Abstract
Aim. The aim of this study was to investigate the effects of the bisphosphonate ibandronate (IBN) in a male osteoporosis animal model.
Methods. Two studies were performed in 9-month-old orchidectomised (ORX) or sham-operated rats. In prevention study, subcutaneous IBN was administered daily (1 μg/kg) or monthly (28 μg/kg every 28 days) starting on day of surgery for 5 months. In treatment study, the same treatment started 6 months after ORX. After sacrifice, bone analyses by dual-energy X-ray absorptiometry, 3-dimensional micro-computed tomography, and 3-point bending were performed in femora or vertebrae. Serum tartrate-resistant acid phosphatase 5b (TRAP-5b) and aminoterminal propeptide of collagen I (PINP) were analysed for resorption and osteocalcin (BGP) for bone formation.
Results. In both studies, ORX resulted in significant femoral and vertebral bone loss and microarchitectural deterioration after 5 months of ORX, and became more pronounced after 11 months. Biomechanical strength was also decreased. Serum levels for TRAP-5b and BGP increased while PINP levels were reduced or unchanged. Both daily and monthly IBN prevented or even restored ORX-induced changes in both studies, with the intermittent regimen showing a improvement in efficacy with respect to many of the biomechanical parameters.
Introduction
Postmenopausal osteoporosis is common worldwide. The disease is characterised by low bone mass, and microarchitectural deterioration of bone tissue, with a consequent increase in bone fragility and susceptibility to fractures [Citation1]. About every second women above the age of 50 will suffer from an osteoporosis-related fracture later in her life while the incidence in men above their 50th year is about half of that in women. About 20% of people affected by osteoporosis in the United States are men [Citation2].
Bisphosphonates are the most widely used medicine for the treatment and prevention of postmenopausal osteoporosis. Ibandronate is a highly potent nitrogen-containing bisphosphonate, which normalises increased bone turnover and prevents bone loss in postmenopausal women with low bone mass and increased risk for fractures. The drug is approved as once-monthly oral tablet and as a quarterly IV injection for the treatment and prevention of postmenopausal osteoporosis in the US and for treatment in EU [Citation3].
Osteoporosis in men develops later in life compared with women. In fact age is the main, but not the unique, cause for osteoporosis development in men. Although the incidence of osteoporosis-related fractures is lower in men, the morbidity and mortality associated with osteoporotic fractures are much greater in men compared with women. In addition secondary causes of osteoporosis are more frequently (in approximately 50% of cases) identified in men compared with women with osteoporosis [Citation4]. Some bisphosphonates have already demonstrated efficacy in the treatment of male osteoporosis [Citation5,Citation6], however limited data are available about the effect of ibandronate in this disease. In particular, only a few studies investigated the effect of ibandronate in men suffering from secondary osteoporosis such as corticosteroid-induced osteoporosis [Citation7] or bone loss following organ transplantation [Citation8]. Also, one study in androgen deficiency-related bone loss in patients with Klinefelter syndrome has been published [Citation9]. Recently, a study in male patients with primary, idiopathic or hypogonadal osteoporosis was conducted with ibandronate [Citation10].
For the submission and approval of new medicines, preclinical studies on the intended indication, such as osteoporosis, are requested by drug regulatory authorities [Citation11–13 ]. The extensive investigation of ibandronate in animal models simulating postmenopausal osteoporosis is fully aligned with these authority expectations, and provided the basis for a variety of successful clinical trials, and consequently for the approval of this drug in many countries (for reviews see [Citation14–16 ]). Although no World Health Organization (WHO) definition for osteoporosis in men exists, the recent update of Committee for Medicinal Products for Human Use (CHMP) guidelines acknowledges the high similarity of clinical features in male and female osteoporosis and offers minimal requirements for clinical studies in male osteoporotic patients provided the tested drug has no gender-specific mode of action, and is extensively investigated in relevant animal models [Citation13]. The WHO specifically lists orchidectomy as an appropriate method to induce bone loss in experimental animals to appropriately simulate male osteoporosis [Citation17]. Consequently, the present study investigates the effects of ibandronate in aged androgen-deficient rats with regard to bone mass, architecture, biochemical markers of bone turnover and biomechanical properties. Daily or intermittent intervention was commenced either directly after orchidectomy (prevention) or when bone loss has already been established (treatment).
Materials and methods
Animals
One hundred twenty male Wistar rats, 9 months old, weighing 498.2 ± 60.9 g (mean ± SD), at the start of the study, were used. At this age, rats were sexually mature and fully grown. Animals were then orchidectomised (ORX) or sham-operated (SHAM) under Ketamine (40 mg/kg, Ketolar, Bayer) and Xilacine (8 mg/kg, Rompun, Parke-Davis, Pfizer), and distributed into two studies. The animals were kept under constant living conditions (22°C, 12 h per day light-dark cycles), and food (standard Laboratory chow) and water were available ad libitum.
Treatment regimens
Two studies were performed: one study on the prevention of orchidectomy-induced bone loss, and one study on the treatment of established orchidectomy-induced osteopenia.
Prevention study.
Forty-eight rats were randomised in the following groups: three orchidectomised (ORX) groups (n = 12 each) and one sham-operated group (SHAM, n = 12). Animals were administered saline daily for 20 weeks (SHAM and one ORX group); or with ibandronate at a daily dose of 1 μg/kg body weight for 20 weeks (ORX + dIBN, n = 12), or with ibandronate at a dose of 28 μg/kg administered every 28 days (monthly) for 20 weeks (ORX + mIBN, n = 12). Ibandronate treatment was started immediately after surgery. Rats were sacrificed at the end of the 5 months treatment period.
