double-bundle ACL reconstruction using passive computer-assisted navigation in 11 cadaver knees. Their individual results were compared to those of three experienced orthopaedic surgeons, each performing the identical procedure without the use of computer-assisted navigation in 9 cadaver knees.
Results and conclusion: There were no significant differences in placement of either the AM or PL tunnels on the tibial plateau between the novice surgeons using computer-assisted navigation and the experienced surgeons. However, on the lateral femoral condyle, the novice surgeons placed the AM and PL tunnels significantly more anterior along Blumensaat’s line, on average, compared to the experienced surgeons.
Introduction
While the anterior cruciate ligament (ACL) is often referenced as a single entity, the two bundles originally described by Palmer in 1938 [Citation1] paved the way for a multitude of studies investigating the unique fascicles comprising the native ACL [Citation1–3]. Previous literature has described in great detail the positioning and location of the native anteromedial (AM) and posterolateral (PL) bundles of the ACL on the tibial plateau and lateral femoral condyle, using both anatomic and radiographic measures [Citation4–18]. Such studies are invaluable, as recurrent instability and graft failure develops in approximately 8% of patients who undergo primary ACL reconstruction, with tunnel malpositioning being implicated in as many as 70–80% of these technical failures [Citation19]. Previous studies have shown that tunnel malpositioning may result in failure to reproduce the native biomechanics of the ACL, increase graft tension in deep knee flexion, increase anterior tibial translation, and lower IKDC (International Knee Documentation Committee) scores [Citation18, Citation20–24]. Because of these previous findings, a great deal of effort has gone into developing methods to aid surgeons in proper tunnel positioning. To evaluate tunnel placement, radiographic techniques using plain radiography or cross-sectional imaging are often implemented. Previously described techniques by Bernard et al. [Citation4] and Stäubli and Rauschning [Citation14] use known bony landmarks on plain radiographs to locate the anatomic femoral and tibial ACL insertions. Slight modifications to the aforementioned techniques can be made in order to use cross-sectional imaging such as computed tomographic (CT) imaging, allowing preoperative planning in three planes to better define anatomic landmarks in space [Citation11].
While double-bundle reconstruction attempts to recreate the two-bundle anatomy of the native ACL, recent research indicates that double-bundle reconstruction also more closely reproduces the biomechanical properties of the ACL [Citation25–26]. Several studies have indicated that double-bundle reconstruction restores rotatory and sagittal stability to the level of the intact knee, which was not attainable with anatomic single-bundle reconstruction [Citation25–27]. However, while double-bundle reconstruction provides these biomechanical benefits, it also presents a significant challenge to the surgeon, who must attempt to place accurately twice as many tunnels as in single-bundle reconstruction while avoiding tunnel convergence [Citation28].
Early studies noted that placing two bundles in the proper anatomic position posed significant challenges and introduced the idea of using computer guidance systems to aid arthroscopic reconstruction [Citation7]. More recent studies have followed up on that suggestion, and there are numerous reports describing the outcomes of ACL reconstruction using computer-assisted surgery (CAS) with varying degrees of success [Citation29–33]. While there has been no general consensus as to the efficacy of CAS in improving the accuracy and precision of tunnel placement or overall patient outcome, it has been generally accepted that CAS increases costs and prolongs operating time, despite there being insufficient evidence to comment on its effectiveness [Citation34–35]. In the absence of definitive answers, it has been speculated that less experienced surgeons may benefit more from using CAS [Citation34], but research to support such speculation is lacking and more work needs to be done to investigate the effects of CAS for surgeons of different skill levels.
We hypothesized that experienced surgeons operating without the use of computer-assisted navigation would place tunnels on the tibial plateau and femoral condyle in positions that more accurately reflected the native AM and PL bundle locations than those tunnels placed by inexperienced surgeons operating with the use of computer-assisted navigation.
Materials and methods
Our study used 20 fresh-frozen human cadaver knees sectioned at mid-thigh and mid-tibia (10 right and 10 left specimens) to ascertain the accuracy of AM and PL tunnel placement by novices and experienced surgeons. The sample size required for this study was determined a priori using the software G*Power 3.1.2 (Heinrich-Heine-Universität Dusseldorf, Dusseldorf, Germany). To determine effect size, we used previous work showing that graft placement differing from anatomic position by more than 3 mm may affect knee kinematics [Citation18], and that AM bundle position along Blumensaat’s line varies by 2.6% [Citation5]. These data were used to determine our effect size coupled to a power requirement of 0.8, p-value of <0.05 and alpha level of 95%. These parameters required that our sample size be set at 9 knee specimens per group, though, given the possibility of novice surgeon operator failure, we chose to set the sample size at 11 knee specimens for the novice surgeon group and 9 specimens for the experienced surgeon group. Cadaver knee specimens were stored at −20°C prior to being thawed at room temperature over a 24-hour period.
