Abstract
The conventional lumbar separation was performed by removing soft tissue, subsidiary structures and leaving only the vertebral body. The vertebral body was cut into two halves along the median sagittal plane, keeping the upper and lower end plates of each half, which were subsequently used for biomechanical, morphological and density experiments. From the age of 20–29 to 30–39 years, both the horizontal trabecular thickness (Tb.Th) and vertical Tb.Th decreased; the horizontal and vertical Tb.Sp increased; the plate-like trabecular Tb.Th decreased; the apparent density and volume ratio decreased; and the elastic modulus and the ultimate stress decreased; with all changes being statistically significant (p < 0.01). Similar trends were obtained from ages 40–49 to 50–59, although the changes were not significant (p > 0.05), except for the reduction in ultimate stress (p < 0.05). With aging, the collagen cross-linking capacity declined; the thicknesses of the collagen fibrils were variable, ranging from almost the same to loose, sparse or disordered thickness; and the finer collagen fibrils between the thick filaments were disorganized. In males aged from 20 to 59 years old, the horizontal and vertical Tb.Th and the plate-like Tb.Th of the vertebral body decreased, while the horizontal and vertical Tb.Sp increased. Additionally, the density, elastic modulus and the ultimate stress of the cancellous bone decreased with age. Thus, the associated changes of bone microstructure, density and biomechanics with age may lead to an increased risk of osteoporosis and fracture.
Introduction
With aging, fracture risk gradually increases. Some studies have indicated that osteoporosis is closely related to the improvement of fracture risk [Citation1]. In the past, osteoporosis has been defined by an absolute reduction in bone mass and decreased bone density [Citation2,Citation3]. Now, however, researchers have been paying increasing attention to cancellous bone microstructural changes, which in turn cause changes in biomechanical and mineral density properties of cancellous bone. These changes have been suggested as the main reasons for osteoporosis [Citation4]. Recent studies [Citation5–7] have made important contributions to the study of bone microstructure. The investigators have analyzed in detail the morphological and density parameters of trabecular bone by reconstructing the three-dimensional structure of trabeculae using computed tomography (CT), micro-CT and fine element analysis. However, the results from previous studies are contradictory. On one hand, the experimental method in prior studies was based on the duality of cancellous bone through the CT threshold. When the threshold was set high, the number of trabecular bone was lost. Any soft tissue was considered as the bone structure while the threshold was set low. Because there are no uniform international CT threshold criteria, the CT thresholds were different for different types of CT and micro-CT or scanning process used. This is probably the main reason for the contradictory results in previous investigations. On the other hand, it would be due to the different subjects, such as men and women, or different races.
In the present study, we used the scanning electron microscope (SEM) to measure men’s lumbar micro-structure parameters in the age groups of 20–29 years, 30–39 years, 40–49 years and 50–59 years old. We also investigated the changes in biochemical and mineral density characteristics of lumbar cancellous bone with aging, and described the relationships between them, thus providing further information for the study of the relationship between age and risk of fracture and the treatment of osteoporosis.
Materials and methods
We analyzed 32 fresh L3 vertebral bodies donated by 32 men cadavers, aged 20–60 years old. We grouped the vertebrae into four age categories, group A: 20–29 years old; group B: 30–39 years old; group C: 40–49 years old; group D: 50–59 years old. Each group consisted of eight centrums. The conventional lumbar separation was performed by removing soft tissue and subsidiary structures, leaving only the vertebral body. The specimens were wrapped in normal saline gauze and stored in a freezer at −20°C. All the vertebrae were selected using CT scans, thereby avoiding vertebrae that exhibited visible pathological changes.