Treatment study.
Seventy-two rats were randomised in the following groups: SHAM group sacrificed 6 months after sham surgery (SHAM1, n = 12), orchidectomised group sacrificed 6 months after ORX (ORX1, n = 12), SHAM group administered daily with saline for 20 weeks starting 6 months after sham surgery (SHAM2, n = 12), ORX group treated with saline for 20 weeks starting 6 months after surgery (ORX2, n = 12) and finally, ORX group treated with ibandronate for 20 weeks at a daily dose of 1 μg/kg body weight starting 6 months after surgery (ORX2 + dIBN, n = 12) and ORX group treated with ibandronate for 20 weeks at a dose of 28 μg/kg administered monthly, i.e. every 28 days (ORX + mIBN, n = 12).
Both, ibandronate and saline were administered subcutaneously. Ibandronate was supplied by Roche Diagnostics GmbH (Mannheim, Germany). Ibandronate [1 hydroxy-3-(methylpentylamino)propylidene] bis-phosphonic acid (monosodium salt monohydrate) was dissolved in isotonic saline and the pH-value was adjusted to 7.4. The solution was freshly prepared, sterile filtered and stored in a normal refrigerator (4°C) throughout the study period. The doses are expressed as μg/kg of free acid equivalents of ibandronate. The volume of administration was 2 ml/kg.
One day following the last treatment, the animals were sacrificed by exsanguination under inhalation anaesthesia. Blood samples were obtained by cardiac puncture and serum samples were immediately frozen at −80°C as aliquots for determination of biochemical markers of bone turnover. Once the blood was collected, animals were frozen at −20°C until determination of femoral bone mineral density (BMD) in previously thawed animals (2 days at −4°C). Prior to BMD analyses, the left femurs were excised and cleaned of adjacent tissue. The right femur was also excised and cleaned for computerised microtomographic analysis (μCT) and determination of biomechanical properties lumbar spine BMD was determined in situ and thereafter. Lumbar vertebrae L4 were separated from the spine and carefully cleaned of adjacent tissue for μCT analyses. Both, right femur and L4 vertebrae were again frozen in sodium chloride solution at −20°C until μCT and biomechanical assays were performed. Repeated freeze-thaw cycles have been shown to not influence mechanical properties of bone [Citation18].
All procedures were carried out in accordance with European Community Standards on the Care and Use of Laboratory Animals.
Bone mineral density
BMD was determined in situ in the lumbar spine (L2, L3 and L4) and in the whole left femur by dual energy X-ray absorptiometry (DEXA) using a HOLOGIC QDR-1000 TM (S/N 277) (Hologic, Inc., Waltham, MA) with small-animal software [Citation19]. Intra-assay and inter-assay variation coefficients were <0.53% and <1.2%, respectively. The scans of the femur were analysed for BMD of the whole femur and the scans of the L2, L3 and L4 vertebrae were analysed for BMD of the whole three vertebrae and results were expressed as the mean of the values obtained.
3D trabecular microarchitecture analysis of femur and vertebrae by microCT
Distal region of right femur and L4 vertebral body were analysed by μCT (Skyscan N.V., Aartselaar, Belgium), imaged both with an X-ray tube voltage of 100 kV and current of 100 μA, and with a 1.0 mm aluminium filter. The scanning angular rotation was 185°, and the angular increment was 0.45°. The voxel size was 11.0 μm and 13.0 μm, respectively. Data sets were reconstructed using a modified Feldkamp algorithm [Citation20] and segmented into binary images (8 bit BMP images) using adaptive local thresholding. For analysis of the microarchitectural properties of trabecular and cortical bone regions, femora specimens were evaluated within a conforming volume of interest (VOI). In the case of the trabecular bone femur region, a VOI was selected starting at a distance of 1.00 mm from the growth plate, and extending a further longitudinal distance of 2.50 mm in the proximal direction (226 image slices analysed, cortical bone excluded). For the analysis of microarchitectural properties of trabecular vertebrae body, the VOI was determined for the entire region of the secondary spongiosa, excluding the cortical surfaces.
Both trabecular and cortical bone regions were obtained by free drawing regions of interest, and analysed using the commercial software provided with the equipment (SkyScan™ CT-analyser software, version 1.7.0). Morphometric indices of trabecular bone region were determined from the microtomographic data sets (integrated over a VOI) using direct 3D morphometry. Total volume of VOI (tissue volume TV, mm3) and trabecular bone volume (BV; mm3) were calculated based on the hexahedral marching cubes volume model of the VOI. Trabecular bone volume (BV/TV; %) was directly calculated. Trabecular thickness (Tb.Th; mm), trabecular separation (Tb.Sp; mm) and trabecular number (Tb.N; 1/mm) were measured directly on 3D images using methods previously described [Citation21,Citation22]. Measurements of trabecular thickness were calibrated by scanning and analysing three aluminium foils with thicknesses of 50, 125 and 250 μm. The non-metric indices, structure model index (SMI), and trabecular bone pattern factor (Tb.Pf; 1/mm), were also calculated using the direct 3D model. The SMI parameter indicates the relative prevalence of rods and plates in a 3D structure [Citation23]. The trabecular bone pattern factor (Tb.Pf; 1/mm) measures the relative convexity or concavity of the total bone surface [Citation24]. The degree of anisotropy (DA) represents trabecular anisotropy, defined as the ratio between the maximal and minimal radius of the mean intercept length [Citation25].
The coefficient of variation (%CV) values for all these measurements were <5%.