Preoperative MR images were obtained using a Siemens TIM Trio 3.0-T MRI scanner (Siemens Medical Solutions, Erlangen, Germany) (3D-DESS acquisition; TR/TE = 16.1/5.4 ms; in-plane resolution 0.45 mm; slice thickness 0.50 mm). Each preoperative knee MR sequence was evaluated for intact soft tissue structures, including the ACL, PCL, MCL and LCL, and bony anatomy in order to exclude any pathologic specimens. The tibial footprint of the ACL was analyzed preoperatively using sagittal and coronal MR images generated from the near-isotropic 3D volume (). In the sagittal plane, an independent observer measured the anterior, center, and posterior extent of the intact tibial footprint, which were expressed as a percentage length from the anterior tibial edge. In the coronal plane, the same observer measured the medial, center, and lateral extent of the intact tibial footprint, which were expressed as a percentage width from the medial tibial plateau. This allowed the footprint to be projected as a rectangle on axial MR images () for eventual postoperative analysis of tibial tunnel placement relative to the footprint. The native footprint of the ACL on the lateral femoral condyle was not measured, as MR images proved to be inadequate for proper evaluation. All other measurements were deferred to the postoperative period.
Figure 1. Mean preoperative tibial ACL footprint as determined using sagittal and coronal MRI with overlay on an axial image. The maximum sagittal and coronal widths of the tibia were determined, and the anterior, posterior, medial and lateral extents of the ACL were measured on MRI and expressed as a percentage of the maximum tibial width from the most anterior and medial aspects of that width. The mean center of the footprint is also expressed as a percentage width.
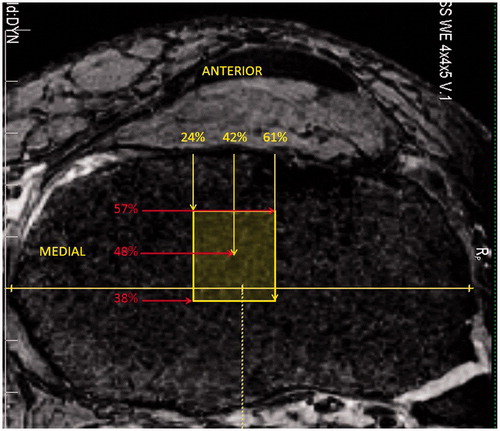
After preoperative analysis, the ACL of each knee was completely bisected and debrided in order to replicate a complete ACL tear. The native stalks of the ACL on both the tibial plateau and lateral condyle of the femur were left intact. Cadaver knees were randomly assigned to one of two groups of surgeons using a random number generator. The novice surgeon group consisted of three medical students with no prior surgical experience and only general knowledge of the knee anatomy. Prior to performing ACL reconstruction, this group was oriented to an FDA-approved computer-assisted surgery system (OrthoPilot®, Aesculap Implant Systems, Tuttlingen, Germany) and basic arthroscopic equipment. This orientation period consisted of an 8-hour period of supervised instruction on the handling and use of arthroscopic equipment, as well as a separate 8-hour period of supervised instruction with the computer-assisted navigation system. During both the arthroscopic and computer-assisted navigation orientations, instruction was given on the correct identification of pertinent knee anatomy, including various extra- and intra-articular bony and soft tissue landmarks. The novice surgeon group was subsequently given two cadaver knee specimens on which to practice before the official study began. The experienced surgeon group consisted of three orthopaedic surgeons with a combined 52 years of post-fellowship experience (range: 2–42 years). Each surgeon in both groups was provided with the same set of arthroscopic tools for the double-bundle reconstruction, with the exception of the CAS system, which was only available to the novice group.
Each group used standardized arthroscopic portals and drilled the femoral tunnels through the anteromedial portal using a 6-mm-diameter drill, allowing the femoral tunnels to be placed independently and outside the constraint of the tibial tunnels. The computer-assisted navigation configuration required the novice surgeon to identify preoperatively various extra- and intra-articular landmarks, including the medial and lateral edges of the tibial plateau, the tibial tubercle, the anterior horn of the lateral meniscus, the medial intercondylar eminence, the posterior cruciate ligament, the inferior edge of the lateral femoral notch wall, and the lateral femoral notch wall ACL footprint. These landmarks were subsequently used by the CAS software to display and communicate intraoperative tool positioning to the novice surgeons through a graphical user interface (GUI) (). Once each subject had located the optimal tunnel location to the best of their ability, a guide pin was placed and the AM and PL tunnels were drilled using a 6-mm drill. Each novice surgeon was asked to determine optimal tunnel location by comparing the intraoperative measurements and readouts of the computer-assisted navigation system to the locations of the AM and PL bundles previously described in the literature. Two novice surgeons each performed three individual double-bundle procedures and one novice surgeon performed five procedures. The experienced surgeon group followed a similar protocol but without the aid of computer navigation. Each experienced surgeon was instructed to position the AM and PL tunnels on both the tibial plateau and lateral notch wall of the femur to the best of their ability using their prior surgical experience and knowledge of the literature. Each experienced surgeon performed three individual double-bundle procedures.