Morphological observation of trabeculae
Vertebral bodies from each age group were thawed at room temperature and cut into two halves, along the median sagittal plane. Each half was then cut into a 12 × 12 mm (length × width) specimen which retained the upper and lower end-plates. In this way, there were 16 specimens in each age group. The cancellous bone around each specimen was repeatedly washed using physiological saline; then it was dehydrated and sprayed with gold, and thereafter imaged with a scanning electron microscope from the upper end-plate to the lower end-plate. Morphological analysis and measurement of trabecular bone and collagen fibrils were performed using efilm2.12 (Merge Healthcare US). Vertical trabeculae are the ones perpendicular to the endplate, while horizontal trabeculae refer to the ones parallel to the endplate. The rod-shaped trabeculae are round, and have the rod-shaped structure, only constituting one wall of the marrow cavity. The plate-like trabeculae is the trabecular bone of the plat structure which is plate, having the irregular shape, constituting one to three wall of the bone marrow cavity (). The plate-like trabeculae can even independently form the bone marrow cavity. The rod-like trabecular thickness (Tb.Th) is the thickness of the midpoint of the trabecular bone. The plate-like trabecular thickness: firstly, we determined the cross-section of the trabecular bone which was set five equidistant points in region of interest (ROI). We measured the thickness of the points and calculated their average which was the plate-like trabecular thickness. The horizontal trabecular separation (Tb.Sp) is the shortest distance between the two vertical planes of the midpoint of the two adjacent vertical trabecular bones. The vertical trabecular separation (Tb.Sp) is the shortest distance between the two horizontal planes of the midpoint of the two adjacent horizontal trabecular bones. The collagen thickness: we took five equidistant fiber points of each collagen in ROI, measured the thickness of the points, and calculated the average which was the collagen thickness.
Figure 1. Left: plate-like trabeculae. The plate-like trabeculae is the trabecular bone of the plat structure which is plate, having the irregular shape. The plate-like trabeculae can even independently form the bone marrow cavity. Right: rod-like trabeculae. The rod-shaped trabeculae are round, and have the rod-shaped structure, only constituting one wall of the marrow cavity.
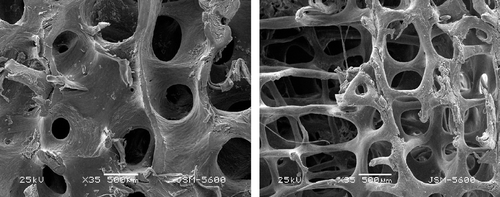
Biomechanics
Eight specimens were selected from each age group. The surfaces of both the upper and lower sides were smoothed to a parallel finish by filling with bone cement. Resistance-strain foils (Germany, lee gauge 1 × 1 mm, resistance 120 Ω, K 2.12) were then affixed on the front of the test pieces (1/3 of the length). After ensuring that the vertical resistance-strain foils on the test pieces were at the same horizontal level as the surface, the resistance-strain foil wires were connected to a resistance-strain gauge (Shanghai Huadong Electronics Instrument Factory, YJ-26-static resistance strain gauge) (). The strain-displacement was then measured when the test pieces were loaded with increasing physical loads from 0, 50 to 100 N on a universal material testing machine (Shimadzu AG-107A). The elastic modulus was calculated based on the observed data. The resistance-strain foils were then removed, and ultimate load experiments were performed on the same test samples.
Density
We used eight remaining specimens from each age group for density measurements. Specimens were milled in the frozen state at an average size of 7 × 7× 20 mm (length × width × height) as regular test specimens (volume = Va) using a CNC milling machine (Changchun CNC Machine Tool Co., LTD, Model XK7825). After repeatedly washing with water for 6 h, the test pieces were soaked in a heparin solution (20000 U/ml) for a total of 12 h, using fresh solution every 6 h. The test samples were then washed with normal saline for 6 h in order to completely remove blood, marrow and tissue debris in the medullary cavity. According to the method of Jun Ouyang et al. [Citation8], the test samples were degreased in anhydrous alcohol for 48 h (switching to fresh solution after the 24 h), air-dried for 24 h and weighed using an electronic analytical balance (weight = W, precision = 0.0001 g). According to the Archimedes principle, the volume of each test specimen trabecular was measured as Vb, apparent density = W/Va; and volume ratio = Vb/Va.
Statistics
The SPSS 10.0 statistical software package was used for statistical analysis. Horizontal rod-like Tb.Th, vertical rod-like Tb.Th, horizontal Tb.Sp, vertical Tb.Sp, Plate-like Tb.Th, apparent density, volume ratio, elastic modulus and ultimate stress were expressed as mean ± standard deviation (sd), Multiple sets of samples were compared using one-way ANOVA. p < 0.05 was considered as statistically significant.
Results
Within group A (20–29 years old), the vertical trabeculae were thicker than the horizontal trabeculae ().