Femur biomechanical testing.
Frozen and thawed right femora were applied to mechanical testing with a Microtest EM1/10/FR/m testing machine (Microtest, S.A., Madrid, Spain). Three-point bending strength was measured with a constant span length of 15 mm. The press head as well as the two support points were rounded to avoid shear load and cutting [Citation26,Citation27]. The bone was positioned horizontally with the anterior surface upwards, centred on the supports, and the pressing force was directed vertically to the midshaft of the bone. Each bone was compressed with a constant load of 3 kN/s until failure. The load-displacement curve obtained was converted into a stress–strain curve.
Whole bone extrinsic biomechanical properties reported include ultimate force (Fult), ultimate displacement (δult) and extrinsic stiffness (S). On the other hand, bone tissue intrinsic biomechanical properties include ultimate stress (σult), ultimate strain (ϵult) and Young's modulus (E) [Citation28].
Fult was defined as bending load at failure, and δult was the deformation at this point. σult and ϵult were defined as stress and strain at breaking force. Using beam bending theory and assuming that bone has a linear elastic behaviour, the quantities of σ, ϵ, E and S were determined.
Biochemical markers of bone turnover
Serum Bone Gla Protein (BGP) was determined by ELISA for the specific quantitative determination of rat osteocalcin levels (Rat-MID Osteocalcin, IDS, UK). Sensitivity of this assay was 50 ng/ml. Intra- and inter-assay coefficients of variation of the method were <5.0% and <6.6%, respectively.
Serum 5b isoenzyme of tartrate-resistant acid phosphatase (TRAP) was measured by an ELISA specific for rat TRAP (RatTRAP Assay, IDS, UK). Sensitivity of the assay was 0.1 U/l. Intra- and inter-assay variation coefficients of the method were <5.0% and <5.5%, respectively.
Serum aminoterminal propeptide of collagen I (PINP) was assayed by an ELISA specific for rat PINP (Rat/Mouse PINP EIA, IDS, UK). Sensitivity of the assay was 0.7 ng/ml. Intra- and inter-assay variation coefficients of the method were <5.0% and <8.2%, respectively.
Statistical analyses
The results of the experiments were expressed as the mean ± SD of the different parameters. A non-parametric method, Mann–Whitney test (Medcalc Software Program, Belgium), was used to compare the different treatment groups. A p-value <0.05 was accepted as denoting a significant difference.
Results
Prevention study
Treatment effects on bone mineral density.
BMD decreased in both in femora and vertebra after 5 months following orchidectomy, however only changes in femora have reached statistical significance (p < 0.05). Daily or monthly treatment with ibandronate (same total cumulative dose) prevented this bone loss completely reaching values close to age-matched SHAM controls ().
Figure 1. BMD (g/cm2) measured (a) in femur (FBMD) and (b) in lumbar vertebra (LBMD); Nine-month-old male Wistar rats at the beginning of the study, distributed into two groups. Prevention study formed by sham-operated rats (SHAM), castrated rats (orchidectomy: ORX) and castrated rats treated for 20 weeks with IBN 1 μg/kg/day (ORX + dIBN) and IBN 28 μg/kg/28 days (ORX + mIBN), beginning immediately after surgery. Treatment study formed by sham-operated rats (SHAM1) and castrated rats (ORX1) killed 6 months after surgery as a baseline; and sham-operated rats (SHAM2), castrated rats (ORX2), and castrated rats treated for 20 weeks with IBN 1 μg/kg/day (ORX2 + dIBN) and IBN 28 μg/kg/28 days (ORX2 + mIBN), beginning 6 months after surgery. Data are expressed as mean ± SD of 12 animals/group. Statistical significance p < 0.05: avs. SHAM/SHAM1/SHAM2; bvs. ORX/ORX1/ORX2; cvs. ORX + dIBN/ ORX1 + dIBN/ORX2 + dIBN.
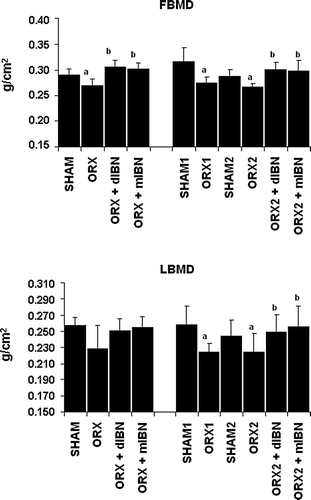
Treatment effects on bone microarchitecture.
Orchidectomy induced a significant decrease in trabecular bone volume BV/TV in the distal femora at 5 and 6 months after ORX (p < 0.05). Both regimens of IBN treatment prevented the androgen-lack related decrease in this parameter. This decrease in BV/TV was accompanied by an increase in trabecular separation (Tb.Sp) and by a decrease in trabecular number (Tb.N.). Daily and monthly IBN treatments prevented these changes in ORX rats, showing similar values of BV/TV, Tb.Sp and Tb.N in treated animals similar to those in the SHAM group. Structural modelling index (SMI) was also significantly increased in ORX rats (p < 0.05), showing an internal-structure bone tending to bars and with fewer rods. Both daily and monthly IBN showed a positive effect by keeping this parameter close to that of the SHAM group. Trabecular pattern factor (Tb.Pf) also increased due to orchidectomy, indicating a less number of trabecular connectivity. Daily- or monthly-IBN treatment prevented this effect observed in orchidectomised rats. The DA did not reflect any changes neither in ORX rats nor treated-ORX rats with respect to SHAM group. Details on femoral microarchitecture parameters are shown in and and .