Figure 2. Computer-assisted navigation graphical user interface as used by the novice surgeon group, allowing the operator to understand the location on the tibial plateau and lateral femoral condyle of the right knee. (A) The interface for the tibial side. (B) The interface for the femoral side.
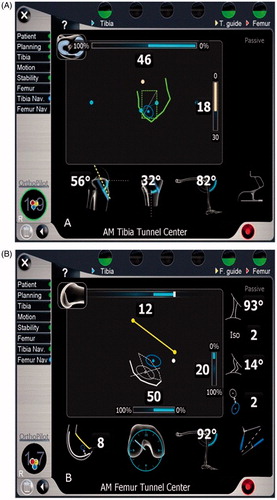
Postoperatively, each knee was imaged using MR with the above-mentioned parameters, and measurements were made by an independent evaluator. AM and PL tunnel locations on the tibial side were determined with slight modification to the technique described by Stäubli and Rauschning [Citation14]. The maximum sagittal diameter of the tibial plateau was measured and copied to all images (). The slices in which the AM and PL tunnels broke through the subchondral bone of the tibia were then identified, and measurements were made perpendicular to the maximum sagittal diameter from the most anterior aspect of that line (). Distances were expressed as a percentage of total sagittal width to account for variability in knee size, as recommended by the first European Society of Sports Traumatology Knee Surgery and Arthroscopy (ESSKA) Scientific Workshop [Citation21]. The same method was used in the coronal plane on the tibial plateau to identify the center of both the AM and PL tunnels. In the coronal plane, all measurements were made from the most medial aspect of the maximum coronal width line and again expressed as a percentage of the total coronal width (). By locating the tunnels in the sagittal and coronal planes, it was possible to determine whether the tunnels fell within the preoperatively MR-measured tibial ACL footprint.
Figure 3. Tibial measurements were made using sagittal and coronal MR images. The maximum sagittal width of the tibia parallel to the joint line, S, was measured and copied to all images (A). The slice at which the AM or PL tunnel broke the subchondral bone of the tibial plateau was located and measured perpendicular to line S. The distance S1 was expressed as a percentage of the maximum sagittal width (B). The maximum coronal width was measured and represented by line C (C). The slice at which the AM or PL tunnel broke the subchondral bone was identified and measured perpendicular to line C. The distance C1 was expressed as a percentage of the maximum coronal width (D).
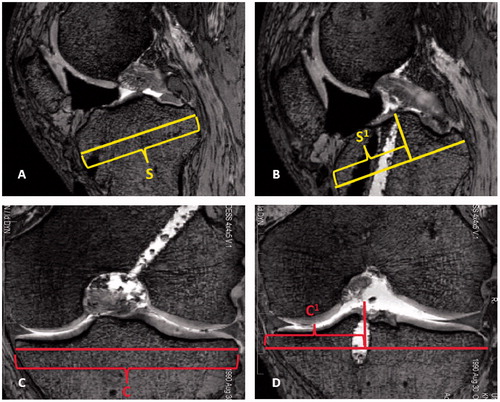
The locations of the AM and PL tunnels on the lateral femoral condyle were determined using the techniques described by Bernard et al. [Citation4] and are visualized in . The most proximal extent of the intercondylar notch representing Blumensaat’s line was identified (), and the maximum sagittal width along Blumensaat’s line was identified and copied to all images (). The most distal aspect of the lateral femoral condyle was then identified and a line was drawn tangential to that point and perpendicular to Blumensaat’s line in order to determine the notch height (). The slice in which the AM or PL tunnel broke through the bony cortex of the lateral femoral condyle was identified, and measurements were made to the center of the tunnel along Blumensaat’s line and the notch height (). Each distance was expressed as a percentage from the most posterior aspect of Blumensaat’s line and from the most proximal aspect of the notch height.
Figure 4. Femoral measurements were made on sagittal MR images of the lateral femoral condyle using modifications to the quadrant technique described by Bernard et al. [Citation4]. First, the most proximal aspect of the femoral notch was identified, correlating to Blumensaat’s line (A). Next, the maximum sagittal width along Blumensaat’s line (W) was identified, measured, and copied to all images (B). The most distal aspect of the lateral femoral condyle was then identified and a line drawn tangential to that point. The perpendicular distance from Blumensaat’s line to this line was the notch height, H (C). Lastly, the slice at which the AM or PL tunnel broke the subchondral bone of the lateral femoral condyle was identified. Measurements were made perpendicular to lines W and H in order to locate the center of the tunnel. The center of the tunnel was expressed as a percentage along Blumensaat’s line and the notch height, displayed as W1 and H1, respectively (D).