From group A to group B (30–39 years old), the horizontal and vertical Tb.Th decreased significantly (p < 0.01). However, there was no significant change (p > 0.05) in Tb.Th from group B to group C (40–49 years old), and the vertical trabeculae were thicker than the horizontal trabeculae (p < 0.05). From group C to group D (50–59 years old), the horizontal and vertical trabecular thicknesses both decreased non-significantly (p > 0.05). Within the 50–59 years group, there was no significant difference in Tb.Th (p > 0.05 ).
Table I. Bone morphological thickness in various age groups (mm).
The horizontal and vertical Tb.Sp were nearly equivalent within 20–29 years group (p > 0.05). From age 20–29 years to 30–39 years, the horizontal and vertical Tb.Sp were significantly increased (p < 0.01). There was no significant change (p > 0.05) in Tb.Sp from age 30–39 years to 40–49 years, and the horizontal Tb.Sp was higher than the vertical Tb.Sp (p > 0.05). From age 40–49 years to 50–59 years, the horizontal and vertical Tb.Sp increased non-significantly (p > 0.05), and the horizontal Tb.Sp was non-significantly higher than the vertical Tb.Sp (p > 0.05, ).
The plate-like trabecular bone was mainly distributed in the upper and lower end-plate regions. With aging, the amount of plate-like trabecular bone gradually decreased. From A to B group, the plate-like Tb.Th significantly decreased (p < 0.01). However, there was no change in plate-like Tb.Th from 30–39 years to 40–49 years old (p > 0.05) as well as from 40–49 years to 50–59 years old (p > 0.05, ).
The apparent density and volume ratio decreased significantly from 20–29 years to 30–39 years old (p < 0.01). There was no obvious change in the apparent density or volume ratio from 30–39 years to 40–49 years old (p > 0.05), whereas these parameters slightly decreased, but non-significantly (p > 0.05, ), from 40–49 years to 50–59 years old. We did not measure tracular number density because the changes in marrow cavity and trabecular bone morphology can fully explain the morphological changes in bone micro-structure, and these indirectly reflect the changes in trabecular number density.
Table II. Density of the L3 in various age groups.
After SEM images were magnified 10,000 times, we could clearly observe the morphology and the arrangement of the trabecular collagen fibers (). The direction of collagen fibers is consistent with the long axis of the trabecular bone. In the 20–40 years age range, the thickness of the collagen fibers was uniform, and arranged in neat rows. The average thickness of the collagen fibers within the ROI can be used to represent the thickness of the collagen fibers. With the increasing age, the arrangement of the collagen fibers was gradually became disordered, and the thickness was uneven. Changes in the collagen fiber morphology were correlated with age. Within the 20–29 years age group, the bone collagen cross-linked into uniform collagen fibers, and the width of each collagen fiber was approximately 2–3 μm. The fibers were closely arranged, and the orientation was consistent. The fibrils were connected obliquely by the thinner collagen fibers for reinforcement. With increasing age, the cross-linking ability of the collagen declined, and the widths of the collagen fibrils were not consistent. However, the orientations of the collagen fibrils were essentially uniform, although the arrangement of the fibrils was loose, sparse and disordered, and the orientation of the oblique collagen fibers between them was disorganized. This change was particularly evident from age group 40–49 years to 50–59 years old ().
Figure 4. Observation of collagen fibers within the bone trabeculae by SEM (×10,000). A: Collagen fibers of a bone trabecula in a man of 26 years. The fibers were closely arranged, and the orientation was consistent (A: the long arrow). The fibrils were connected obliquely by the thinner collagen fibers (A: the short arrow) for reinforcement. B: Collagen fibers of 35 years. C: Collagen fibers of 48 years. With increasing age, the widths of the collagen fibrils were not consistent (C: the short arrow). However, the orientations of the collagen fibrils were essentially uniform, D: Collagen fibers of 55 years. The arrangement of the fibrils was loose, sparse and disordered (D: the long arrow). The orientations of the oblique collagen fibers between them were unorganized (D: the short arrow).