Figure 2. Representative images of bone trabecular 3D microarchitecture in femur sections of the prevention study groups obtained by computed microtomography. Prevention study formed by sham-operated rats (SHAM), castrated rats (orchidectomy: ORX) and castrated rats treated for 20 weeks with IBN 1 μg/kg/day (ORX + dIBN) and IBN 28 μg/kg/28 days (ORX + mIBN), beginning immediately after surgery.
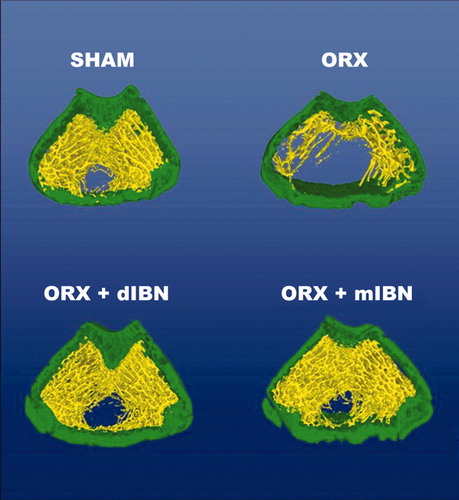
Figure 3. Femoral trabecular microarchitecture analysis: bone volume/total volume (BV/TV), trabecular number (TbN), trabecular separation (TbS) and Trabecular pattern factor. Nine-month-old male Wistar rats at the beginning of the study, distributed into two groups. Prevention study formed by sham-operated rats (SHAM), castrated rats (orchidectomy: ORX) and castrated rats treated for 20 weeks with IBN 1 μg/kg/day (ORX + dIBN) and IBN 28 μg/kg/28 days (ORX + mIBN), beginning immediately after surgery. Treatment study formed by sham-operated rats (SHAM1) and castrated rats (ORX1) killed 6 months after surgery as a baseline, and sham-operated rats (SHAM2), castrated rats (ORX2), and castrated rats treated for 20 weeks with IBN 1 μg/kg/day (ORX2 + dIBN) and IBN 28 μg/kg/28 days (ORX2 + mIBN), beginning 6 months after surgery. Data are expressed as mean ± SD of 12 animals/group. Statistical significance p < 0.05: avs. SHAM/SHAM1/SHAM2; bvs. ORX/ORX1/ORX2; cvs. ORX + dIBN/ ORX1 + dIBN/ORX2 + dIBN.
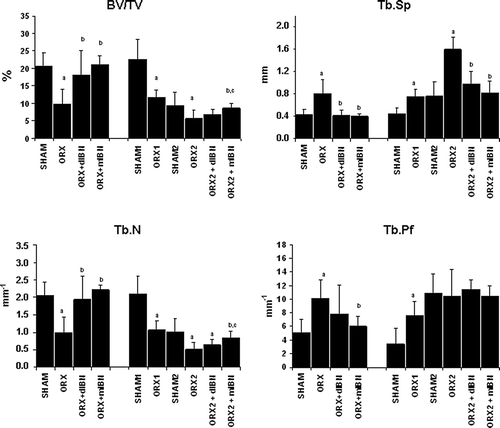
Table I. Femoral Structure Model Index (SMI) and degree of anisotropy (DA).
In contrast to lumbar spine BMD, there was a significant decrease in vertebral BV/TV in the ORX control group, and this was avoided with daily and monthly IBN treatment. Tb.N was significantly decreased in ORX rats, with no changes in Tb.Sp. The two IBN regimens prevented this decrease in Tb.N, maintaining values of Tb.Sp similar to the SHAM group. No significant changes were detected for SMI, Tb.Pf and DA in lumbar spine of untreated-ORX and treated-ORX groups ().
Table II. Trabecular microarchitecture analysis of vertebrae.
Treatment effects on biomechanical properties.
shows extrinsic (structural) femoral properties (ultimate force, ultimate displacement and extrinsic stiffness) and intrinsic (material) femoral properties (ultimate stress, ultimate strain and apparent Young modulus) of all the studied groups. Orchidectomy produced a significant increase in ultimate displacement and ultimate strain, and a decrease in extrinsic stiffness and apparent Young modulus with respect to their respective SHAM group. Ibandronate treatment in the prevention study avoided changes in ultimate displacement in monthly and daily treated groups and produced a significant increase in ultimate force, extrinsic stiffness, ultimate stress and apparent Young modulus in the monthly treated (ORX + IBNm) group with respect to the SHAM group, being these values significantly higher than those of the daily treated group.
Table III. Femoral extrinsic (structural) and intrinsic (material) properties of control, orchidectomized orchidectomised and ibandronate treated rats.
Treatment effects on biochemical markers of bone turnover.
Five months after orchidectomy, ORX rats showed an significant increase in serum levels for BGP and a significant decrease for PINP (p < 0.05) without significant differences in TRAP levels with respect to sham group. Both, daily and monthly IBN treatment decreased BGP and TRAP-5b levels to values below those of the SHAM group. On the other hand, daily IBN treatment maintained low levels of PINP in ORX controls, and monthly IBN treatment decreased PINP levels below those of ORX controls and ORX + dIBN ().
Table IV. Biochemical markers of bone turnover.
Treatment study
Treatment effects on bone mineral density.
As expected, 11 months after orchidectomy, ORX rats (ORX2) presented a significant decrease in femoral BMD and lumbar spine BMD (). Daily and monthly treatment with IBN for 20 weeks (ORX2 + dIBN and ORX2 + mIBN), restored BMD levels in both the ORX groups to levels similar to the SHAM control group (SHAM2) (Figure1).