![Figure 4. Femoral measurements were made on sagittal MR images of the lateral femoral condyle using modifications to the quadrant technique described by Bernard et al. [Citation4]. First, the most proximal aspect of the femoral notch was identified, correlating to Blumensaat’s line (A). Next, the maximum sagittal width along Blumensaat’s line (W) was identified, measured, and copied to all images (B). The most distal aspect of the lateral femoral condyle was then identified and a line drawn tangential to that point. The perpendicular distance from Blumensaat’s line to this line was the notch height, H (C). Lastly, the slice at which the AM or PL tunnel broke the subchondral bone of the lateral femoral condyle was identified. Measurements were made perpendicular to lines W and H in order to locate the center of the tunnel. The center of the tunnel was expressed as a percentage along Blumensaat’s line and the notch height, displayed as W1 and H1, respectively (D).](/cms/asset/9ba84f98-de45-4040-bc0a-64ae2bff0848/icsu_a_795244_f0004_b.jpg)
Statistical analysis was performed using SAS/STAT® software (SAS Institute, Cary, NC). Mean values for the preoperative tibial footprint as well as the postoperative tunnel locations for the novice and experienced surgeon groups were calculated. Means were obtained for both absolute values measured in millimeters as well as percentage values. A two-tailed Student’s t-test was used to compare tunnel location in order to determine statistically significant differences between the novice and experienced surgeon groups. Significance was defined as a p-value <0.05, yielding an alpha level of 95%.
Results
Comparing the results for the novice and experienced surgeon groups, there were no statistically significant differences in AM or PL tunnel position on the tibial plateau as evaluated in both the sagittal and coronal planes (). However, on the femoral condyle, the novice surgeon group placed the AM tunnel significantly anterior along Blumensaat’s line compared to the experienced surgeon group (p = 0.0073) (). The PL tunnel was also placed significantly more anterior on the femoral condyle by the novice surgeon group compared to the experienced surgeon group (p-value = 0.0098). There were no significant differences between novice and experienced surgeons in tunnel placement with respect to the notch height for both the AM and PL tunnels (). Novice surgeons using CAS successfully placed the centers of both the AM and PL tunnels within the confines of the MR-measured tibial ACL footprint in 9 of 11 cases. In one case the PL tunnel was placed 1 mm posterior to the footprint, and in the other case an AM tunnel was placed 1 mm medial to the footprint. The experienced surgeon group successfully placed the centers of both the AM and PL tunnels within the confines of the MR-measured tibial ACL footprint in 8 of 9 cases. In a single case the PL tunnel was placed 1 mm posterior to the native footprint.
Table I. Preoperative dimensions of the tibial ACL footprint.
Table II. Comparison of tunnel placement by experienced and novice surgeons expressed as percentage values.
On average, the preoperative tibial ACL footprint measured 21 ± 2.0 mm in the sagittal plane and 14 ± 1.5 mm in the coronal plane. The footprint was centered at 42 ± 3.7% in the sagittal plane and 48 ± 2.1% in the coronal plane ( and ). The novice surgeon group placed the tibial AM tunnel at an average of 32.1 ± 7.1% and the tibial PL tunnel at an average of 54 ± 5.8% of the sagittal distance of the tibial plateau as measured from the anterior cortex. The experienced surgeon group placed the tibial AM tunnel at an average of 37.7 ± 7.4% and the tibial PL tunnel at an average of 53 ± 8.7%. In the coronal plane, the novice surgeon group placed the tibial AM tunnel at an average of 43 ± 3.3% and the PL tunnel at an average of 47 ± 3.0% of the maximum coronal width as measured from the medial cortex. The experienced surgeon group placed the tibial AM tunnel at an average of 43 ± 1.8% and the tibial PL tunnel at 49 ± 2.2%. On the lateral femoral condyle, the novice surgeon group placed the femoral AM tunnel at an average of 43 ± 10% along the distance of Blumensaat’s line and 11 ± 11% of the notch height, while the experienced surgeon group placed the femoral AM tunnel at an average of 30 ± 7.2% along Blumensaat’s line and 5.9% ± 6.5% of the notch height. The novice surgeon group placed the femoral PL tunnel at an average of 46 ± 7.3% along Blumensaat’s line and 49 ± 12% of the notch height, while the experienced surgeon group placed the femoral PL tunnel at an average of 37 ± 7.2% along Blumensaat’s line and 42 ± 14% of the notch height.