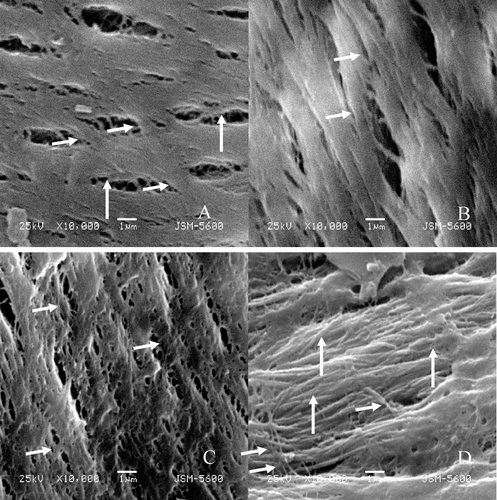
From 20–29 years to 30–39 years old, the elastic modulus and the ultimate stress decreased significantly (p < 0.01) (). There was no obvious change in the elastic modulus or the ultimate stress from 30–39 years to 40–49 years old (p > 0.05). From 40–49 years to 50–59 years old, the ultimate stress decreased significantly (p < 0.05), but there was a non-significant decrease (p > 0.05, ) in the elastic modulus.
Table III. Biomechanical data of the L3 in various age groups (MPa).
Discussion
In the present study, we measured the morphological, density and biomechanical parameters of the lumbar micro-structure of 32 fresh cadavers (20–60 years), and found that the various parameters of the bone micro-structure changed significantly every 10-year, and thus we took a 10-year interval as a year group. The endplate plays a very important position in vertebral mechanical transmission. The force is passed to the endplate through the disc, and then passed to the trabeculae by the end plate. From its anatomy, the endplate is not level, and thus the lumbar trabeculae are not forced in a single vertical direction. By using bone cement to fill the irregular areas of the endplate, we preserved the endplate and the action also better reflected the authenticity of the force the trabeculae suffered in the coming mechanical experiments.
The physical and anatomical changes of bone stem from the response to applied external loads. Cvijanovic et al. [Citation9] and Keller et al. [Citation10] suggested that, because the vertebrae mainly receive an axial load, the vertical trabeculae are thicker than the horizontal trabeculae; with increasing age, the horizontal Tb.Th decreases gradually, while the vertical trabeculae thicken in compensation. Mosekide et al. [Citation11] reported that the horizontal Tb.Th decreased gradually from 20 to 80 years old, whereas the vertical Tb.Th had no obvious change and no correlation with age. Additionally, they reported that the horizontal and vertical Tb.Sp are significantly increased in correlation with age. Some study has reported that the vertical trabeculae receive more stress because of the decrease in horizontal Tb.Th, thus, the vertical trabeculae thicken gradually with increasing age [Citation12]. The present study showed that, within the 20–29 years group, the vertical trabeculae were significantly thicker than horizontal trabeculae. This finding is consistent with the above mentioned reports.
However, with increasing age, the differences between the horizontal and vertical Tb.Th decrease gradually until they are not statistically different in the 50–59 years group (p > 0.05). This result might be due to the degeneration of intervertebral disc. One of the main functions of the intervertebral disc is to transfer the weight of the trunk and other loads to the vertebral body. Within the 20–29 years group, discs have been reported to transfer 60% stress to trabeculae that were in the vertebral body [Citation13,Citation14]. Although no significant change in the intervertebral disc thickness was observed with advancing age in our study, it is likely that the internal structure of the intervertebral discs changed. This phenomenon was suggested by a loss of water, by a denatured fibrous ring and by a gradual increase in the fissures of the intervertebral discs [Citation15]. These results suggest that the intervertebral discs received more stress within the cortical bone of the vertebral body [Citation16]. With advancing age, an increase of cortical bone has been observed, verifying the aforementioned findings [Citation17]. Therefore, regardless of whether horizontal or vertical, the trabeculae in the vertebral body gradually received less stress, resulting in a gradual decrease in Tb.Th, eventually approximating the vertical Tb.Th.
Moreover, the plate-like Tb.Th decreases gradually with increase in age. It is likely that the activity of osteoclasts also weaken with age, leading to reduced bone deposition. In addition, a decrease of stress and microfractures may contribute to the thinner plate-like trabeculae.