Treatment effects on microarchitecture.
Six months after surgery, ORX rats (ORX1) presented low values for femoral BV/TV and Tb.N, with an increase in Tb.Sp. SMI and Tb.Pf. DA did not reflect any change ( and ). Twenty weeks later, solvent-treated orchidectomised animals (ORX2) showed low levels for BV/TV and Tb.N, and a significant increase in Tb.Sp (p < 0.05), with no changes in SMI, Tb.Pf or DA. Daily treatment with IBN starting 6 months after surgery recovered BV/TV to values similar to SHAM controls (SHAM2) and without significant differences to ORX2. However, monthly IBN treatment recovered BV/TV values reaching similar values to those of the age-matched SHAM control (SHAM2), being significantly higher than values for ORX2 controls and ORX2 + dIBN group (p < 0.05).
Both regimens of IBN treatment, daily and monthly dosing, recovered values for Tb.Sp to those of the SHAM controls (SHAM2). Monthly (ORX2 + mIBN), but not daily (ORX2 + dIBN) treatment, restored Tb.N values up to values of the SHAM group (SHAM2). Neither daily nor monthly treatment produced detectable changes in SMI or Tb.Pf in femur, but monthly treatment produced a decrease in DA to values below those of the SHAM group ( and and ).
Figure 4. Representative images of bone trabecular 3D microarchitecture in femur sections of the treatment study groups obtained by computed microtomography. Treatment study formed by sham-operated rats (SHAM2), castrated rats (ORX2) and castrated rats treated for 20 weeks with IBN 1 μg/kg/day (ORX2 + dIBN) and IBN 28 μg/kg/28 days (ORX2 + mIBN), beginning 6 months after surgery.
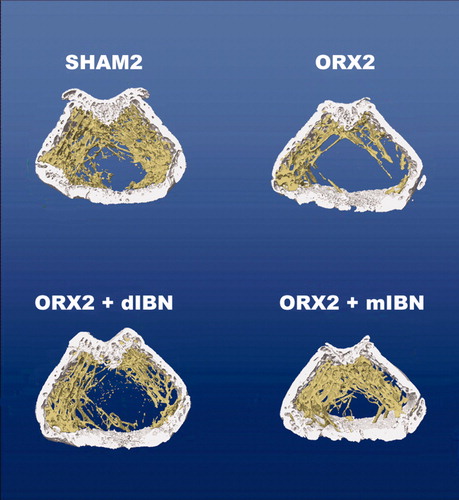
Six months after orchidectomy rats decreased their vertebral BV/TV values and Tb.N without any significant differences in the other analysed parameters related to trabecular microarchitecture. As expected, 5 months later, values for vertebral BV/TV were lower in ORX2 group than in SHAM2. No significant differences were observed in the other parameters between these two groups. Both, daily and monthly treatment with ibandronate for 5 months restored vertebral BV/TV levels to those of the SHAM group ().
Treatment effects on biomechanical properties.
Ibandronate treatment recovered levels of SHAM2 group in extrinsic stiffness and ultimate strain, being values of ultimate force higher than those of SHAM2 group in daily and monthly treatment and values of ultimate stress and apparent Young modulus in the monthly treatment higher than those of SHAM2 group and also higher than those of the daily treated group.
Treatment effect on biochemical markers of bone turnover.
Six months after orchidectomy, ORX rats (ORX1) presented high levels of BGP and TRAP-5b, and low levels of PINP with respect to levels in SHAM1 controls (Table 1V). Eleven months after orchidectomy, untreated ORX rats (ORX2) maintained the elevated levels of BGP, but TRAP-5b levels returned to normal values while PINP levels remained considerably unchanged. Daily and monthly IBN treatment, starting 6 months after orchidectomy, significantly decreased TRAP-5b and BGP levels with respect to ORX2 and SHAM2 groups. Daily treatment with IBN maintained the low levels of PINP as observed in ORX rats (ORX2) while those following monthly treatment further decreased ().
Discussion
Animal models reflecting the respective cause of osteoporosis are valuable and accepted tools to study osteoporosis development and the effects of treatments. The orchidectomised rat model constitutes a validated experimental model to simulate male osteoporosis [Citation17,Citation29,Citation30]. In particular the aged rat model, using animals at the end of their growth phase, represents a valuable model since almost all growth-related effects on bone, as it the case with younger rats, can be excluded. Consequently, the present study used 9 months-old androgen-deficient male rats to investigate the effects of daily and intermittent treatment of ibandronate in preventive and curative settings. Doses and treatment schedules are based on efficacy data published for aged ovariectomised rats [Citation31,Citation32].
Prevention study
Androgen deprivation in ORX rats lead to a significant decrease in trabecular BV/TV in femur and vertebrae whereas significance for BMD was only reached in femur but not in lumbar spine. This is in line with previously reported data in mature ORX rats indicating a higher sensitivity of 2-dimensional histomorphometryic analyses for trabecular bone, when compared to whole-bone DEXA [Citation33]. Besides the differences in method-specific sensitivity, the main reason for this finding seems to include the impact of trabecular bone, which is more prominent in whole vertebrae than in distal femur, thus dominating BMD in lumbar spine.