Discussion
There is a great deal of literature describing the locations of the native AM and PL bundles [Citation4–17, Citation28]. More recently, the importance of performing anatomic double-bundle ACL reconstruction has been emphasized, considering the improved biomechanics and outcomes with anatomic reconstruction [Citation18, Citation22, Citation25, Citation26]. Still, achieving such anatomic reconstruction is often easier said than done, as technical failure, most notably tunnel malpositioning, continues to be one of the most common factors in ACL reconstruction failure [Citation19]. Our work was initiated by a desire to better understand double-bundle ACL reconstruction and evaluate a previously suggested technology, computer-assisted surgery, that may allow surgeons to place tunnels more accurately and precisely. We also hoped to challenge the boundaries and limitations of how CAS may be used in ACL reconstruction by designing a study that used medical students to represent a truly novice group of surgeons. The results of this study reiterate that tunnel positioning remains a challenging aspect of double-bundle ACL reconstruction, even with computer assistance.
The tibial footprint of the ACL evaluated using MRI in the present study was slightly larger in both the sagittal and coronal planes than that described previously in anatomic dissections [Citation3, Citation5, Citation7, Citation9, Citation14]. However, the center of the tunnel expressed as a percentage of the sagittal and coronal width was consistent with the aforementioned studies. Several of these studies dissected the synovium overlying the intact ACL prior to making width measurements [Citation9, Citation28]. As our measurements were made using MRI, some additional width could be attributed to the intact synovium. Additionally, as noted previously, there is a great deal of inter-study variation in the size, shape, and method of measurement of the tibial footprint, making it difficult to compare studies and apply the data clinically [Citation7, Citation9].
There was no significant difference between novice and experienced surgeons in the placement of the AM and PL tunnels on the tibial side. On the femoral side, however, novice surgeons placed both the AM and PL tunnels significantly more anterior along Blumensaat’s line compared to experienced surgeons who performed the procedure without the theoretical advantage of computer navigation (). This is of particular importance considering that small changes in femoral tunnel placement have a profound effect on laxity and graft tension [Citation18, Citation20–22, Citation24]. When converting the average difference between the groups in AM and PL tunnel positioning along Blumensaat’s line into absolute distances (AM 6 mm; PL 5 mm), the present study finds support in the previous literature indicating that knee kinematics would most likely be affected by the average anterior placements of the novice surgeon group [Citation18, Citation20–22, Citation24]. With regard to CAS, our findings are consistent with those reported throughout the literature. In a Cochrane Review, Meuffels et al. [Citation35] found that there were no differences in subjective knee outcome scores, knee stability, tunnel placement, or adverse events when comparing the four randomized control trials that evaluated computer-assisted ACL reconstruction versus conventional arthroscopic reconstruction [Citation35]. They speculated that use of CAS may aid less experienced surgeons more than experienced surgeons. While the present study did not compare novice surgeons with and without the aid of computer navigation, our findings suggest that CAS allows novice surgeons to perform at the level of experienced surgeons on the tibial side with respect to anatomic tunnel location. On the other hand, the study also demonstrates that CAS does not allow novice surgeons to perform at the same level as experienced surgeons with respect to anatomic tunnel location on the lateral femoral condyle.
Both the novice and experienced surgeons placed the AM and PL tunnels on the tibial side in similar locations to those reported in previous anatomic studies (). Both groups had a tendency to place the AM and PL tunnels more anteriorly along Blumensaat’s line compared to previous anatomic studies evaluating AM and PL tunnels on the lateral femoral condyle ( and ) [Citation5, Citation11, Citation12, Citation17, Citation26, Citation28]. While this could simply be attributed to the technical difficulty of placing the femoral tunnels [Citation21], several other factors may play a role. Our measurements were made from cross-sectional MR images, and as femoral tunnels enter the lateral femoral condyle obliquely, it is often difficult to determine exactly where the tunnels break through the bony cortex, resulting in small discrepancies compared to measurements made in situ on cadavers using calipers, where it may be easier to locate the precise point of osseo-ligamentous integration. Several studies have described femoral tunnel placement using single-bundle reconstruction in vivo [Citation34, Citation36]. However, relatively little information exists regarding femoral tunnel position following anatomic double-bundle reconstruction. Iriuchishima et al. [Citation8] compared AM and PL tunnel placement on the lateral femoral condyle in cadaveric knees and in vivo. Their measurements were made using the quadrant technique described by Bernard et al. using true lateral radiographs of the knee [Citation4]. In their study, the AM and PL tunnels were placed at 22 and 28% of the total sagittal width along Blumensaat’s line, respectively, on the in vivo knees [Citation8]. Taketomi et al. presented similar findings while investigating whether the order of AM or PL tunnel drilling affected graft placement. Using 3D CT imaging, the AM and PL tunnels were placed at 22.4 and 35.0%, respectively, along Blumensaat’s line [Citation37]. In a learning curve study by Snow and Stanish [Citation38], the AM and PL tunnels were placed 22.8 and 49.1% of the total sagittal diameter along Blumensaat’s line, and anatomic placement improved as the single surgeon in the study performed subsequent surgeries. In the present study, experienced surgeons placed the AM and PL tunnels at 30 and 37%, respectively, along Blumensaat’s line.