On the contrary, the present study indicates that the heights of the horizontal and vertical Tb.Sp increased directly with age. Within the 20–29 years age group, the horizontal and vertical Tb.Sp were essentially equivalent, whereas after 30 years old, the horizontal Tb.Sp was higher than the vertical. These results are consistent with those reported in literature [Citation12]. However, some studies have reported that, with an increase in age, the height of the vertebral body decreased, which is inconsistent with our observed increase in the vertical Tb.Sp. The reduction of trabeculae number as well as the thinner plate-like and rod-like Tb.Th with age may also lead to a vertical and horizontal Tb.Sp increase. The overall height of the vertebral body may decrease because of changes in trabeculae microstructure. Some studies have suggested that a reduction in trabeculae number mainly refers to the horizontal trabeculae, while the numbers of vertical trabeculae remain unchanged. The significant increase of horizontal Tb.Sp over time also indirectly confirms that the number of vertical trabeculae decreases with increasing age.
The present study indicated the differences between vertical and horizontal trabeculae, and between rod-like and plate-like trabeculae. However, these differences were not so easily distinguished. Many researchers, such as Fields et al. [Citation18]. tried to classify the vertebral trabeculae into vertical, horizontal and oblique one. Exactly, on the basis of the three-dimensional reconstruction and finite element analysis, the quantitative study of the bone micro-structure is not precise enough, resulting in some results with many contradictions. Therefore, in the present study, we sprayed specimen with gold, directly imaged the trabecular by SEM, and measured the trabecular with efilm2.12 software. Although it can not reflect the three-dimensional structure of the trabecular bone, it may objectively quantitatively analyze the two-dimensional space structure. The parameters of the bone marrow cavity and the trabecular bone reflected the changes of the bone micro-structure related to age, and the bone marrow cavity is constituted by the transverse, longitudinal and plate-like bone trabeculae. So the morphological parameters we measured only included the horizontal, vertical and plate-like Tb.Th, as well as the horizontal and vertical Tb.Sp.
Since birth, bone formation is influenced by external applied stress, which orchestrates a continual bone remodeling process. When bone formation is greater than bone absorption, the process is known as osteogenesis. With the advancement of age, bone absorption gradually becomes dominant [Citation19], resulting in a substantial loss of bone mass or reduced bone density [Citation4,Citation20]. Our study results indicate that the apparent density of bone peaked in the 20–29 years age group, whereas from 30 to 39 years of age the bone density dropped significantly. While some studies have reported that the peak of the human bone surface density appears between 30 and 39 years of age [Citation21], this is inconsistent with findings in our study. The observed volume ratio increase also confirms that the bone marrow cavity enlarged with age.
The main organic component of bone is type I collagen, a type of microfiber protein that has three polypeptide chains [Citation22]. In the body, type I collagen induces microfibrosis through enzyme and non-enzyme-mediated cross-linking reactions, forming aggregated collagen fibers [Citation23,Citation24]. After type I collagen fibrosis induces microfibrosis, cells are stimulated to differentiate and to contribute to monomer collagen fibril deposition. This microfibrous deposition not only adsorbs various cytokines, but can also induce osteoblasts to proliferate and differentiate, thus accelerating bone formation [Citation25]. The cross-linking of bone collagen can also increase bone toughness and elastic modulus [Citation26,Citation27]. In this study, we observed that, with advancing age, the ability to cross-link decreased; the thickness of the collagen fibrosis was not same, and the collocation of the collagen fibrils was loose, sparse and disordered. This change was particularly evident within the 40–49 years and 50–59 years age groups. It is likely that, in the bone microenvironment, the cross-linking between enzyme-mediated and non-enzyme-mediated reactions changed with increase in age. The reduction of bone mineralization also likely reduced the capacity of collagen cross-linking, while the changes in collagen cross-linking ability accelerated the decline in bone mineral deposition [Citation28]. With age, the shortened peptide chains of collagen microfiber tail peptides may also reduce the ability of collagen microfibrosis.
Bone elastic modulus is a very important mechanical parameter [Citation29,Citation30]. When doing the experiment of the elastic modulus and ultimate load test of trabecular bone, in the preload of 0N, 50N, 100N, we measured separately the elastic modulus of the specimen and calculated the mean value. After completing the test of modulus elasticity, we removed strain gages. The excessive preload would cause micro-damage of the trabeculae. However, in our preliminary experiments, the 100N force did not damage the bone micro-structure. As a viscoelastic material, bone also exhibits relaxation and creep. In our previous study, we investigated relaxation and creep, and the results were consistent with the views of most researchers.