Daily and monthly ibandronate treatment prevented the decrease of femoral and lumbar BV/TV, and prevents the increase in Tb.Sp and the decrease in Tb.N. In the case of femur, the increase in Tb.Pf and SMI, and the decrease in DA, as observed in ORX rats, were avoided by ibandronate treatment, resulting in similar effects of the two administration schedules (daily and monthly). In rats, the half-life of ibandronate in female rat bone is estimated to be 440–500 days [Citation31]. As a consequence of this long half-life, ibandronate accumulates in bone in a dose-dependent manner when given by repeated administration over extended periods [Citation15,Citation33]. The concentration of ibandronate in bone was similar when daily or intermittent treatment schedules were applied, provided the cumulative doses are the same [Citation34]. For this reason, ibandronate efficacy is mainly determined by cumulative doses, rather than by the frequency of administration [Citation31,Citation34]. Thus, treatment with ibandronate provided equivalent results in male ORX rats as described for female ovariectomised rats [Citation15,Citation34], and confirms the total dose concept for both genders.
With respect to biochemical properties, there is an important difference between the mechanical properties of bone tissue as ‘material’ and those of the whole bone as a structure. This is the reason of the classification in extrinsic or structural properties and intrinsic or material properties. When a force is applied, bone suffers a displacement or deformation. This relationship between applied force and displacement characterises the structural properties (extrinsic). However, the material properties of the sample reflect the intrinsic characteristics of bone tissue itself. Generally, fractures are the result of material fails in bone tissue that leads to a mechanical fail of the whole bone. For this reason, in the evaluation of the effects of a treatment on bone resistance, it is important to analyse both extrinsic and intrinsic properties. Extrinsic properties come from the load-displacement curve and intrinsic from stress–strain curve.
In the present work, we found that ibandronate treatment avoided many of the changes in biomechanical parameters produced by orchidectomy and it is important to note that in some of these parameters (ultimate force, extrinsic stiffness, ultimate stress, ultimate strain and apparent Young modulus) levels got through monthly treatment were still better than those got with the daily treatment.
With respect to serum levels for biochemical markers of bone turnover, our results are in line with those of others, who described increased serum BGP levels in 13-month-old ORX Fischer 344 rats starting 2 weeks after surgery [Citation29]. For bone resorption markers, the same authors reported a rapid and sustained increase in urinary excretion of deoxypyridoline compared with SHAM controls. In our study, serum levels for TRAP-5b were increased 5 months after castration when compared to age-matched SHAM controls. The increase in bone formation (BGP) and resorption (TRAP-5b) indicates a general increase in bone remodelling in ORX rats as it had also been demonstrated for osteoclast number, osteoblast surface, bone-formation rate and activation frequency, which all were increased in ORX animals after 1 month post surgery until the end of other studies [Citation29]. Other authors [Citation35], however, found a transient increase of turnover 1 month after ORX in 13-month-old rats, with a return to normal values 4 months later. When compared to these previous studies, and to our finding of an increase in BGP, which is regarded to also reflect bone turnover rather than pure bone formation, the decrease in PINP levels in ORX rats seems to more appropriately reflect pure bone formation. Due to the fact that in our study, levels of PINP have been determined only 5 months after castration, it might be possible that there would have been an early but transient increase in this marker, which was reduced below that of SHAM controls after 5 months, thus demonstrating different turnover kinetics between bone formation and resorption. Recently, significantly increased PINP levels were described during the first 2 weeks after ovariectomy in rats, which returned to SHAM levels at 8 weeks [Citation36]. Daily and monthly preventive treatment with ibandronate for 20 weeks decreased the levels of biochemical markers of bone remodelling, both formation and resorption, even below those of the SHAM control group. Since there is no study describing PINP levels in ORX rats so far, a more detailed kinetic on changes in this parameter needs to be studied in this model.
Treatment of established bone loss.
Daily or monthly treatment with ibandronate, for 20 weeks starting 6 months after ORX, decreased BGP, TRAP-5b and PINP to levels even lower than that of the SHAM group, and also recovered femoral and lumbar BMD to levels of intact animals, without differences between daily or monthly administrations. In the case of trabecular microarchitecture, monthly treatment significantly exceeded the results for daily treatment, because monthly treatment completely overcompensated bone loss as indicated by BV/TV, Tb.N and trabecular separation. So, although femoral and lumbar BMD are restored by daily or monthly treatment, some differences were observed when microarchitecture was compared.
Previous studies in rats and dogs comparing continuous and intermittent treatment schedules with ibandronate indicate similar efficacy when the same accumulative dose was applied over the duration of the study [Citation31]. In the present work, daily and monthly ibandronate administration produced the same beneficial effects on bone mass in the prevention study, when administered immediately after orchidectomy. However, when ibandronate administration restored lost bone, monthly administration was slightly more efficient than daily as can be observed in biomechanical parameters. A similar effect was described in human postmenopausal osteoporosis, in which 1-year once-monthly oral ibandronate therapy resulted in a significantly larger proportion of women achieving predefined changes in lumbar spine or total hip BMD than those with daily treatment [Citation37]. This effect continued in the second year of the study, with once-monthly 150 mg ibandronate consistently producing larger increases in BMD and stronger CTX suppression than the daily regimen [Citation38].