Figure 5. Centers of the anteromedial (AM) and posterolateral (PL) tunnels mapped on the lateral femoral condyle for each knee studied. Tunnel location was determined using the extension of Blumensaat’s line and notch height on sagittal MRI. Novice surgeon computer aided AM and PL tunnels were significantly anterior to tunnels made by experienced surgeons. The range of AM and PL centers from previous anatomic studies are also mapped on the lateral femoral condyle for comparison.
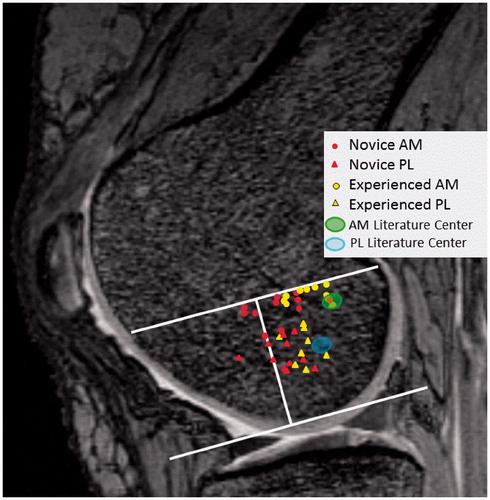
Table III. Overview of studies evaluating the femoral and tibial insertion of the AM and PL bundles compared to tunnel position in the present study. All numerical values are percentages.
Differences between the studies could partially be attributed to measuring technique. In all the aforementioned studies, as well as in the present study, the center of the AM or PL tunnel was referenced for measurements. In our experience, determining the center of the tunnel is complicated by tunnel obliquity, rotation, and artifact, depending on the imaging modality of choice, which makes identification of the center of the tunnel a best estimate. Furthermore, the 8% difference in AM tunnel position between the present study and that of Taketomi et al. results in an absolute difference of only 3.3 mm, given the mean sagittal width along Blumensaat’s line of 46.5 mm in the present study. Such a difference falls within the breadth of the 6-mm-diameter drill used to create the tunnel, though, as a previous study has noted [Citation18], this may still be enough to incite knee instability. The type of imaging used also varied in each study and included true lateral radiographs, 3D CT, and MR images, making comparisons between studies difficult. Additionally, pending longer follow-up and more expansive clinical studies, double-bundle reconstruction is not the primary reconstruction technique performed by surgeons at this institution. Therefore, the results obtained by experienced surgeons may change in the future due to the learning curve described by Snow and Stanish [Citation38]. As there is a general paucity of literature evaluating tunnel placement following double-bundle reconstruction, the values obtained in the present study, as well as in the aforementioned studies, could fall within the variance and standard deviation of tunnel placement as more evaluations are performed in the future.
Limitations of the present study include the relatively small sample size, the fact that it was a cadaver-based study, and the lack of inter- and intra-rater reliability data. Additionally, this study focused solely on tunnel position and did not assess other important operative factors, including duration of surgery, graft tensioning, and impingement, although the latter often occurs as a result of tunnel malpositioning. We also acknowledge that comparing novice surgeons with experienced surgeons may not accurately portray how computer-assisted surgery could aid resident surgeons in training or general orthopaedic surgeons unfamiliar with the double bundle technique. Clearly, there was a large gap in both general orthopaedic knowledge and surgical skill between the novice and experienced surgeon groups in this study. However, earlier studies had difficulty reaching a conclusion on the effectiveness of CAS compared to conventional surgery, and we sought to investigate the speculation expressed in those studies that CAS might provide more benefit to less experienced surgeons [Citation7, Citation34, Citation35]. We believe that the current study at least entertains such speculation and lays the groundwork for further investigation.
Strengths of this study include the use of multiple experienced surgeons trained at different institutions and at various stages in their careers. Most importantly, our study describes a novel technique for measuring tunnel location using MRI. Lorenz et al. [Citation11] modified the quadrant technique described by Bernard et al. [Citation4] to make measurements on CT images, allowing evaluation of the footprint in three planes on the lateral femoral condyle. While cross-sectional imaging using MRI does pose some challenges, particularly when evaluating tunnels that enter the bone obliquely rather than in an orthogonal fashion, in our experience, MRI is the most commonly used imaging modality when evaluating acute ACL injury or prior to revision surgery. We acknowledge that three-dimensional reconstruction using trace and volume fill functions may provide a more accurate and anatomic assessment of the tunnels, particularly as they travel through the bone. However, we sought to describe a technique for making measurements using MR images that would be applicable in the clinical setting using conventional picture archiving and communication systems (PACS) and associated software.