The present study revealed an obvious change in the elastic modulus or the ultimate stress from 20–29 years to 30–39 years old (p < 0.01). The decrease in elastic modulus of bone with age could be attributed to the reductions in bone mineral density, bone inorganic constituents, and changes in bone microstructure morphology. Uchiyama et al. [Citation31] reported that, when the deposition of bone was reduced, the elastic modulus correspondingly decreased. This finding is consistent with our experimental results, demonstrating that the limit load of the trabeculae decreases with an increase in age. This is in agreement with other previous studies [Citation20]. For all four age groups, there was a significant decrease in ultimate stress (p < 0.05). The first significant decrease might be due to the decrease in trabeculae widths and bone density. The decrease in the limit load from age 40–49 years to 50–59 years might be due to the chaotic arrangement of collagen fibers and due to the lower bone toughness [Citation32].
In summary, the widths of the horizontal and vertical rod-like Tb.Th and the plate-like Tb.Th gradually decreased in the vertebral body, and the heights of the horizontal and vertical Tb.Sp gradually increased from age 20–29 years to 50–59 years old. While spongy bone density, limit load and elastic modulus decreased with age. Therefore, age is associated with biochemical, density and microstructural bone changes. The present study also contributes a theoretical basis for the study of osteoporosis and the increased risk of bone fracture with age.
The present study also has some limitations. It lacks the information on the medical history of the subjects, such as the cause of death, the PTH, vitamin D status, as well as physical activity of the subjects, so that can not analyze the bone metabolism with age. The number of sample size is a little small and cannot include all different aspects and variables of age changes. We would collect the medical history and the body physical data (height, weight, BMI) of the subjects, enlarge the sample size, and start by dividing all vertebrae to maximum two age groups to reduce the amount of variability in the next work.
Declaration of Interest: The authors declare no conflict of interest.
References
- Nachemson AL, Schultz AB, Berkson MH. Mechanical properties of human lumbar spine motion segments. Influence of age, sex, disc level, and degeneration. Spine 1979;4:1–8.
- Beck JS, Nordin BE. Histological assessment of osteoporosis by iliac crest biopsy. J Pathol Bacteriol 1960;80:391–397.
- Caldwell RA. Observations on the incidence, aetiology, and pathology of senile osteoporosis. J Clin Pathol 1962;15:421–431.
- Stauber M, Müller R. Age-related changes in trabecular bone microstructures: global and local morphometry. Osteoporos Int 2006;17:616–626.
- Chen H, Washimi Y, Kubo KY, Onozuka M. Gender-related changes in three-dimensional microstructure of trabecular bone at the human proximal tibia with aging. Histol Histopathol 2011;26:563–570.
- Nishiyama KK, Boyd SK. In vivo assessment of trabecular and cortical bone microstructure. Clin Calcium 2011;21:1011–1019.
- Tjong W, Kazakia GJ, Burghardt AJ, Majumdar S. The effect of voxel size on high-resolution peripheral computed tomography measurements of trabecular and cortical bone microstructure. Med Phys 2012;39:1893–1903.
- Jun O, Yang G, Wu W. Biomechanical characters of human lumbar vertebral trabecular bone. Chin J Biomed Eng 1997;16:289–293.
- Cvijanovic O, Bobinac D, Zoricic S, Ostojic Z, Maric I, Crncevic-Orlic Z, Kristofic I, Ostojic L. Age- and region-dependent changes in human lumbar vertebral bone: a histomorphometric study. Spine 2004;29:2370–2375.
- Keller TS, Hansson TH, Abram AC, Spengler DM, Panjabi MM. Regional variations in the compressive properties of lumbar vertebral trabeculae. Effects of disc degeneration. Spine 1989;14:1012–1019.
- Mosekide L. Age-related changes in vertebral trabecular bone architecture--assessed by a new method. Bone 1988;9:247–250.
- Thomsen JS, Ebbesen EN, Mosekilde LI. Age-related differences between thinning of horizontal and vertical trabeculae in human lumbar bone as assessed by a new computerized method. Bone 2002;31:136–142.
- Rockoff SD, Sweet E, Bleustein J. The relative contribution of trabecular and cortical bone to the strength of human lumbar vertebrae. Calcif Tissue Res 1969;3:163–175.