What could be the reason why 1 μg/kg/day of preventive ibandronate administration completely prevented bone loss while the same daily dose given at established bone loss is not able to completely recover bone mass? One of the reasons could be the different baseline status of bone mass and turnover, suggesting a benefit for treatment initiation as early as possible. Of greater importance might be the different remodelling rate at treatment initiation in the two experiments. In 13-month-old rats a transient increase in bone turnover was described 1 month after ORX, with a return to normal values 4 months later [Citation35]. Although BGP levels are still increased at the end of the study in 19-month-old ORX rats, values for TRAP are in the normal range, indicating a decrease in bone remodelling. In another recent study performed in our laboratory with male orchidectomised rats, 12-month-old Wistar rats, orchidectomised 3 months before, showed a significant greater number of tetracycline bound surfaces than 18-month-old rats orchidectomised 9 months before, indicating a significantly higher bone remodelling rate at 3 months after orchidectomy than at 9 months after surgery (unpublished results). Therefore, the activation status of osteoclasts, which are the target cells for bisphosphonates, seems to play an important role. This conclusion is in line with data obtained from ovariectomised beagle dogs, demonstrating that osteoclasts with high metabolic activity are more receptive to direct or indirect effects of bisphosphonates than osteoclasts during their steady state [Citation39].
With respect to biomechanical parameters, as in the case of preventive treatment, ibandronate treatment to osteopenic rats recovers or overcompensates the most important changes in biomechanical parameters produced by orchidectomy and also, in some of these parameters (ultimate stress, ultimate strain and apparent Young modulus) levels got with monthly treatment were still better than those got with the daily treatment.
The reasons for these results remain to be elucidated, in particular since bone strength was only maintained in ibandronate-treated ovariectomised aged rats [Citation34]. Our results show that monthly dosing was overall more effective than daily treatment. The reason for this finding might be explained by the higher drug exposure at the intermittent administration days, resulting in a more potent suppression of bone resorption and thus inducing stronger transient positive effects allowing relatively more bone to be formed during the treatment-free periods.
Nevertheless, the results of the present study confirm the overall positive effects of ibandronate administered monthly on bone quality in animal studies [Citation15].
To our knowledge, this is the first study using ibandronate in the treatment of bone loss due to androgen deficiency in rats. Overall, we can conclude that ibandronate treatment, administered daily or once-monthly prevents bone loss or restores bone in orchidectomised aged rats, with monthly treatment being slightly more effective than daily. Although it is not possible to extrapolate the results achieved in animals directly to humans, in particular with respect to dose and intervals, our results at least match those obtained in aged oestrogen-depleted rats used as a model in postmenopausal osteoporosis. In addition, pilot studies in humans [Citation10,Citation40] confirm that intermittent ibandronate is a promising treatment option for male osteoporosis. In the work of Lamy et al. [Citation40], 40 men with primary osteoporosis received 2 mg of ibandronate i.v. every 3 months for 2 years. BMD increased at lumbar spine and femoral neck and CTX and BGP decreased significantly, showing that this intermittent regimen was adequated for the treatment of male osteoporosis. The recent work published by Orwoll et al. [Citation10] studies 87 men with low BMD who were treated for 1 year with once-monthly 150 mg oral ibandronate (also intermittent treatment). In treated patients increased BMD at the lumbar spine and hip, and percent decreases in CTX and bone alkaline phosphatase were greater from baseline versus placebo. Ibandronate was generally well tolerated.
Acknowledgement
This work was supported by a grant from F. Hoffmann La Roche (Basle, Switzerland).
References
- National Institute of Health. Osteoporosis prevention, diagnosis, and therapy. NIH Consens Statement 2000;17:1–36.
- Melton LJ, Atkinson EJ, O'Connor MK, O'Fallon WM, Riggs BL. Bone density and fracture risk in men. J Bone Miner Res 1998;13:1915–1923.
- Bauss F, Schimmer RC. Ibandronate: the first once-monthly oral bisphosphonate for treatment of postmenopausal osteoporosis. Ther Clin Risk Manage 2006;2:3–18.
- Kamel HK. Male osteoporosis: new trends in diagnosis and therapy. Drugs Aging 2005;22:741–748.
- Rackoff P. Efficacy and safety of risedronate 150 mg once a month in the treatment of postmenopausal osteoporosis. Clin Interv Aging 2009;4:207–214.
- Piper PK, Gruntmanis U; Management of osteoporosis in the aging male: focus on zoledronic acid. Clin Interv Aging 2009;4:289–303.
- Ringe JD, Dorst A, Faber H, Ibach K, Sorenson F. Intermittent intravenous ibandronate injections reduce vertebral fracture risk in corticosteroid-induced osteoporosis: results from a long-term comparative study. Osteoporos Int 2003;14:801–807.
- Fahrleitner-Pammer A, Piswanger-Soelkner JC, Pieber TR, Obermayer-Pietsch BM, Pilz S, Dimai HP, Prenner G, Tscheliessnigg KH, Hauge E, Portugaller RH, Ibandronate prevents bone loss and reduces vertebral fracture risk in male cardiac transplant patients: a randomized double-blind, placebo-controlled trial. J Bone Miner Res 2009;24:1335–1344.
- Stepan JJ, Burckhardt P, Hána V. The effects of three-month intravenous ibandronate on bone mineral density and bone remodeling in Klinefelter's syndrome: the influence of vitamin D deficiency and hormonal status. Bone 2003;33:589–596.
- Orwoll ES, Binkley NC, Lewiecki EM, Gruntmanis U, Fries MA, Dasic G. Efficacy and safety of monthly ibandronate in men with low bone density. Bone 2010;46:970–976.
- Rockville, MD; Food and Drug Administration. United States Food and Drug Administration Guidelines for preclinical and clinical evaluation of agents used in the prevention or treatment of postmenopausal osteoporosis; 1994
- Committee for Proprietary Medicinal Products (CPMP). Note for guidance on involutional osteoporosis in womenLondon: The European Agency for the Evaluation of Medicinal Products; Human Medicines Evaluation Unit; 2001.