In conclusion, the present study found that novice surgeons using computer-assisted surgery systems placed tunnels on the tibial side with a similar degree of accuracy to experienced orthopaedic surgeons operating without the aid of computer navigation. It was also found that novice surgeons using computer-assisted surgery systems placed the femoral AM and PL tunnels in a significantly more anterior position along Blumensaat’s line compared to experienced surgeons operating without computer navigation. The study also reiterates the technical difficulty of placing anatomic femoral tunnels during double-bundle procedures for both novice and experienced surgeons alike. Lastly, we have described a novel, clinically applicable technique that allows measurement and evaluation of tunnel position on standard MR images.
Declaration of interest
Funding for this project was provided by the University of Iowa Carver College of Medicine and Aesculap Implant Systems in the form of an unrestricted research grant. The authors have no ongoing financial relationships that might cause a conflict of interest related to this study.
Acknowledgements
The authors would like to thank Yubo Gao for providing statistical support and for his continued dedication to the University of Iowa Department of Orthopaedics and Rehabilitation.
References
- Palmer I. 2007. On the injuries to the ligaments of the knee joint: a clinical study. 1938. Clin Orthop Relat Res 454:17–22; discussion 14
- Girgis FG, Marshall JL, Monajem A. 1975. The cruciate ligaments of the knee joint: Anatomical, functional and experimental analysis. Clin Orthop Relat Res 106:216–31
- Tallay A, Lim MH, Bartlett J. 2008. Anatomical study of the human anterior cruciate ligament stump’s tibial insertion footprint. Knee Surg Sports Traumatol Arthrosc 16:741–6
- Bernard M, Hertel P, Hornung H, Cierpinski T. 1997. Femoral insertion of the ACL. Radiographic quadrant method. Am J Knee Surg 10(1):14–21; discussion 21–22
- Colombet P, Robinson J, Christel P, et al. 2006. Morphology of anterior cruciate ligament attachments for anatomic reconstruction: A cadaveric dissection and radiographic study. Arthroscopy 22(9):984–92
- Doi M, Takahashi M, Abe M, et al. 2009. Lateral radiographic study of the tibial sagittal insertions of the anteromedial and posterolateral bundles of human anterior cruciate ligament. Knee Surg Sports Traumatol Arthrosc 17(4):347–51
- Edwards A, Bull AM, Amis AA. 2007. The attachments of the anteromedial and posterolateral fibre bundles of the anterior cruciate ligament. Knee Surg Sports Traumatol Arthrosc 15:1414–21
- Iriuchishima T, Tajima G, Shirakura K, et al. 2011. In vitro and in vivo AM and PL tunnel positioning in anatomical double bundle anterior cruciate ligament reconstruction. Arch Orthop Trauma Surg 131(8):1085–90
- Kopf S, Musahl V, Tashman S, et al. 2009. A systematic review of the femoral origin and tibial insertion of the ACL. Knee Surg Sports Traumatol Arthrosc 17:213–19
- Kuroda R, Matsushita T. 2011. Anatomic double-bundle anterior crucial ligament reconstruction with G-ST. Curr Rev Musculoskelet Med 4(2):57–64
- Lorenz S, Elser F, Mitterer M, et al. 2009. Radiologic evaluation of the insertion sites of the 2 functional bundles of the anterior cruciate ligament using 3-dimensional computed tomography. Am J Sports Med 37:2368–76
- Pietrini SD, Ziegler CG, Anderson CJ, et al. 2011. Radiographic landmarks for tunnel positioning in double-bundle ACL reconstructions. Knee Surg Sports Traumatol Arthrosc 19(5):792–800
- Siebold R, Ellert T, Metz S, Metz J. 2008. Tibial insertions of the anteromedial and posterolateral bundles of the anterior cruciate ligament: morphometry, arthroscopic landmarks, and orientation model for bone tunnel placement. Arthroscopy 24(2):154–61
- Stäubli HU, Rauschning W. 1994. Tibial attachment area of the anterior cruciate ligament in the extended knee position. Anatomy and cryosections in vitro complemented by magnetic resonance arthrography in vivo. Knee Surg Sports Traumatol Arthrosc 2(3):138–46
- Takahashi M, Doi M, Abe M, et al. 2006. Tibial insertions of the anteromedial and posterolateral bundles of human anterior cruciate ligament. Am J Sports Med 34:787–92
- Tanaka Y, Shiozaki Y, Yonetani Y, et al. 2011. MRI analysis of the attachment of the anteromedial and posterolateral bundles of anterior cruciate ligament using coronal oblique images. Knee Surg Sports Traumatol Arthrosc 19(Suppl 1):S54–9