- Yoganandan N, Myklebust JB, Cusick JF, Wilson CR, Sances A. Functional biomechanics of the thoracolumbar vertebral cortex. Clin Biomech 1988;3:11–18.
- Vernon-Roberts B, Moore RJ, Fraser RD. The natural history of age-related disc degeneration: the pathology and sequelae of tears. Spine 2007;32:2797–2804.
- Homminga J, Weinans H, Gowin W, Felsenberg D, Huiskes R. Osteoporosis changes the amount of vertebral trabecular bone at risk of fracture but not the vertebral load distribution. Spine 2001;26:1555–1561.
- Keaveny TM, Yeh OC. Architecture and trabecular bone - toward an improved understanding of the biomechanical effects of age, sex and osteoporosis. J Musculoskelet Neuronal Interact 2002;2:205–208.
- Fields AJ, Lee GL, Liu XS, Jekir MG, Guo XE, Keaveny TM. Influence of vertical trabeculae on the compressive strength of the human vertebra. J Bone Miner Res 2011;26:263–269.
- Marie PJ, de Vernejoul MC, Connes D, Hott M. Decreased DNA synthesis by cultured osteoblastic cells in eugonadal osteoporotic men with defective bone formation. J Clin Invest 1991;88:1167–1172.
- Ebbesen EN, Thomsen JS, Beck-Nielsen H, Nepper-Rasmussen HJ, Mosekilde L. Age- and gender-related differences in vertebral bone mass, density, and strength. J Bone Miner Res 1999;14:1394–1403.
- Clarke BL, Ebeling PR, Jones JD, Wahner HW, O’Fallon WM, Riggs BL, Fitzpatrick LA. Changes in quantitative bone histomorphometry in aging healthy men. J Clin Endocrinol Metab 1996;81:2264–2270.
- Williams BR, Gelman RA, Poppke DC, Piez KA. Collagen fibril formation. Optimal in vitro conditions and preliminary kinetic results. J Biol Chem 1978;253:6578–6585.
- Knott L, Bailey AJ. Collagen cross-links in mineralizing tissues: a review of their chemistry, function, and clinical relevance. Bone 1998;22:181–187.
- Robins SP, Brady JD. Collagen cross-linking and metabolism. In: Bilezikian JP, Raisz LG, Rodan GA, eds. Principles of Bone Biology. Vol. 1. San Diego, CA, USA: Academic Press; 2002. pp 211–223.
- Vashishth D, Gibson GJ, Khoury JI, Schaffler MB, Kimura J, Fyhrie DP. Influence of nonenzymatic glycation on biomechanical properties of cortical bone. Bone 2001;28:195–201.
- Wang X, Shen X, Li X, Agrawal CM. Age-related changes in the collagen network and toughness of bone. Bone 2002;31:1–7.
- Paschalis EP, Shane E, Lyritis G, Skarantavos G, Mendelsohn R, Boskey AL. Bone fragility and collagen cross-links. J Bone Miner Res 2004;19:2000–2004.
- Hernandeza CJ, Tanga SY, Baumbach BM. Trabecular bone modeling and subcapital femoral fracture. J Musculoskelet Neuronal Interact 2007;7:69–73.
- Wolfram U, Wilke HJ, Zysset PK. Rehydration of vertebral trabecular bone: influences on its anisotropy, its stiffness and the indentation work with a view to age, gender and vertebral level. Bone 2010;46:348–354.
- Yeni YN, Zinno MJ, Yerramshetty JS, Zauel R, Fyhrie DP. Variability of trabecular microstructure is age-, gender-, race- and anatomic site-dependent and affects stiffness and stress distribution properties of human vertebral cancellous bone. Bone 2011;49:886–894.
- Uchiyama T, Tanizawa T, Muramatsu H, Endo N, Takahashi HE, Hara T. Three-dimensional microstructural analysis of human trabecular bone in relation to its mechanical properties. Bone 1999;25:487–491.
- Chen XY, Zhang XZ, Guo Y, Li RX, Lin JJ, Wei Y. The establishment of a mechanobiology model of bone and functional adaptation in response to mechanical loading. Clin Biomech (Bristol, Avon) 2008;23 Suppl 1:S88–S95.