- Committee for Medicinal Products for Human use (CHMP). Guideline for the evaluation of medicinal products in the treatment of primary osteoporosisLondon: European Medicines Agency Human Medicines Evaluation Unit; 2006.
- Bauss F, Russell RGG. Ibandronate in osteoporosis: preclinical data and rationale for intermittent dosing. Osteoporos Int 2004;15:423–433.
- Bauss F, Dempster DW. Effects of ibandronate on bone quality: preclinical studies. Bone 2007;40:265–273.
- Hothorn LA, Bauss F. Biostatistical design and analyses of long-term animal studies simulating human postmenopausal osteoporosis. Drug Inf J 2004;38:47–56.
- World Health Organization. Guidelines for preclinical evaluation and clinical trials in osteoporosis. Geneva: WHO; 1998.
- Borchers RE, Gibson LJ, Burchardt H, Hayes WC. Effects of selected thermal variables on the biomechanical properties of trabecular bone. Biomaterials 1995;16:545–551.
- Gala J, Díaz Curiel M, De la Piedra C, Castilla C, Torralbo M. Bone mass assessment in rats by dual energy X-ray absorptiometry. Br J Radiol 1998;71:754–758.
- Feldkamp LA, Davis LC, Kress JW. Practical cone-beam algorithm. J Opt Soc Am A 1984;1:612–619.
- Hildebrand T, Ruegsegger P. A new method for the model-independent assessment of thickness in three-dimensional images. J Microsc 1997;185:67–75.
- Ulrich D, Van RB, Laib A, Ruegsegger P. The ability of three-dimensional structural indices to reflect mechanical aspects of trabecular bone. Bone 1999;25:55–60.
- Hildebrand T, Ruegsegger P. Quantification of bone microarchitecture with the Structure Model Index. Comput Methods Biomech Biomed Eng 1997;1:15–23.
- Harrigan TP, Mann RW. Characterisation of microstructural anisotropy in orthotropic materials using a second rank tensor. J Mater Sci 1984;19:761–767.
- Hahn M, Vogel M, Pompesius-Kempa M, Delling G. Trabecular bone pattern factor, a new parameter for simple quantification of bone microarchitecture. Bone 1992;13:327–330.
- Jamsa T, Jalovaara P, Peng Z, Vaananen HK, Tuukkanen J. Comparison of threepoint bending test and peripheral quantitative computed tomography analysis in the evaluation of the strength of mouse femur and tibia. Bone 1998;23:155–161.
- Saffar KP, JamilPour N, Rajaai SM. How does the bone shaft geometry affect its bending properties? Am J Appl Sci 2009;6:463–470.
- Turner CH. Biomechanics of bone: determinants of skeletal fragility and bone quality. Osteoporos Int 2002;13:97–104.
- Erben RG, Eberle J, Stahr K, Goldberg M. Androgen deficiency induces high turnover osteopenia in aged male rats: a sequential histomorphometric study. J Bone Miner Res 2000;15:1085–1098.
- Blouin S, Libouban H, Moreau MF, Chappard D. Orchidectomy models of osteoporosis. Methods Mol Biol 2008;455:125–134.
- Bauss F, Lalla S, Endele R, Hothorn LA. The effects of treatment with ibandronate on bone mass, architecture, biomechanical properties and bone concentration of ibandronate in ovariectomized aged rats. J Reumathol 2002;29:2200–2208.
- Libouban H, Moreau MF, Legrand E, Audran M, Baslé MF, Chappard D. Comparison of histomorphometric descriptors of bone architecture with dual-energy X-ray absorptiometry for assessing bone loss in the orchidectomized rat. Osteoporos Int 2002;13:422–428.
- Epstein S, Zaidi M; Biological properties and mechanism of action of ibandronate: application to the treatment of osteoporosis. Bone 2005;37:433–440.
- Bauss F, Wagner M, Hothorn LH. Total administered dose of ibandronate determines its effect on bone mass and architecture in ovariectomized aged rats. J Reumathol 2002;29:990–998.
- Vanderschueren D, Van Herk D, Sinker AMH, Visser WJ, Shot LPC, Bouillon R. Bone and mineral metabolism in aged male rats: short and long term effects on androgen deficiency. Endocrinology 1992;130:2906–2916.
- Rissanen JP, Suominen MI, Peng Z, Morko J, Rasi S, Risteli J, Halleen JM. Short-term changes in serum PINP predict long-term changes in trabecular bone in the rat ovariectomy model. Calcif Tissue Int 2008;82:155–161.
- Miller PD, McClung MR, Macovei L, Stakkestad JA, Luckey M, Bonvoisin B, Reginster JY, Recker RR, Hughes C, Lewiecki EM, Monthly oral ibandronate therapy in postmenopausal osteoporosis: 1-year results from the MOBILE study. J Bone Miner Res 2005;20:1315–1322.
- Reginster JY, Adami S Lakatos P. Efficacy and tolerability of once-monthly oral ibandronate in postmenopausal osteoporosis: 2 year results from the MOBILE study. Ann Rheum Dis 2006;65:654–661.
- Monier-Faugere MC, Geng Z, Paschalis EP, Qi Q, Arnala I, Bauss F, Boskey AL, Malluche HH. Intermittent and continuous administration of the biphosphonate ibandronate in ovariohysterectomized beagle dogs: Effects on bone morphometry and mineral properties. J Bone Miner Res 1999;14:1768–1778.
- Lamy O, Sandini L, Pache I, Fatio S, Burnand J, Burckhardt P. Intravenous ibandronate in men with osteoporosis: an open pilot study over 2 years. J Endocrinol Invest 2003;26:728–732.