- Tsukada H, Ishibashi Y, Tsuda E, et al. 2008. Anatomical analysis of the anterior cruciate ligament femoral and tibial footprints. J Orthop Sci 13:122–9
- Zavras T, Amos R, Amis AA. 2005. The effect of femoral attachment location on anterior cruciate ligament reconstruction: graft tension patterns and restoration of normal anterior-posterior laxity patterns. Knee Surg Sports Traumatol Arthrosc 13:92–100
- Getelman MH, Friedman MJ. 1999. Revision anterior cruciate ligament reconstruction surgery. J Am Acad Ortho Surg 7:189–98
- Abebe ES, Utturkar GM, Taylor DC, et al. 2011. The effects of femoral graft placement on in vivo knee kinematics after anterior cruciate ligament reconstruction. J Biomech 44(5):924–9
- Amis AA, Jakob RP. 1998. Anterior cruciate ligament graft positioning, tensioning and twisting. Knee Surg Sports Traumatol Arthrosc 6:S2–12
- Odensten M, Gillquist J. 1985. Functional anatomy of the anterior cruciate ligament and a rationale for reconstruction. J Bone Joint Surg Am 67:257–62
- Sommer C, Friederich NF, Müller W. 2000. Improperly placed anterior cruciate ligament grafts: correlation between radiological parameters and clinical results. Knee Surg Sports Traumatol Arthrosc 8(4):207–13
- Zantop T, Diermann N, Schumacher T, et al. 2008. Anatomical and nonanatomical double-bundle anterior cruciate ligament reconstruction: importance of femoral tunnel location on knee kinematics. Am J Sports Med 36(4):678–85
- Yagi M, Wong EK, Kanamori A, et al. 2002. Biomechanical analysis of an anatomic anterior cruciate ligament reconstruction. Am J Sports Med 30(5):660–6
- Yamamoto Y, Hsu WH, Woo SL, et al. 2004. Knee stability and graft function after anterior cruciate ligament reconstruction: A comparison of a lateral and anatomical femoral tunnel placement. Am J Sports Med 32:1825–32
- Plaweski S, Grimaldi M, Courvoisier A, Wimsey S. 2011. Intraoperative comparisons of knee kinematics of double-bundle versus single-bundle anterior cruciate ligament reconstruction. Knee Surg Sports Traumatol Arthrosc 19(8):1277–86
- Zantop T, Wellmann M, Fu FH, Petersen W. 2008. Tunnel positioning of anteromedial and posterolateral bundles in anatomic anterior cruciate ligament reconstruction: Anatomic and radiographic findings. Am J Sports Med 36:65–72
- Cheng T, Zhang GY, Zhang XL. 2012. Does computer navigation system really improve early clinical outcomes after anterior cruciate ligament reconstruction? A meta-analysis and systematic review of randomized controlled trials. Knee 19(2):73–7
- Endele D, Jung C, Becker U, et al. 2009. Anterior cruciate ligament reconstruction with and without computer navigation: a clinical and magnetic resonance imaging evaluation 2 years after surgery. Arthroscopy 25(10):1067–74
- Klos T, Habets R, Banks AZ, et al. 1998. Computer assistance in arthroscopic anterior cruciate ligament reconstruction. Clin Orthop Relat Res 354:65–9
- Mauch F, Apic G, Becker U, Bauer G. 2007. Differences in the placement of the tibial tunnel during reconstruction of the anterior cruciate ligament with and without computer-assisted navigation. Am J Sports Med 35(11):1824–32
- Plaweski S, Rossi J, Merloz P, Julliard R. 2011. Analysis of anatomic positioning in computer-assisted and conventional anterior cruciate ligament reconstruction. Orthop Traumatol Surg Res 97(6 Suppl):S80–5
- Meuffels DE, Reijman M, Verhaar J. 2012. Computer-assisted surgery is not more accurate or precise than conventional arthroscopic ACL reconstruction. J Bone Joint Surg Am 94:1–8
- Meuffels DE, Reijman M, Scholten R, Verhaar J. 2011. Computer assisted surgery for knee ligament reconstruction. Cochrane Database Syst Rev (6):CD007601
- Suomalainen P, Moisala AS, Paakkala A, et al. 2013. Comparison of tunnel placements and clinical results of single-bundle anterior cruciate ligament reconstruction before and after starting the use of double-bundle technique. Knee Surg Sports Traumatol Arthrosc 21(3):646–53
- Taketomi S, Nakagawa T, Takeda H, et al. 2011. Anatomical placement of double femoral tunnels in anterior cruciate ligament reconstruction: anteromedial tunnel first or posterolateral tunnel first? Knee Surg Sports Traumatol Arthrosc 19(3):424–31
- Snow M, Stanish WD. 2010. Double-bundle ACL reconstruction: how big is the learning curve? Knee Surg Sports Traumatol Arthrosc 18(9):1195–200